Abstract
Objectives
We performed in vitro validation of a non-invasive skin-mounted system that could allow quantification of anteroposterior (AP) laxity in the outpatient setting.
Methods
A total of 12 cadaveric lower limbs were tested with a commercial image-free navigation system using trackers secured by bone screws. We then tested a non-invasive fabric-strap system. The lower limb was secured at 10° intervals from 0° to 60° of knee flexion and 100 N of force was applied perpendicular to the tibia. Acceptable coefficient of repeatability (CR) and limits of agreement (LOA) of 3 mm were set based on diagnostic criteria for anterior cruciate ligament (ACL) insufficiency.
Results
Reliability and precision within the individual invasive and non-invasive systems was acceptable throughout the range of flexion tested (intra-class correlation coefficient 0.88, CR 1.6 mm). Agreement between the two systems was acceptable measuring AP laxity between full extension and 40° knee flexion (LOA 2.9 mm). Beyond 40° of flexion, agreement between the systems was unacceptable (LOA > 3 mm).
Conclusions
These results indicate that from full knee extension to 40° flexion, non-invasive navigation-based quantification of AP tibial translation is as accurate as the standard validated commercial system, particularly in the clinically and functionally important range of 20° to 30° knee flexion. This could be useful in diagnosis and post-operative evaluation of ACL pathology.
Cite this article: Bone Joint Res 2013;2:233–7.
Article focus
Non-invasive adaptation of computer navigation technology is as reliable, precise and accurate as a commercially available, image-free, invasive navigation system
Key messages
From extension to 40° of knee flexion the non-invasive method is as reliable, precise and accurate as the commercial invasive system
Beyond 40° knee flexion, reliability and accuracy are unacceptable
Strengths and limitations
This is the first validation of a non-invasive adaptation of navigation-based technology that uses similar frames of reference to those used intra-operatively in measuring anteroposterior tibial translation
These data provide a foundation and rationale for further invivo analysis
A limitation of this study is the use of cadaveric material, which was mandatory given the nature of the invasive comparison. Further in vivo validation must now be performed before the device is used in in vivo research or clinical practice
Introduction
Evaluation of anterior cruciate ligament integrity in the clinical setting relies predominantly on establishing anteroposterior (AP) laxity by manual testing. The Lachman test has been shown to be highly sensitive in the diagnosis of cruciate deficiency,1-5 but in terms of evaluating cruciate ligament reconstruction, the test remains examiner-dependent and subjective. The reliability of non-invasive methods that objectively evaluate AP translation is reported as inconsistent in the literature.5-15
Image-free navigation has been thoroughly validated and is used by many surgeons to provide intra-operative assessment of AP tibial laxity.16-19 The role of this technology is limited to the operative setting due to the requirement for invasive optical tracker placement. A non-invasive adaptation of this technology using software algorithms identical to those used in a commercially available image-free navigation system has been validated to quantify lower limb mechanical and coronal knee laxity in early flexion.20,21 Using the same fabric strap method, a pilot study on six embalmed cadaveric lower limbs gave acceptable reliability, precision and agreement with a conventional image-free navigation system measuring AP translation in early flexion.22
The primary aim of this study was to compare a non-invasive system with a validated and commonly used intra-operative computer navigation system in terms of reliability and repeatability of AP translation measurement and agreement with the invasive system. The secondary aim was to observe the effect of knee flexion on measurement reliability, precision and agreement between the two systems.
Materials and Methods
A single investigator (DFR) carried out all testing. A total of 12 lower limbs were used from eight cadavers (five female and three male, mean age 80.5 years (65 to 91)). The image-free OrthoPilot navigation system was used with passive optical trackers (B. Braun Aesculap, Tuttlingen, Germany). The optical camera was positioned 2 m from the specimen. Experimental software allowed registration of the centres of the hip, knee and ankle following a series of prescribed lower limb movements and localisation of key bony landmarks. The registration algorithms in this software are identical to validated, commercially available software used in computer-assisted surgery.
Two separate methods of tracker fixation were used: standard bone screws with tracker mounts, and a fabric strap used to secure a baseplate. The fabric strap and baseplate used in this study had been validated.20 In order to allow attachment of a transducer to the anterior tibia to allow application of a moment perpendicular to the coronal plane of the tibia, a screw with eyelet was inserted into the tibial tuberosity. A 3 cm incision was made over the proximal anterior femur down to bone and all soft tissues were cleared from the anterior femoral cortex. Both cortices were drilled and a screw with an eyelet inserted perpendicular to the long axis of the femur. This screw was used to suspend the thigh above the laboratory table, thus minimising soft-tissue artefacts from the work-surface. In order to create a foot support, a loop of cord from a second laboratory stand was secured proximal to the metatarsal heads; this maintained the angle of flexion of the knee. In order to limit knee extension during testing, four separate bungee cords were attached to two screws inserted into the medial and lateral distal tibial cortices. These cords were secured to the laboratory table. Various lengths were available and changed to adapt to various positions of knee flexion, keeping the bungee cords as tight as possible according to position of the foot throughout range of knee flexion. The pull of the bungee cord was counteracted by the foot support, resulting in no flexion or extension of the knee joint during AP tibial stress testing.
The limbs were put through 24 full cycles of flexion and extension and ten applications of 100 N anterior force applied via the tibial tuberosity screw before testing in order to minimise systematic error due to progression of tissue elasticity. The experiments were carried out over 12 days, during which the temperature of the laboratory was controlled and constant. The specimens were not refrigerated between experiments.
AP tibial translation was recorded by the system following force application at intervals of 10° from extension to 60° of knee flexion. This procedure was performed twice using optical trackers mounted invasively using bone screws, and twice using the non-invasive fabric strap method. A force transducer was secured to the tibial tuberosity eyelet screw and a linear force applied in an anterior direction perpendicular to the long axis of the tibia until a force of 100 N had been reached. The value of 100 N was selected considering various methodologies used in previous in vitro and in vivo testing of AP laxity of the knee joint.6,7,10,23,24 The software automatically recorded maximum displacement in millimetres. The investigator did not watch the computer monitor during testing – however, true blinding to results throughout the entire experiment was not possible due to single investigator setup and potential contamination issues while handling fresh cadaveric material. Only the foot pedal could be accessed during testing and the screen could not be repeatedly obscured between tests.
Statistical analysis
Statistical testing was applied to measurements taken from each interval of 10° flexion separately to allow analysis of the effect of knee flexion angle on reliability, repeatability and agreement. Reliability within each method of tracker fixation used in measuring AP tibial translation was analysed by calculating the intraclass correlation coefficients (ICCs).25 A coefficient of ≥ 0.75 demonstrates very good reliability.26,27 The calculation of ICC was performed using IBM SPSS v17.0 software (IBM Corp., Armonk, New York). Coefficient of repeatability (CR) was calculated to demonstrate repeatability between test–retest measurements within each method of tracker fixation.28 The CR defines the interval within which 95% of test–retest differences lie (i.e. within 2 sds of the test–retest differences.28 A limit of 3 mm was chosen for the repeatability of measurements when considering each system separately, and as a limit for agreement margin between the systems. This value was chosen based on diagnostic criteria for dichotomous testing between ‘normal’ and ‘injured’ knees when testing for ACL insufficiency using other measurement devices.29 A CR ≤ 3 mm denotes that 95% of all measurements are within a range of ±1.5 mm. Bland–Altman plots were generated as a visual representation of the limits of agreement. 95% limits of agreement (LOA) were determined using the corrected standard deviation of the differences (sdc)28 to allow for repeated measurements. Mean difference between the system measurements ±1.96 sdc reflects the limits of agreement between the two systems. Acceptable limits of agreement were once again set at 3 mm. CR and LOA calculations were performed using Microsoft Excel (Microsoft Corp., Redmond, Washington).
Results
The mean fixed flexion for the 12 specimens was 6.8° (0° to 15°).
Figure 1 demonstrates the mean CR at each flexion interval throughout the range of flexion tested in this experiment (12.8° to 60°). Bland–Altman plots demonstrated no systematic error plotting screw fixation versus fabric strap fixation (Fig. 2). Figure 3 displays LOA at each 10° interval of knee flexion between measurements taken using invasive versus non-invasive tracker mounting.
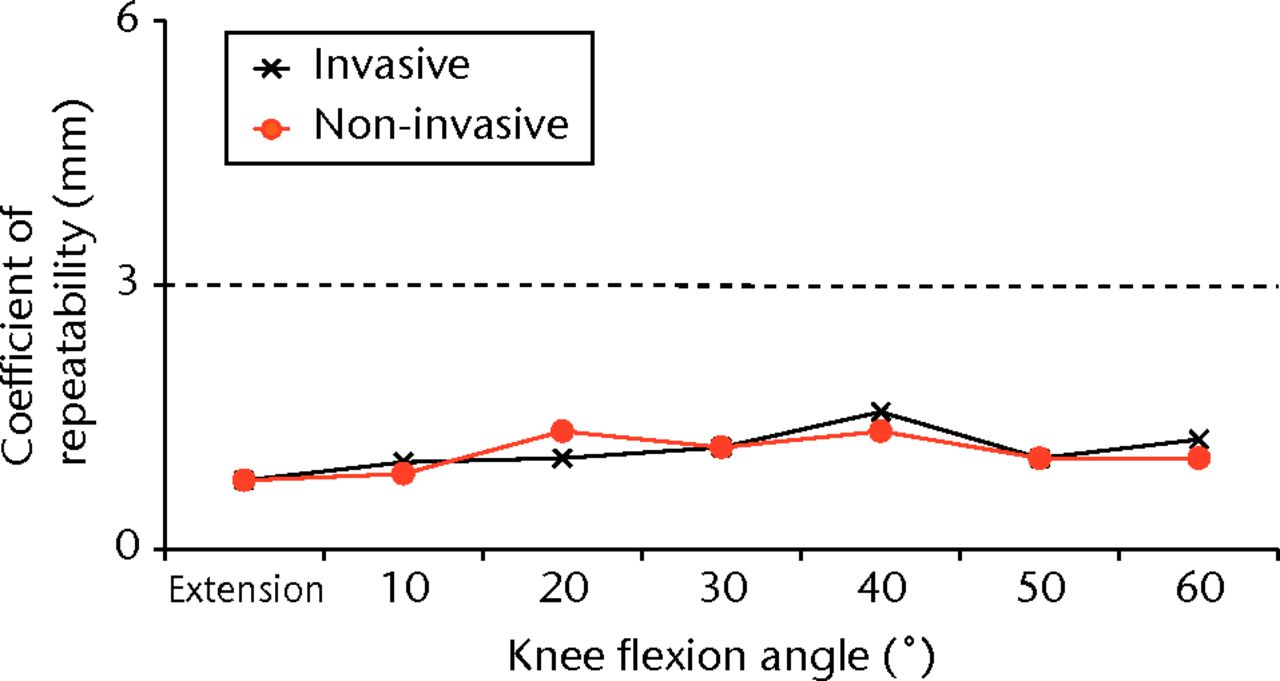
Fig. 1
Graph of the coefficient of repeatability (CR) for the invasive and non-invasive methods of measuring anteroposterior tibial translation, showing that both are acceptable (within the limit of 3 mm) throughout knee flexion.
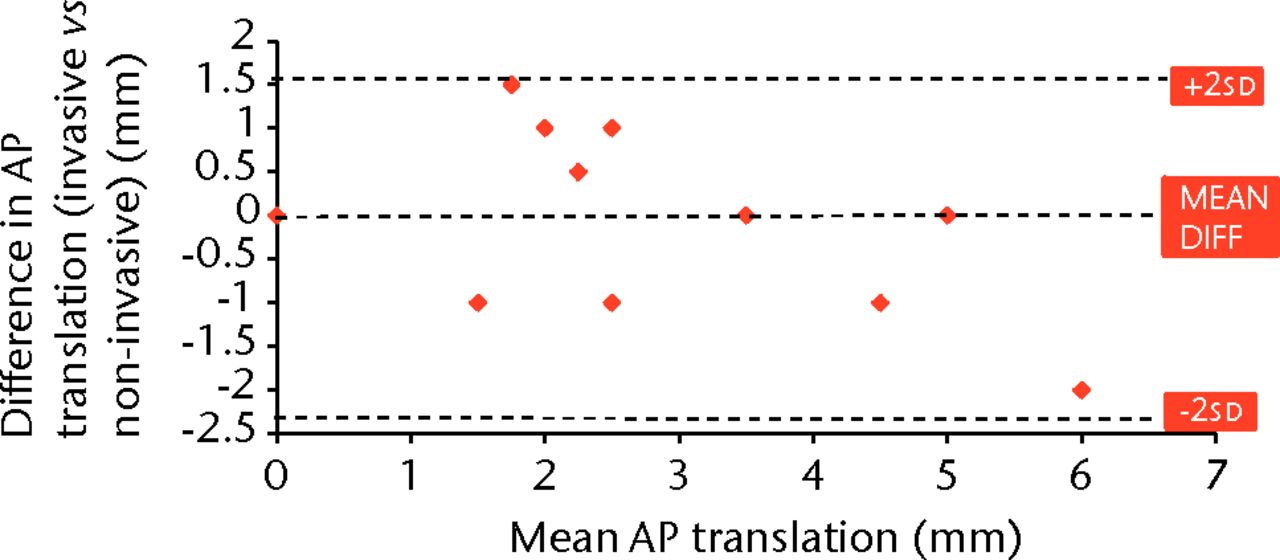
Fig. 2
Bland–Altman plot of the mean difference between anteroposterior (AP) measurements with trackers secured using bone screws (invasive) and fabric strapping (non-invasive) against the mean measurements of AP translation taken with the knee in full extension.
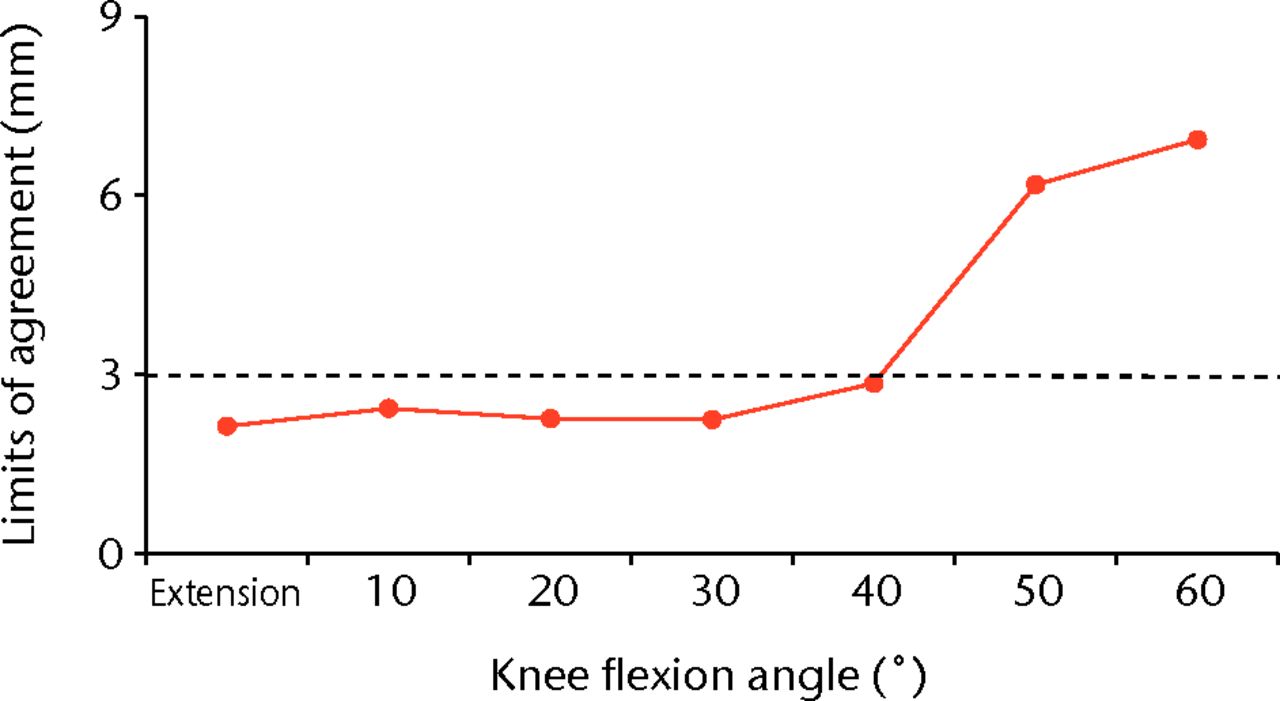
Fig. 3
Graph showing the limits of agreement (LOA) calculated from measurements taken using both methods of tracker fixation at each interval of 10° flexion. The limit for acceptable LOA is marked by the horizontal line at 3 mm, which is exceeded at flexion > 40°.
It was noted during the experiment that despite obvious subjective AP movement of the tibia, the system measured ‘0 mm’ AP displacement. This occurred at higher angles of flexion only, during one test at 50° flexion and during six tests at 60° flexion using the invasive and non-invasive methods.
Discussion
From extension to 40° knee flexion, both devices displayed similarly good reliability and precision. In this range, agreement between the devices is also acceptable. The angle of knee flexion does not affect precision, but it does affect accuracy of the non-invasive method in flexion > 40°. The validity of this non-invasive device has been demonstrated in the in vitro setting as it has demonstrated acceptable reliability, precision and accuracy between extension and 40°. Additional in vivo work should be carried out to further validate the device, as this range of knee flexion permits important tests of knee laxity such as the Lachman test,1 and is a useful range for demonstrating dynamic weight-bearing stability in early flexion such as squatting, ascending or descending a step when patients with ACL insufficiency often report feelings of ‘giving way’.30,31
Limitations of this experiment include use of cadaveric tissue, which lacks muscle tone and has different tissue properties to the in vivo setting. The invasive nature of the validation methodology used in this experiment mandated the use of cadaveric limbs. However, it is now important to study the effect on the non-invasive system of artefacts from live soft tissue in a range of live subjects. The experiment set-up involving limb suspension and securing knee flexion angle will alter kinematics of the lower limb to some degree. However, this set-up provided consistent testing conditions in terms of joint positioning, removing variables that may have obscured testing of precision and accuracy. These limitations are characteristic to in vitro validation of clinical devices32 and such experimental work is very important before progressing to reliability testing in vivo, in which the comparison of kinematic measurement is either not attempted or involves consequential intervention such as invasive placement of markers or ionising radiation. Based on the results of this study, in vivo validation should now be carried out. A limitation common to all image-free navigation systems is a decrease in accuracy beyond 50° of knee flexion, which is well documented in the literature.33-35 This is due to the femoral frame being defined by the transepicondylar axis (TEA), the landmarks for which are acquired at registration. The TEA collected at registration is not accurate enough to compensate for the marked displacement of the femoral and tibial axes during high knee flexion.33-35 This phenomenon was observed at higher flexion angles in this study. The limitation beyond 50° of knee flexion is important to note when using image-free navigation in any setting.
From extension to 40° flexion, the results of reliability, precision and accuracy are favourable for the non-invasive method of tracker fixation compared with arthrometric devices. Generally these devices provide a reliability (ICC) of 0.6.11,12 Concerns have been raised over accuracy of the most popular clinical devices, such as the KT-1000 (MEDmetric Corporation, San Diego, California).7,8,10,36
AP rather than rotatory laxity is the most reliable kinematic indicator of cruciate ligament integrity. Increases in anterior translation of between 2 mm and 14.4 mm following sectioning of the ACL have been reported using various methods of force application and measurement.37-40 Isberg et al37 compared normal knees with those with ACL rupture in 22 patients using radiostereometric analysis, and found a mean difference in anterior translation of 7.4 mm (2.2 to 17.4). Sectioning of the ACL has been shown in biomechanical studies to increase internal rotation by only 2° to 4° with the knee in early flexion (i.e., 20° to 30°).41-46 The posterior cruciate ligament is even less involved in rotatory stability, only demonstrating significant effect at 90° of knee flexion.47,48 Furthermore, reconstruction of the ACL may not restore rotational kinematics.49 Non-invasive devices assessing tibial rotation with an aim of detecting cruciate ligament pathology or dysfunction would have to be very sensitive compared with those detecting AP instability. The reliability of current devices used to quantify rotational laxity is relatively low and such devices are not routinely used in clinical practice, with the vast majority still in pre-clinical development.50 The pivot shift phenomenon has been mapped and characterised using invasive navigation-based technology, allowing comparison of ACL reconstruction techniques.51-55 However, a non-invasive adaptation of this has not yet tested in the clinical setting. Until more sensitive means of analysing tibial rotation are available, it may be more useful to detect AP instability for diagnosis of cruciate pathology and evaluation of surgical reconstruction.
Should the non-invasive method of tracker fixation method prove valid in vivo, it would provide a useful adjunct to clinical examination aiding diagnosis of cruciate pathology. A method of quantifying the forces applied during examination would increase knowledge of ‘normal’ laxity, allow standardisation of examination technique and permit comparison of surgical results between practitioners.
Conclusion
In the in vitro setting, the non-invasive method of tracker fixation proved as reliable and precise as the invasive method in measuring AP tibial translation, and demonstrated acceptable agreement within a diagnostically applicable range from full knee extension to 40° knee flexion.
The authors would like to thank the technical and administrative staff at the Laboratory of Human Anatomy, University of Glasgow. They would also like to thank Mr P. Cleary and Mr I. Freer for the excellent support provided in supplying equipment to facilitate this study.
1 Torg JS , ConradW, KalenV. Clinical diagnosis of anterior cruciate ligament instability in the athlete. Am J Sports Med1976;4:84–93.CrossrefPubMed Google Scholar
2 Donaldson WF 3rd , WarrenRF, WickiewiczT. A comparison of acute anterior cruciate ligament examinations: initial versus examination under anesthesia. Am J Sports Med1985;13:5–10. Google Scholar
3 Mitsou A , VallianatosP. Clinical diagnosis of ruptures of the anterior cruciate ligament: a comparison between the Lachman test and the anterior drawer sign. Injury1988;19:427–428.CrossrefPubMed Google Scholar
4 Zarins B , RoweCR. Combined anterior cruciate-ligament reconstruction using semitendinosus tendon and iliotibial tract. J Bone Joint Surg [Am]1986;68-A:160–177.PubMed Google Scholar
5 Liu SH , OstiL, HenryM, BocchiL. The diagnosis of acute complete tears of the anterior cruciate ligament: comparison of MRI, arthrometry and clinical examination. J Bone Joint Surg [Br]1995;77-B:586–588. Google Scholar
6 Lerat JL , MoyenB, JennyJY, PerrierJP. A comparison of pre-operative evaluation of anterior knee laxity by dynamic X-rays and by the arthrometer KT 1000. Knee Surg Sports Traumatol Arthrosc1993;1:54–59.CrossrefPubMed Google Scholar
7 Daniel DM , StoneML, SachsR, MalcomL. Instrumented measurement of anterior knee laxity in patients with acute anterior cruciate ligament disruption. Am J Sports Med1985;13:401–407.CrossrefPubMed Google Scholar
8 Forster IW , Warren-SmithCD, TewM. Is the KT1000 knee ligament arthrometer reliable?J Bone Joint Surg [Br]1989;71-B:843–847.CrossrefPubMed Google Scholar
9 Bach BR Jr , WarrenRF, FlynnWM, KrollM, WickiewieczTL. Arthrometric evaluation of knees that have a torn anterior cruciate ligament. J Bone Joint Surg [Am]1990;72-A:1299–1306.PubMed Google Scholar
10 Wiertsema SH , van HooffHJ, MigchelsenLA, SteultjensMP. Reliability of the KT1000 arthrometer and the Lachman test in patients with an ACL rupture. Knee2008;15:107–110.CrossrefPubMed Google Scholar
11 Branch TP , MayrHO, BrowneJE, et al.Instrumented examination of anterior cruciate ligament injuries: minimizing flaws of the manual clinical examination. Arthroscopy2010;26:997–1004.CrossrefPubMed Google Scholar
12 Kuroda R , HoshinoY, ArakiD, et al.Quantitative measurement of the pivot shift, reliability, and clinical applications. Knee Surg Sports Traumatol Arthrosc2012;20:686–691.CrossrefPubMed Google Scholar
13 Hoshino Y , KurodaR, NagamuneK, et al.In vivo measurement of the pivot-shift test in the anterior cruciate ligament-deficient knee using an electromagnetic device. Am J Sports Med2007;35:1098–1104.CrossrefPubMed Google Scholar
14 Araki D , KurodaR, KuboS, et al.The use of an electromagnetic measurement system for anterior tibial displacement during the Lachman test. Arthroscopy2011;27:792–802.CrossrefPubMed Google Scholar
15 Robert H , NouveauS, GageotS, GagniereB. A new knee arthrometer, the GNRB: experience in ACL complete and partial tears. Orthop Traumatol Surg Res2009;95:171–176.CrossrefPubMed Google Scholar
16 Zaffagnini S , BignozziS, MartelliS, et al.New intraoperative protocol for kinematic evaluation of ACL reconstruction: preliminary results. Knee Surg Sports Traumatol Arthrosc2006;14:811–816.CrossrefPubMed Google Scholar
17 Martelli S , ZaffagniniS, BignozziS, LopomoN, MarcacciM. Description and validation of a navigation system for intra-operative evaluation of knee laxity. Comput Aided Surg2007;12:181–188.CrossrefPubMed Google Scholar
18 Bignozzi S , ZaffagniniS, LopomoN, et al.Clinical relevance of static and dynamic tests after anatomical double-bundle ACL reconstruction. Knee Surg Sports Traumatol Arthrosc2010;18:37–42.CrossrefPubMed Google Scholar
19 Yamamoto Y , IshibashiY, TsudaE, et al.Comparison between clinical grading and navigation data of knee laxity in ACL-deficient knees. Sports Med Arthrosc Rehabil Ther Technol2010;2:27.CrossrefPubMed Google Scholar
20 Clarke JV , RichesPE, PicardF, DeakinAH. Non-invasive computer-assisted measurement of knee alignment. Comput Aided Surg2012;17:29–39.CrossrefPubMed Google Scholar
21 Clarke JV , WilsonWT, WearingSC, et al.Standardising the clinical assessment of coronal knee laxity. Proc Inst Mech Eng H2012;226:699–708.CrossrefPubMed Google Scholar
22 Russell DF, Deakin AH, Fogg QA, Picard F. Noninvasive quantification of knee joint kinematics [abstract]. CAOS International Congress, 2012. Google Scholar
23 Markolf KL , Graff-RadfordA, AmstutzHC. In vivo knee stability: a quantitative assessment using an instrumented clinical testing apparatus. J Bone Joint Surg [Am]1978;60-A:664–674. Google Scholar
24 Markolf KL , KochanA, AmstutzHC. Measurement of knee stiffness and laxity in patients with documented absence of the anterior cruciate ligament. J Bone Joint Surg [Am]1984;66-A:242–252.PubMed Google Scholar
25 Shrout PE , FleissJL. Intraclass correlations: uses in assessing rater reliability. Psychol Bull1979;86:420–428.CrossrefPubMed Google Scholar
26 Portney LG, Watkins MP. Foundations of clinical research: applications to practice. Norwalk: Appleton & Lange, 1993:722. Google Scholar
27 Fleiss JL. Statistical methods for rates and proportions. Second ed. New York: Wiley, 1981:321. Google Scholar
28 Bland JM , AltmanDG. Statistical methods for assessing agreement between two methods of clinical measurement. Lancet1986;1:307–310.PubMed Google Scholar
29 Arneja S , LeithJ. Review article: Validity of the KT-1000 knee ligament arthrometer. J Orthop Surg (Hong Kong)2009;17:77–79.CrossrefPubMed Google Scholar
30 Kocher MS , SteadmanJR, BriggsK, et al.Determinants of patient satisfaction with outcome after anterior cruciate ligament reconstruction. J Bone Joint Surg [Am]2002;84-A:1560–1572.CrossrefPubMed Google Scholar
31 Ristanis S , GiakasG, PapageorgiouCD, et al.The effects of anterior cruciate ligament reconstruction on tibial rotation during pivoting after descending stairs. Knee Surg Sports Traumatol Arthrosc2003;11:360–365.CrossrefPubMed Google Scholar
32 Lorbach O , WilmesP, MaasS, et al.A non-invasive device to objectively measure tibial rotation: verification of the device. Knee Surg Sports Traumatol Arthrosc2009;17:756–762.CrossrefPubMed Google Scholar
33 Jenny JY , BoeriC. Low reproducibility of the intra-operative measurement of the transepicondylar axis during total knee replacement. Acta Orthop Scand2004;75:74–77.CrossrefPubMed Google Scholar
34 Koh J . Computer-assisted navigation and anterior cruciate ligament reconstruction: accuracy and outcomes. Orthopedics2005;28(Suppl):1283–1287.CrossrefPubMed Google Scholar
35 Yau WP , LeungA, ChiuKY, TangWM, NgTP. Intraobserver errors in obtaining visually selected anatomic landmarks during registration process in nonimage-based navigation-assisted total knee arthroplasty: a cadaveric experiment. J Arthroplasty2005;20:591–601.CrossrefPubMed Google Scholar
36 Graham GP , JohnsonS, DentCM, FaircloughJA. Comparison of clinical tests and the KT1000 in the diagnosis of anterior cruciate ligament rupture. Br J Sports Med1991;25:96–97.CrossrefPubMed Google Scholar
37 Isberg J , FáxenE, BrandssonS, et al.KT-1000 records smaller side-to-side differences than radiostereometric analysis before and after an ACL reconstruction. Knee Surg Sports Traumatol Arthrosc2006;14:529–535.CrossrefPubMed Google Scholar
38 Oh YK , KreinbrinkJL, Ashton-MillerJA, WojtysEM. Effect of ACL transection on internal tibial rotation in an in vitro simulated pivot landing. J Bone Joint Surg [Am]2011;93-A:372–380.CrossrefPubMed Google Scholar
39 Noyes FR , GroodES, CummingsJF, WrobleRR. An analysis of the pivot shift phenomen: the knee motions and subluxations induced by different examiners. Am J Sports Med1991;19:148–155. Google Scholar
40 Christel PS , AkgunU, YasarT, KarahanM, DemirelB. The contribution of each anterior cruciate ligament bundle to the Lachman test: a cadaver investigation. J Bone Joint Surg [Br]2012;94-B:68–74.CrossrefPubMed Google Scholar
41 Andersen HN , Dyhre-PoulsenP. The anterior cruciate ligament does play a role in controlling axial rotation in the knee. Knee Surg Sports Traumatol Arthrosc1997;5:145–149.CrossrefPubMed Google Scholar
42 McQuade KJ , CrutcherJP, SidlesJA, LarsonRV. Tibial rotation in anterior cruciate deficient knees: an in vitro study. J Orthop Sports Phys Ther1989;11:146–149.CrossrefPubMed Google Scholar
43 Lane JG , IrbySE, KaufmanK, RanggerC, DanielDM. The anterior cruciate ligament in controlling axial rotation: an evaluation of its effect. Am J Sports Med1994;22:289–293. Google Scholar
44 Nielsen S , OvesenJ, RasmussenO. The anterior cruciate ligament of the knee: an experimental study of its importance in rotatory knee instability. Arch Orthop Trauma Surg1984;103:170–174.CrossrefPubMed Google Scholar
45 Lipke JM , JaneckiCJ, NelsonCL, et al.The role of incompetence of the anterior cruciate and lateral ligaments in anterolateral and anteromedial instability: a biomechanical study of cadaver knees. J Bone Joint Surg [Am]1981;63-A:954–960. Google Scholar
46 Diermann N , SchumacherT, SchanzS, et al.Rotational instability of the knee: internal tibial rotation under a simulated pivot shift test. Arch Orthop Trauma Surg2009;129:353–358.CrossrefPubMed Google Scholar
47 Gollehon DL , TorzilliPA, WarrenRF. The role of the posterolateral and cruciate ligaments in the stability of the human knee: a biomechanical study. J Bone Joint Surg [Am]1987;69-A:233–242. Google Scholar
48 Grood ES , StowersSF, NoyesFR. Limits of movement in the human knee: effect of sectioning the posterior cruciate ligament and posterolateral structures. J Bone Joint Surg [Am]1988;70-A:88–97. Google Scholar
49 Georgoulis AD , RistanisS, ChouliarasV, MoraitiC, StergiouN. Tibial rotation is not restored after ACL reconstruction with a hamstring graft. Clin Orthop Relat Res2007;454:89–94. Google Scholar
50 Branch TP , BrowneJE, CampbellJD, et al.Rotational laxity greater in patients with contralateral anterior cruciate ligament injury than healthy volunteers. Knee Surg Sports Traumatol Arthrosc2010;18:1379–1384.CrossrefPubMed Google Scholar
51 Bedi A , MusahlV, O’LoughlinP, et al.A comparison of the effect of central anatomical single-bundle anterior cruciate ligament reconstruction and double-bundle anterior cruciate ligament reconstruction on pivot-shift kinematics. Am J Sports Med2010;38:1788–1794.CrossrefPubMed Google Scholar
52 Lane CG , WarrenRF, StanfordFC, KendoffD, PearleAD. In vivo analysis of the pivot shift phenomenon during computer navigated ACL reconstruction. Knee Surg Sports Traumatol Arthrosc2008;16:487–492.CrossrefPubMed Google Scholar
53 Musahl V , BediA, CitakM, et al.Effect of single-bundle and double-bundle anterior cruciate ligament reconstructions on pivot-shift kinematics in anterior cruciate ligament- and meniscus-deficient knees. Am J Sports Med2011;39:289–295.CrossrefPubMed Google Scholar
54 Pearle AD , KendoffD, MusahlV, WarrenRF. The pivot-shift phenomenon during computer-assisted anterior cruciate ligament reconstruction. J Bone Joint Surg [Am]2009;91-A(Suppl 1):115–118.CrossrefPubMed Google Scholar
55 Suero EM , CitakM, ChoiD, et al.Software for compartmental translation analysis and virtual three-dimensional visualization of the pivot shift phenomenon. Comput Aided Surg2011;16:298–303.CrossrefPubMed Google Scholar
Funding statement:
The first author received a grant to aid career development in orthopaedic research from the West of Scotland Orthopaedic Research Society. The first author received material and software support necessary to carry out this study from BBraun Aesculap (Tuttlingen, Germany). The Department of Orthopaedics at the Golden Jubilee National Hospital receives research funding from a variety of orthopaedic manufacturers including BBraun Aesculap, however no industry funding was received for this study.
Author contributions:
D. F. Russell: Design of the experimental set-up, Data collection, Data analysis, Writing the paper
A. H. Deakin: Study design, Data analysis, Writing the paper
Q. A. Fogg: Supervision of design of experimental set-up, Data collection, Review of manuscript
F. Picard: Study concept, Study design, Review of data analysis, Review and editing of manuscript
ICMJE Conflict of Interest:
Professor F. Picard has licences and patents with BBraun Aesculap. The Department of Orthopaedics receives research funding from a number of companies including BBraun Aesculap.
©2013 The British Editorial Society of Bone & Joint Surgery. This is an open-access article distributed under the terms of the Creative Commons Attributions licence, which permits unrestricted use, distribution, and reproduction in any medium, but not for commercial gain, provided the original author and source are credited.