Abstract
As our understanding of hip function and disease improves, it is evident that the acetabular fossa has received little attention, despite it comprising over half of the acetabulum’s surface area and showing the first signs of degeneration. The fossa’s function is expected to be more than augmenting static stability with the ligamentum teres and being a templating landmark in arthroplasty. Indeed, the fossa, which is almost mature at 16 weeks of intrauterine development, plays a key role in hip development, enabling its nutrition through vascularization and synovial fluid, as well as the influx of chondrogenic stem/progenitor cells that build articular cartilage. The pulvinar, a fibrofatty tissue in the fossa, has the same developmental origin as the synovium and articular cartilage and is a biologically active area. Its unique anatomy allows for homogeneous distribution of the axial loads into the joint. It is composed of intra-articular adipose tissue (IAAT), which has adipocytes, fibroblasts, leucocytes, and abundant mast cells, which participate in the inflammatory cascade after an insult to the joint. Hence, the fossa and pulvinar should be considered in decision-making and surgical outcomes in hip preservation surgery, not only for their size, shape, and extent, but also for their biological capacity as a source of cytokines, immune cells, and chondrogenic stem cells.
Cite this article: Bone Joint Res 2020;9(12):857–869.
Article focus
-
This study aimed to determine the function of the acetabular fossa.
-
The morphology and physiology of the fossa may play a role in decision-making for hip preservation surgery.
-
We aimed to determine if the acetabular fossa has a biological capacity of response to pre-arthritic and arthritic hip disease.
Key messages
-
The acetabular fossa is more than an inert acetabular surface.
-
The shape and relative size of the fossa with regards to the articular cartilage may preclude functional outcomes in hip preservation surgery.
-
The acetabular fossa is a source of cytokines, immune cells, and chondrogenic stem cells.
Strengths and limitations
-
This is an in-depth review of the embryology, anatomy, histology, radiology, function, and biology of the acetabular fossa.
-
The histology of the fossa was only described in the context of osteoarthritis or in newborns with hip dysplasia.
-
Biological function of the fossa is mostly described in proinflammatory scenarios.
Introduction
Our understanding of the native hip’s mechanics, physiology, and pathology has dramatically improved over the last two decades.1-6 This has been facilitated by the introduction of open and arthroscopic procedures to the native hip and the advancements in engineering and basic sciences. Although a clear association between abnormal mechanics and osteoarthritis (OA) development exists,7 there is little doubt that genetic predisposition is important8,9 and that the biological cascade leading to OA is not clearly understood or defined.10,11
In the last two decades most of the focus on the origins and treatment of pre-arthritic hip disease has been on acetabular rim pathology/degeneration.12,13 However, clinical observations often show central acetabular osteophytes as an early manifestation of hip arthritis, which are significantly associated with the degree of chondral damage and size of the articular defects.14
An area of the hip joint that has received relatively little attention is the acetabular fossa. It is composed of the ligamentum teres and adipose tissue (pulvinar). Lesions of the acetabular fossa are an uncommon cause of hip pain when diagnosed in isolation. Such lesions classically refer to injuries to the ligamentum teres (complete or partial tears), and rarely to synovitis of the fat pad, plicae, or fibrosis of the fibro-connective tissue.15,16 Byrd15 described the pulvinar tissue within the acetabular fossa as ‘a canary in a coal mine’ since various arthritic and pre-arthritic hip disorders, which usually affect the hip cartilage and labrum, can initially damage this particular area.17
The acetabular fossa seems to be more than just an inert acetabular surface. A holistic knowledge of the morphology and physiology of the acetabulum is crucial for surgeons and scientists in the field of hip joint preservation. This report aims to provide a comprehensive overview of the embryology, anatomy, histology, radiology, function (mechanical and physiological), and role in clinical decision-making of the acetabular fossa as currently described in literature.
Embryonic and postnatal development of the acetabulum and acetabular fossa
The hip joint derives from the blastematous cells that differentiate from primordial mesoderm into pre-cartilage and foetal bone.18 At four weeks, the limb buds develop as a cluster of three-layered mesenchymal tissue at the lateral parts of the embryonic trunk (lateral plate mesoderm).19 In the interzone (prospective joint), a densely cellular inner layer progressively becomes thinner and evolves into a number of tissues including: 1) articular cartilage of both the femoral head and the acetabulum; 2) the ligamentum teres; 3) the pulvinar; 4) the joint’s synovial membrane; and 5) the labrum.20-23
During the embryonic period, the acetabular fossa undergoes substantial macroscopic and histological changes.22,24 The fossa is the first acetabular structure to become vascularized, allowing for further development of the peripheral articular cartilage through invasion of chondrogenic cells (Table I).22 Such differentiation relies on complex genetic and molecular signalling processes.25-27
Table I.
Ontogeny of the acetabular fossa.
Time of intrauterine development | Stage of development | Stage of acetabular development | Stage of fossa development | Stage of cartilage development | Molecular development |
---|---|---|---|---|---|
4 weeks |
![]() |
|
|
|
|
8 weeks |
![]() |
|
|
|
|
12 weeks |
![]() |
|
|
|
|
16 weeks |
![]() |
|
|
|
|
Last 6 months |
![]() |
|
|
|
|
-
IAAT, intra-articular adipose tissue
During the last six months (foetal period), there is a six-fold increase in size until birth. At birth, the articular cartilage is mostly located posteriorly (at the ischium), whereas the nonarticular fossa is sited anteriorly.28 After birth, the Y-shaped triradiate cartilage defines the final depth of the acetabulum.
The development of the articular cartilage (by apposition) in the weight-bearing area requires the constant dynamic stimulus of the femoral head.29,30 The acetabular dome is derived from the iliac centre of ossification; the posterior and anterior walls originate from the ischial ossification centre and the anterior os acetabuli, respectively.31 The ilium and ischium are the first to fuse followed by the ilium and pubis that begin to fuse superomedially and progress downwards to the midpoint of the acetabulum. Finally, the pubis and ischium fuse from their inner margin outwards. At the apex of the fossa, some original blastemal connective junctions remain over a still unfused Y.19
Section key points
-
The fossa is the first acetabular structure to become vascularized, allowing for further development of the peripheral articular cartilage.
-
At 16 weeks, the fossa is mature and invaded by synovium-like cells at the pulvinar, surrounded by highly vascularized articular adipose tissue.
Gross anatomy and histology of the adult acetabular fossa
Gross anatomy
The hip is a ball-and-socket diarthrodial joint between the acetabulum and the proximal femur. The acetabulum is a rounded vault with a mean diameter of 52 mm (SD 4),32 however variations do exist depending on sex and race.33-36 The joint has two distinct parts: the acetabular fossa (i.e. no articular cartilage) and a peripheral horseshoe-shaped articulating surface, lined with cartilage that embraces the fossa circumferentially except for its distal aspect (six o’clock position), where the acetabular notch becomes a foramen by the presence of the transverse acetabular ligament (TAL). The acetabular fossa is filled with the ligamentum teres and the pulvinar (Figure 1), which is composed of fatty tissue (intra-articular adipose tissue (IAAT)) and a synovial membrane. The pulvinar has a thickness of 2 mm to 4.4 mm.37 In order to match the acetabulum, the femoral head comprises two-thirds of a sphere, with a medial nonarticulating small groove (fovea capitis) for the ligamentum teres.38
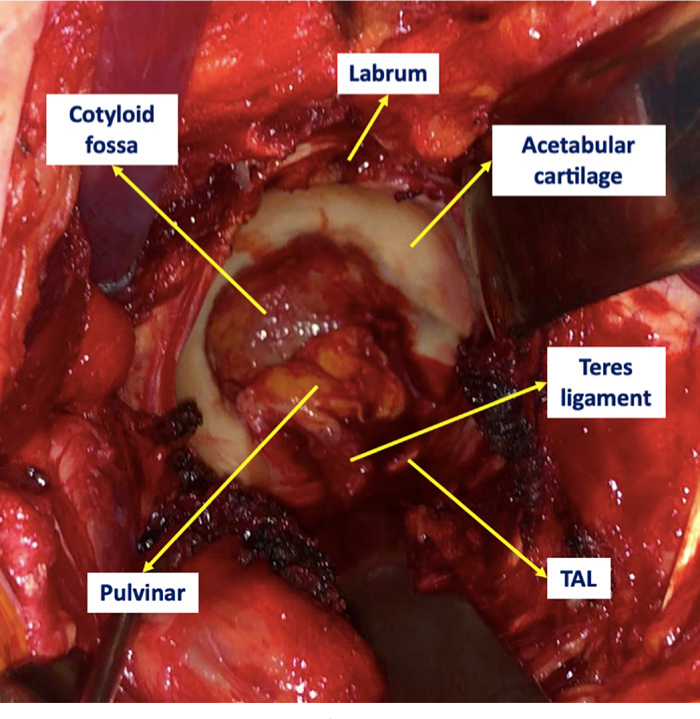
Fig. 1
Clinical image of the gross anatomy of a nonarthritic acetabulum from a 75-year-old male diagnosed with a right neck of femur fracture.
The mean size of the acetabular fossa is 26.1 mm (SD 6.5) by 33.9 mm (SD 6.7),39 with the ligament’s widest diameter being smaller than the fossa’s diameter. The quadrilateral plate represents the bottom of the fossa and is the place where the closed triradiate cartilage converges. The term ‘acetabular point’ corresponds with the point of the fusion lines for each bony element at the level of the acetabular fossa, represented in fused pelves by the indentation between the superior and the anterior lobes of the acetabular fossa.40
Govsa et al41 identified four different types of acetabular fossae with regards to their shape: type 1, with a cloverleaf form, present in 60% of cases (137/226); type 2, with a semicircular shape and a prevalence of 29% (65/226); type 3, spongy-type, present in 2% of cases (4/226); and type 4, with an isolated defect above the superior lobe seen in 9% of cases (20/226). The shape of the fossa determines the corresponding shape and rigidity of the peripheral cartilaginous surface (oval, piriform, or elongated),42 which ultimately defines the biomechanics of the joint.43 The relative proportions of the articular and nonarticular surfaces of the acetabulum vary depending on the acetabular shape (Figure 2). The acetabular fossa is reported to comprise over two-thirds (64% to 73%) of the acetabular area in acetabula with normal morphological parameters.
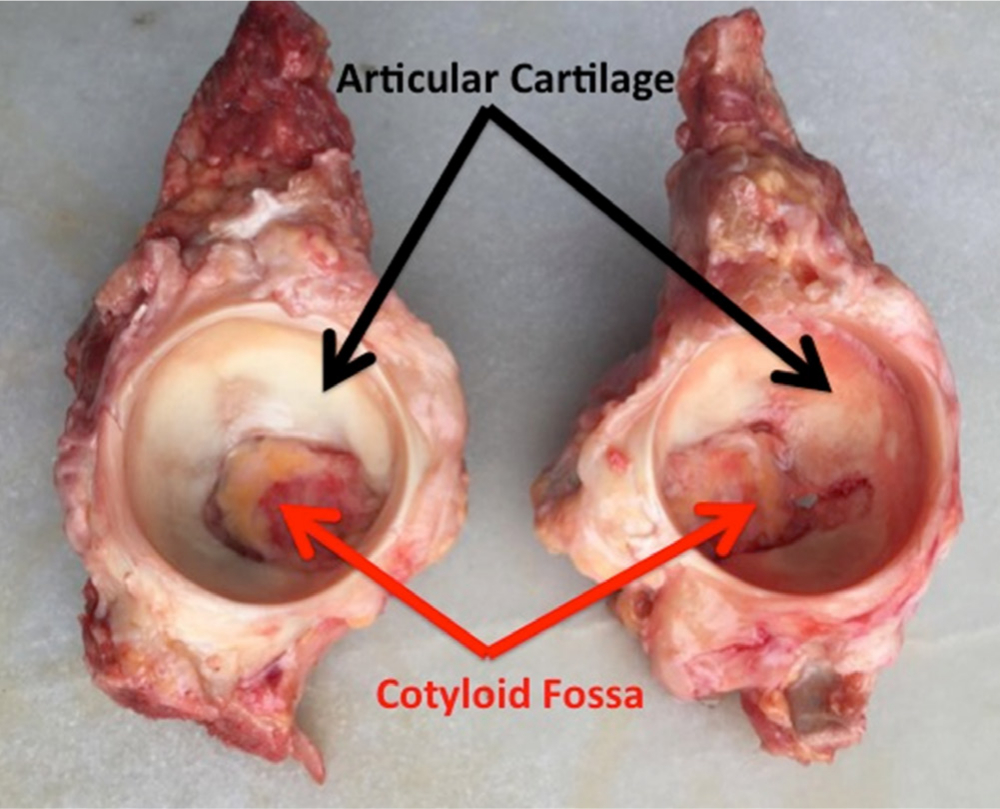
Fig. 2
Cadaver model of the acetabulum demonstrating different shapes of acetabular fossa and articular cartilage.
Both the margins of the notch and the TAL serve for the attachment of the ligamentum teres through five different fascicles (anterior notch, posterior notch, floor of the fossa, TAL, and inferior capsule).21 The ligament is a fibrous connective band with a pyramidal shape, with the base facing the acetabular fossa and the TAL.44 It is composed of collagen fibres and fibrous and adipose tissue, embraced by synovium and blood vessels of diverse diameters.45 In almost 75% of cases, the loose, tent-shaped synovial membrane covering the distal two-thirds of the fossa and the intra-articular fat pad advances towards the ligament like a sleeve and inserts in the fovea capitis femoris.46,47 Usually, there is a frenulum-like superior synovial fold at the base of the ligament;48,49 this well-padded fibroelastic plicae is a potential pitfall when diagnosing a partially torn ligament.
Blood supply to the fossa is provided by the obturator artery, with a few branches penetrating the quadrilateral plate, running through the fat pad and irrigating both walls as well as the acetabular dome.50 The number of vascular foramina varies.41 Additionally, the fossa has numerous proprioceptive nerve branches derived from the sciatic and obturator nerves.51-53
A constant pattern of changes in the acetabular morphology with ageing allows both the lunate surface and the acetabular fossa to act as age estimators.54,55 Rissech et al56 suggested to measure the following post-mortem parameters: acetabular groove, rim shape, rim porosity, apex cavity, activity on the outer edge of the fossa, activity of the fossa, and porosity of the fossa (acetabular fossa texture).56-58 The pulvinar is likely to be associated with these age-related changes seen in the acetabular fossa.57
Histological findings
Little is known on the histology of the pulvinar in the absence of arthritis or in pre-arthritic disorders as well as during cellular differentiation in phases of development. The composition of the pulvinar’s IAAT may resemble other well-described IAATs (Table II) such as the Hoffa’s fat pad; a white adipose tissue composed of mature adipocytes, fibroblasts, macrophages, and other leucocytes, including abundant mast cells,59 grounded in an extracellular matrix of lax connective tissue and rich capillaries.60 The acetabular fossa contents have mostly been described in the context of OA or in newborns with hip dysplasia.
Table II.
Characteristics of intra-articular adipose tissue versus white adipose tissue.
Characteristic | IAAT | WAT |
---|---|---|
Location | Pulvinar of the hip, Hoffa’s fat pad in the knee, lumbar facet, intermetacarpal, olecranon, and coronoid fossa | Subcutaneous, viscera, mediastinum, muscles |
Thickness | 2.0 mm to 4.4 mm | 1.65 mm to 18 mm |
Cellularity | Mature adipocytes, fibroblasts, macrophages and other leucocytes, mast cells, and mesenchymal stem cells | Mature adipocytes, pericytes, adipose precursor cells, leucocytes, mesenchymal progenitor cells |
Vascularization | Rich capillaries (derived from obturator artery in case of the pulvinar) | Diverse |
Innervation | Branches from obturator and sciatic nerves in case of the hip. Peptidergic C-fibres | Diverse. Sympathetic nerve (tyrosine hydroxylase immunoreactive (TH+) fibres |
Most relevant cytokines produced | IL-6, IL-8, PGE2, leptin, adiponectin, resistin, adipsin, ghrelin | TNF-alpha, IL-6, IL-8, PGE2, leptin, adiponectin, resistin, adipsin, ghrelin, visfatin, chemerin, VEGF, HGF, TGF-beta, FGF |
Role | Sensory regulation, load transmission, synovial fluid production, development and growth (in case of the acetabulum), local regulation of inflammatory response | Lipid and glucose (insulin) metabolism, coagulation, appetite and body weight regulation, reproduction and fertility, mechanical protection, temperature regulation, bone marrow metabolism, immune response modulator, systemic inflammatory response |
Variation in gross morphology during metabolic disorders (obesity, metabolic syndrome, lipodystrophy, cachexia) | No | Yes |
Variation in cellular composition and cytokine secretion during metabolic disorders | Yes, adopting a proinflammatory profile | Yes |
-
FGF, fibroblast growth factor; HGF, hepatocyte growth factor; IAAT, intra-articular adipose tissue; IL, interleukin; PGE2, prostaglandin E2; TGF, transforming growth factor; TNF, tumour necrosis factor; VEGF, vascular-endothelial growth factor; WAT, white adipose tissue.
Synovium
Although the synovium usually lines the spaces of diarthrodial joint, tendon sheaths, and bursae, it can also be located intra-articularly (i.e. lining the fossa’s fat pad). It has two layers: the most superficial one (intima) consisting of macrophages and fibroblasts; whereas the deeper (subintima) is filled with fibro-cartilage and vessels in a lax or dense extracellular matrix with scarce fibroblasts.61 Adipose synovium is generally organized in pads, separated by thin fibrous layers and infiltrated with vascular villi associated with mast cells.62 Synovial macrophages have non-specific esterase activity and are positive for CD163, CD68, CD14, and CD45, as well as for immunoglobulin receptor FcgRIIIa, associated with several rheumatic and synovial diseases.63,64
The fossa as a neuroendocrine organ
After performing immunohistochemistry using the polyclonal antibody against the S-100 protein, Leunig et al53 reported that the base of the ligamentum teres had an abundance of unmyelinated type IVa receptors, with a frequency of 5 to 54 per 50 mm2. These receptors are thought to be in charge of transmitting detailed somatosensory afferent signals to the spinocerebral regulatory systems. In the setting of nonarthritic or degenerative joint disease, mediators such as histamine, bradykinin, or prostaglandin E2 (PGE2) can activate nociceptive nerve endings, triggering the hip pain found in these cases.65,66 However, whether the pulvinar has neuroreceptors is presently unknown.67
After collecting samples during total knee arthroplasty performed in lean and obese patents, Harasymowicz et al68 reported that unlike non-obese patients, those with obesity had the patellar fat pad infiltrated with larger adipocytes (than in the synovium) and the synovial IAAT with increased fibrosis and macrophage infiltration. Additionally, both tissues showed significantly more CD45+ CD14+ total macrophages, and CD14+ CD206+ M2 type macrophages and a significant reduction in the expression of peroxisome proliferator-activated receptor gamma (PPARγ), which plays a key role in lipid homeostasis.69 These findings suggest that body weight may have a role in the expression of intra-articular adipose-related markers with a proinflammatory profile.68
Eymard et al59 phenotyped the acetabular IAAT biopsied during total hip arthroplasty (THA) and compared it to that of subcutaneous adipose tissue (SCAT). The authors found an osteoarthritic-induced inflammatory pattern in the hip’s fat pad that had only been described in the knee (infrapatellar fat pad).70,71 Unlike SCAT, and independently of sex or body mass index, IAAT showed increased fibrosis, vascularization, and leucocyte infiltration, with a significantly higher expression and production of inflammatory factors including IL-6, IL-8, and PGE2. In this sense, the synovium surrounding these fat pads should be considered as a distinctive functional unit,72 rather than an inert fold of fibrous tissue.
The fossa as a stem cell reservoir
Arthroscopic-assisted autologous chondrocyte transplantation (ACT) is a promising minimally invasive alternative for the treatment of full-thickness chondral injuries in the hip.73,74 Recent experimental studies have used the acetabular fossa for cell-based therapies.
In a retrospective study comparing ACT with cartilage debridement for traumatic chondral injuries of around 2 cm2, Fontana et al75 supported the cell-based approach as it significantly improved the clinical scores when compared to arthroscopic debridement. In this study, the peripheral area of the pulvinar was harvested to seek chondrocytes that were later cultured on resorbable gel-polymer scaffolds of polyglycolic and polylactic acid in order to promote 3D growth.
The acetabular fossa is also a reservoir of mesenchymal stem cells (MSCs). Murata et al76 compared the different characteristics of MSCs derived from the synovial tissue of the hip joint in patients with femoroacetabular impingement (FAI) obtained from two sites, the paralabral region and the fossa. Histology findings revealed that although cellular morphology was similar between the two sites, the number of colony-forming units (CFUs) per 104 nucleated cells from the fossa were markedly higher than from the paralabral area, presenting also an increased osteogenic (by expressing higher levels of COL1a1 and BGLAP genes) and chondrogenic potential (with a higher expression of COL2a1 and SOX9 genes).76 Thus, it appears that acetabular fossa-derived MSCs could be more suitable for stem cell therapies than paralabral-MSCs.
Other studies support the use of IAAT-derived stem cells for articular cartilage repair or regeneration. Hindle et al77 showed that pericytes from the stromal-vascular fraction of the infrapatellar fat pad were a better source of chondrogenic cells compared with bone marrow. Similarly, Liu et al78 demonstrated the use of infrapatellar fat pad-derived stem cells for tissue engineering of articular cartilage of a clinically relevant size.
The fossa in congenital hip dislocation
Some histological differences have been described in the ligamentum teres of patients with hip dysplasia when compared to controls. Ippolito et al79 described a thicker and longer appearance of the ligamentum teres in dysplastic hips, together with wide areas of fibro-cartilaginous metaplasia, mostly seen at the superolateral capsule and within the substance of the ligamentum teres in paediatric cases with congenital dislocation of the hip. Sarban et al52 described that, unlike sub-luxated hips, the ligamentum teres of congenitally dislocated hips had an irregular cellular distribution, with different shapes and in different stages of degeneration. Additionally, completely dislocated hips evidenced thicker and more numerous collagen and elastic fibres, as well as a greater deposit of hyaline matrix.52
The fossa in the setting of osteoarthritis
The fossa is a common location of acetabular osteophytes in the setting of degenerative changes.80 Osteophyte analysis has been well described in the knee joint. Osteophytes extracted from knees showed significant expression of carboxypeptidase A3 (CPA3) and membrane-spanning 4-domains subfamily A member 2 (MS4A2), indicating a considerable participation of mast cells, and expression of phospholipase A2, group IIA (PLA2G2A), suggesting prostaglandin synthesis and activation of the inflammatory cascade.81 These findings are in line with those described by Eymard et al59 who reported that tryptase-positive cells (mast cells) were densely observed in the perivascular area of the IAAT of osteoarthritic hips and knees. Such proinflammatory cascade seen in fossas with degenerative disease is also observed in the cartilage area of both pre-arthritic and osteoarthritic hips (Figure 3).82
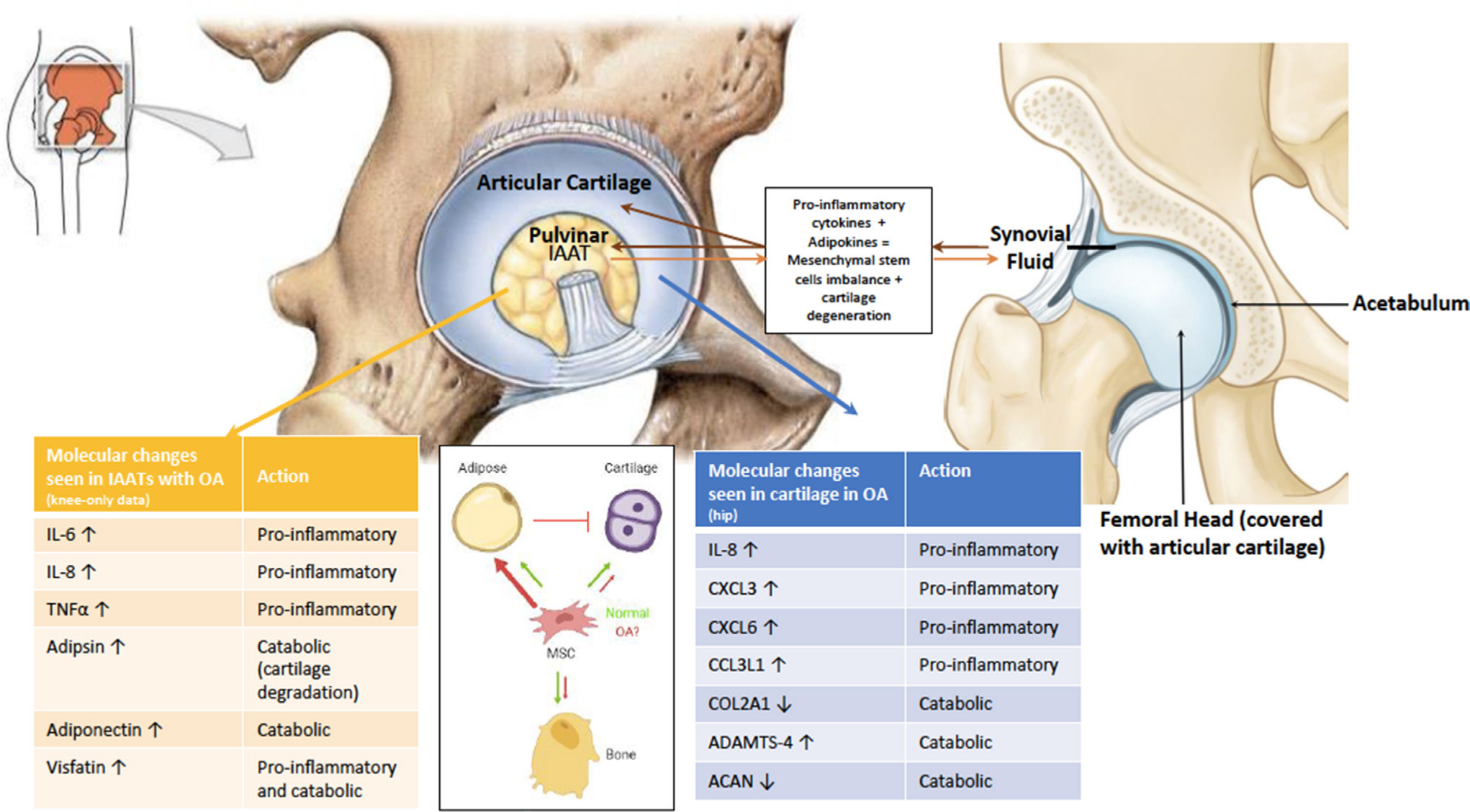
Fig. 3
Cellular and molecular changes in the pulvinar leading to osteoarthritis (OA) development. Recent studies highlighted the proinflammatory role of intra-articular adipose tissue (IAAT) in the development of OA. Our hypothesis is that these changes cause an imbalance in the differentiation of mesenchymal stem cells (MSCs), biasing towards the adipocyte lineage at the expense of the osteoblast and chondrocyte lineages. Adipokines combined with proinflammatory cytokines accumulate in the synovial fluid to further accelerate cartilage degradation and degeneration. Arrows refer to upregulation and downregulation. ACAN, aggrecan; ADAMTS-4, a disintegrin and metalloproteinase with thrombospondin motifs 4; CCL3L1, C-C motif chemokine ligand 3 like 1; COL2A1, collagen type 2 alpha 1; CXCL3, chemokine C-X-C motif ligand 3; IL, interleukin.
Sampatchalit et al83 described gradual acetabular fossa changes in 11 cadaveric specimens with degenerative, osteoarthritic changes. The authors found foci of fat necrosis and fibrous strings, of variable thickness, in all cases. Changes related to OA included a decrease in the volume of the adipose tissue, fibro-cartilaginous and/or chondroid focal metaplasia, along with intra-substance calcifications. Based on these findings, the authors further created a three-stage classification that correlates with progressive stages of articular cartilage damage.83
Section key points
-
The relative proportions of the articular and nonarticular surfaces of the acetabulum vary depending on the acetabular shape.
-
A constant pattern of changes in the acetabular morphology with ageing allows both the lunate surface and the acetabular fossa to act as age estimators.
-
Being slightly different to SCAT, the composition of the pulvinar’s IAAT includes synovial fibroblasts and macrophages, mast cells, as well as acetabular fossa-derived MSCs.
-
Unlike SCAT, IAAT acts like a distinctive functional unit showing increased fibrosis, vascularization, and leucocyte infiltration, with a significantly higher expression and production of inflammatory factors including IL-6, IL-8, and PGE2 in osteoarthritic models.
Radiological findings
The acetabular fossa can be easily interpreted on plain radiographs. On an anteroposterior pelvic radiograph, the acetabular fossa extends from the teardrop to an ill-defined area between the medial sourcil and the superior edge of the fovea capitis. Its distal part (at the level of the teardrop) is an important radiological marker of acetabular depth.84 Coxa profunda exists when the acetabular fossa touches or crosses the ilioischial line,85 whereas protrusio acetabuli means that the femoral head touches or crosses the ilioischial line. 86Coxa profunda can be seen in up to 76% of asymptomatic patients (25/33), with acetabular over-coverage (lateral centre-edge angle > 40° or Tönnis angle < 0°) detected in only 22% of all coxa profunda hips (19/86).87
As previously mentioned, the acetabular fossa is a distinctive area in which osteophytes develop.88 The prevalence of central acetabular osteophytes is as frequent as that of marginal ones or those found in the femoral head.80 They can be present in Tönnis 0 hips89 and can be detected as saber-tooth bony excrescence on cross-sectional imaging or as a double acetabular medial wall on plain radiographs.90 Their recognition is important as they are an independent factor associated with reduced chances of hip preservation surgery for the treatment of symptomatic FAI.89 When detected intraoperatively, favourable results have been reported with arthroscopic debridement/notchplasty.91,92
Cross-sectional imaging, including CT and MRI, is an important complimentary tool for assessing the morphology of the acetabular fossa. Leunig et al93 reported on specific hip preservation cases with protrusio acetabuli with MRI slices showing that the acetabular fossa extended far into the weight-bearing zone of the roof. This finding, initially described by Dunlop et al94 and untraceable on plain radiographs, might jeopardize the indication of rim trimming that would further reduce the cartilaginous weight-bearing zone. Axial views of the MRI are also useful to detect saber-tooth osteophytes rising from the acetabular fossa.89 MRI arthrography is a sensitive tool to identify the changes that occur with OA within the fossa as described by Sampatchalit et al.83
Lastly, the ‘acetabular fossa hot spot’ was described by Kubicki et al95 in a positron emission tomography (PET)/CT study of asymptomatic volunteers. The prevalence is low (0.36%) and the aetiology is unclear; it brings to light the fact that increased cellular activity may take place within the fossa.
Section key points
-
The fossa can be easily interpreted on plain radiographs, and is a distinctive area in which osteophytes develop.
-
Its morphology and position in the acetabulum can help define femoral head coverage and acetabular volume (e.g. coxa profunda, coxa protrusio).
-
Cross-sectional imaging can further delineate the limits of the fossa with the weight-bearing zone.
-
Saber-tooth osteophytes located at the fossa, which are associated with inferior outcomes of hip preservation surgery, can be easily identified with axial views of CT/MRI scans.
Function of the acetabular fossa
Mechanical
Mechanical loads (compressive vs tensile forces) can have an effect on cellular and molecular components of bony structures.96 The acetabular fossa has important roles in the dynamic function of the acetabulum. Greenwald et al97 studied how load is transmitted in the hip joint. The two columns of the pelvis, configured in an inverted Y, allow for dynamic deformation of the acetabular walls with increasing load bearing. At lower loads, only the anterior and posterior walls transmit force, and the acetabular dome has no contact with the femoral head. With increasing loads, the two columns gradually separate deforming both walls, thus allowing the dome to absorb force. Lazennec et al42 further assessed how the acetabulum absorbed the load during gait. Since the anterior wall is more rigid than the posterior one, it is the latter that deforms the most.42 For a given load, there are asymmetric anterior and posterior contact surfaces between the femur and the acetabulum, also promoting differential mobility of the anterior and posterior acetabular horns, causing a subsequent widening of the acetabular notch.98 This progressive deformation shows an asymptotic configuration, being less noticeable at higher loads. This process is repeated continuously under physiological loads (gait), where both the fossa and obturator foramen compress and relax, ultimately allowing for intermittent full contact between the femoral head and the upper part of the acetabulum to occur.97
The horseshoe shape of the acetabular fossa is considered to be advantageous for the load to be distributed more homogeneously. Daniel et al99 were the first to highlight the importance of the acetabular fossa as a means to redistribute the vector force medially, away from the lateral acetabular rim. In their mathematical model, the authors advocate that the shape of the fossa contributes to a uniform load distribution in the hip joint, thus reducing the peak contact forces.99 The authors assumed that the acetabulum was a perfect sphere and that shape of the fossa remained constant among the different anatomical models, although a wide variety of morphological types of both the acetabulum and the fossa had been described.41,100
The acetabular fossa also contributes to the lubrication of the hip joint. Synovial fluid synthesis is mostly done by the peripheral synovium.101 The fossa is the only intra-articular tissue associated with fluid production. Synovial fluid is essential for cartilage nutrition and load transmission. By applying a seal to fluid escape, the labrum reduces maximum contact between the articular surfaces with a uniform distribution of the interstitial synovial fluid.102
Additional functionality to the fossa is provided by the ligamentum teres. This ligament can tolerate up to 204 N before it tears.103 Historically, the ligament was thought to be an important hip stabilizer with the femur in adduction.44 However, further studies suggested it could be an important stabilizer in the setting of hip dysplasia and mostly in the squatting position, in which the iliofemoral ligament is relaxed.104,105
Biological
The pulvinar may have a direct influence on joint homeostasis.106 Adipokines secreted by IAAT (e.g. leptin, adiponectin, resistin, ghrelin) have been shown to have an effect on bone marrow metabolism, central regulation of bone turnover, and even chondral homeostasis.107-110 High doses of leptin can accelerate chondrocyte senescence and further cartilage degeneration.111 Since human chondrocytes express adipokine receptors under physiological conditions, there seems to be a close interaction between such proteins and articular inflammatory diseases.112,113 The inflammatory profile of the IAATs is not only related to the amount of adipokines they can produce, but also to the number of adipocyte-associated and development genes that they express, in charge of activating positive CD45 and tryptase cells.59 However, it remains unclear by which means IAAT secretes adipokines or activates inflammatory cells, and no information exists regarding normal synovial levels of these proteins.
Different osteoarthritic phenotypes have been described as risk factors for degenerative disease, with specific joint/bone shapes being only one such factor.114 Therefore, it should be acknowledged that there is a relatively high percentage of hips with OA but without any morphological or mechanical underlying cause.115 Patients with symptomatic FAI showed increased molecular metabolic activity (interleukin-8 (IL-8), chemokine (C-X-C motif) ligand 3 (CXCL3), CXCL6, CCL3L1, collagen type 2 alpha 1 chain (COL2A1), a disintegrin and metalloproteinase with thrombospondin motifs 4 (ADAMTS-4), and aggrecan (ACAN)) when compared to asymptomatic controls.116 However, the metabolic pattern of cytokine activity has not yet been elucidated in different stages of pre-arthritic joint disease. Since IAAT products may play a key role in maintaining hip joint homeostasis, specific genetic and/or phenotype alterations inducing proinflammatory changes in the IAAT can affect cartilage health independently of age.11 Further molecular studies are necessary to understand the interaction between IAAT and OA.
Section key points
-
The horseshoe shape of the acetabular fossa is considered to be advantageous for the load to be distributed more homogeneously.
-
The pulvinar may have a direct influence on joint homeostasis through IAAT products. Specific genetic and/or phenotype alterations inducing proinflammatory changes in the IAAT can affect cartilage health independently of age.
The acetabular fossa as a decision aid for hip surgery
Hip preservation surgery
The underlying principle for successful joint-preserving surgery is to restore/optimize joint biomechanics so that cartilage is not loaded beyond its failure point. Because there is an intimate relationship between size of the fossa and functional hyaline cartilage,93 understanding the varying morphological fossa characteristics is relevant when considering hip preservation surgery.
Relative size of acetabular fossa
In a detailed 3D study of the acetabular morphology in the painful young adult hip, Steppacher et al117 showed that dysplastic hips had a decreased articular cartilage surface and an increased acetabular fossa surface. Also, the authors reported that in contrast to protrusio hips, retroverted and deep hips did not have an increased size of the acetabular cartilage surface.117 Thus, rim trimming should be performed with caution and a periacetabular osteotomy (PAO) would be the treatment of choice. In line with the above findings, Slullitel et al118 determined the articular cartilage/acetabular fossa (AC/CF) ratio in a subset of hips that underwent hip preservation surgery of various indications. Lower AC/CF ratios were seen in dysplastic hips (0.55 ± 0.047), compared to the pincer (0.68 ± 0.063) and cam groups (0.67 ± 0.061; p < 0.001).
Pun et al39 reviewed 200 hips undergoing PAO, reverse PAO, and open rim trimming and compared the three cohorts with controls in terms of cartilage and fossa widths and heights measured on MRI and CT scans. The authors reported the following mean cartilage/fossa ratios: 0.79 (95% confidence interval (CI) 0.72 to 0.87) for controls, 0.74 (95% CI 0.67 to 0.82) for dysplastic hips, 0.84 (95% CI 0.77 to 0.92) for deep acetabula type-1 (i.e. with increased anterior and posterior cartilage lengths), and 0.49 (95% CI 0.38 to 0.61) for deep acetabula type-2 (i.e. with a larger fossa in height and width and smaller superior cartilage length) (p = 0.001). Compared to control acetabula, dysplastic hips showed proportionally lower cartilage and fossa dimensions, but without differences in the ratio.39 Unlike the study from Slullitel et al,118 dysplasias did not show lower ratios because cartilage and fossa heights and widths were lower in equal proportion (i.e. lower volume overall). Moreover, the authors described two types of deep acetabula: type-1 with increased anterior and posterior cartilage lengths; and type-2 with a larger fossa and small superior cartilage extension.39 Hence, due to its deficient articular cartilage area, this latter group might not benefit from rim trimming and an anteverting PAO might better address the acetabular morphological problem.
Lastly, Trinh et al119 recently performed a 3D-CT polyhedral reconstruction of 60 FAI and dysplasia cases to define a correlation between acetabular cartilage area and lateral centre-edge angle. Instead of calculating cartilage/fossa ratios, the authors measured articular surface area through 3D stereolithographical models. They reported that Wiberg’s angle had a significant but moderate correlation with acetabular cartilage area (R2 = 0.38; p = 0.002, Pearson correlation following a linear regression model), although this correlation was poor in non-dysplastic hips (R2 = 0.02; p = 0.88, Pearson correlation following a linear regression model).119 It is thus evident that until novel radiological parameters are described, the most reliable method to determine the cartilage/fossa ratio or the percentage of articular cartilage within the hip is only from axial imaging.
Ability of the fossa to remodel
The relative size of the acetabular fossa may change following reorientation osteotomy of the hip. Data from Yamasaki et al120 have shown that a significant amount of remodelling can take place within the acetabular fossa. The authors retrospectively compared the clinical and radiological outcomes of 21 rotational acetabular osteotomies (RAOs) that had undergone additional multiple (six to eight) drillings at the acetabular fossa in order to induce expansion of the medial subchondral bone with 14 RAOs without additional drilling.120 The authors noted a significant difference in the extent of the radiological weight-bearing area (from the medial to the lateral sourcil) in the group with adjuvant drilling, as a result of supposed cartilaginous metaplasia or cartilaginous ossification of the acetabular fossa.120 Even though the same authors reported that this medial acetabular enlargement can happen spontaneously following RAO,121 it was markedly increased with the addition of post-RAO arthroscopic-assisted drilling of the fossa.
In order to further elucidate whether the fossa is able to ‘remodel’ following a reorientational osteotomy, Shimogaki et al122 performed an experimental study to detect changes in the acetabular cartilage in 24 rabbit hips, of which 12 underwent RAO and the remaining 12 conservative treatment. At 26 weeks, the nonoperated hips evidenced exfoliation of the articular cartilage, while those subjected to RAO showed cloning and increased uptake of the cartilage matrix without degenerative changes, with additional cartilaginous metaplasia at the acetabular fossa, which became the new weight-bearing area.122 This concept of histological remodelling as an environmental response is not new. In an experimental model, Hiranuma et al123 described cartilaginous metaplasia resembling cartilage in the interposed joint capsule after performing a Chiari osteotomy in 20 rabbits.
Tears of ligamentum teres
Tears of the ligamentum teres are an additional common finding in hip preservation candidates, thought to have a prevalence rate of 51%.124 Hips with structural deficiencies may be at an increased risk of ligamentum teres injuries.125 In open rim trimming and/or femoral osteochondroplasty (surgical hip dislocation), the ligamentum teres is routinely cut without leading to further instability.126 However, in the setting of hip dysplasia or hyperlaxity, the ligamentum teres might have a more important role in hip stabilization and should be preserved or repaired.127 Still, the routine indication of ligamentum teres repair remains controversial.
Hip arthroplasty surgery
The acetabular fossa has historically been useful for locating the centre of rotation when reaming the acetabular bone. The identification of the outer lamina of the fossa is essential to determine the depth of the acetabular component and appropriately reconstruct the hip joint’s centre of rotation (mediolaterally and anteroposteriorly) and offset.128-131 However, some believe that the fossa might not be located at the true centre of rotation, but rather rely anterior to the hip of rotation, and this should be contemplated so as not to over-ream the anterior wall.132
Section key points
-
The relative size of the fossa may help delineate between dysplasia and femoroacetabular impingement in hips with a lateral centre-edge angle between 20° and 25°.
-
Further studies are required to determine whether dysplasia is associated with a reduced cartilage to fossa ratio within the acetabulum.
-
A considerable amount of remodelling (i.e. cartilaginous metaplasia) can occur in the fossa after reorientational osteotomy, where the fossa becomes a weight-bearing area.
-
In THA, the identification of the outer lamina of the fossa is essential to determine acetabular depth for acetabular component implantation; however, the fossa might not be located at the true centre of rotation, but rather rely more anteriorly.
Potential clinical relevance of the acetabular fossa
Given its potential influence on decision-making for both THA and hip preservation surgery, the clinical relevance of the fossa (and cartilage ratio) may become more evident in the near future. Large cohort studies are still necessary to define the cartilage/fossa ratio in dysplastic, overcovered, and retroverted hips as well as ‘normal’ values in asymptomatic controls. Following Stepaccher et al’s117 and Slullitel et al’s studies,118 such ratios can be useful to distinguish between dysplasia and impingement when lateral centre-edge angle (and other common measurements) is measured within ‘normal’ parameters. However, a cip arthrorrelation of cartilage/fossa ratio with easy to measure radiological landmarks still needs to be demonstrated in order to make such measurements/assessments easy to make by all hip preservation surgeons.
Additionally, the identification of the extent of the fossa into the weight-bearing zone can be of value in selecting the best candidate for hip preservation surgery in the setting of dysplasia or FAI.39 In this scenario, some cases of protrusio acetabuli may not be the ideal candidates for rim trimming in the event that a large extension of the fossa determines a small cartilaginous area.93 Equally, hip dysplasia patients with a considerably low cartilage/fossa ratio may not benefit from a reorientational osteotomy if the reoriented fragment (which will become the ‘new’ weight-bearing area) has a large amount of non-cartilaginous tissue (i.e. fatty tissue from the fossa’s pulvinar zone).
Lastly, characterization of the fossa’s biological activity might provide useful diagnostic information (e.g. degree of inflammation and the presence of cellular alterations), acting as novel biomarkers and therapeutic targets for the hip preservation surgery.
Section key points
-
Large cohort studies are still necessary to define the cartilage/fossa ratio in dysplastic, overcovered, and retroverted hips as well as ‘normal’ values in asymptomatic controls.
-
The identification of the extent of the fossa into the weight-bearing zone can become of value to select the best candidate for hip preservation surgery in the setting of dysplasia or FAI.
In summary, several parameters regarding the fossa were explored. This ‘silent’ yet large area within the acetabulum appears to play an important role for the joint’s function, although this is not clearly defined at present. Nevertheless, the fossa is more than a radiological parameter of acetabular depth for acetabular component implantation within THA. The fossa should have an impact on decision-making and surgical outcomes in hip preservation surgery: the analysis of cartilage/fossa ratio may help to distinguish between impingement and dysplasia in so-called borderline hips; it can also aid in patient selection or rejection for specific preservation procedures (e.g. rim trimming for deep hips with low cartilage ratio). As there is a paucity of literature on the potential acetabular fossa changes detected in nonarthritic hip disorders, future studies should explore the fossa’s role in hip preservation surgery not only for its size, shape, and extent, but also for its biological capacity as a source of cytokines, chondrocytes, and stem cells.
References
1. Bozic KJ , Chan V , Valone FH , Feeley BT , Vail TP . Trends in hip arthroscopy utilization in the United States . J Arthroplasty . 2013 ; 28 ( 8 Suppl ): 140 – 143 . Crossref PubMed Google Scholar
2. Palmer AJR , Malak TT , Broomfield J , et al. Past and projected temporal trends in arthroscopic hip surgery in England between 2002 and 2013 . BMJ Open Sport Exerc Med . 2016 ; 2 ( 1 ): e000082 . Crossref PubMed Google Scholar
3. Chen AW , Steffes MJ , Laseter JR , et al. The education and training of future hip preservation surgeons: aggregate recommendations of high-volume surgeons . J Hip Preserv Surg . 2018 ; 5 ( 3 ): 307 – 311 . Crossref PubMed Google Scholar
4. Peters CL , Beaulé PE , Beck M , Tannast M , Jiranek W , Sierra RJ . Report of breakout session: strategies to improve hip preservation training . Clin Orthop Relat Res . 2012 ; 470 ( 12 ): 3467 – 3469 . Crossref PubMed Google Scholar
5. Dick AG , Houghton JM , Bankes MJK . An approach to hip pain in a young adult . BMJ . 2018 : k1086 . Crossref PubMed Google Scholar
6. Beaulé PE , Allen DJ , Clohisy JC , Schoenecker PL , Leunig M . The young adult with hip impingement: deciding on the optimal intervention . Instr Course Lect . 2009 ; 58 : 213 – 222 . PubMed Google Scholar
7. Beaulé PE , Grammatopoulos G , Speirs A , et al. Unravelling the hip pistol grip/cam deformity: origins to joint degeneration . J Orthop Res . 2018 ; 36 ( 12 ): 3125 – 3135 . Crossref PubMed Google Scholar
8. Anwander H , Beck M , Büchler L . Influence of evolution on cam deformity and its impact on biomechanics of the human hip joint . J Orthop Res . 2018. (Epub ahead of print) PMID: 29405367 . Crossref PubMed Google Scholar
9. Wylie JD , Kim Y-J . The natural history of femoroacetabular impingement . J Pediatr Orthop . 2019 ; 39 : S28 – S32 . Crossref PubMed Google Scholar
10. Bhattaram P , Chandrasekharan U . The joint synovium: a critical determinant of articular cartilage fate in inflammatory joint diseases . Semin Cell Dev Biol . 2017 ; 62 : 86 – 93 . Crossref PubMed Google Scholar
11. Woodell-May JE , Sommerfeld SD . Role of inflammation and the immune system in the progression of osteoarthritis . J Orthop Res . 2020 ; 38 ( 2 ): 253 – 257 . Crossref PubMed Google Scholar
12. Leunig M , Beck M , Woo A , Dora C , Kerboull M , Ganz R . Acetabular rim degeneration: a constant finding in the aged hip . Clin Orthop Relat Res . 2003 ; 413 ( 413 ): 201 – 207 . Crossref PubMed Google Scholar
13. Klaue K , Durnin CW , Ganz R . The acetabular rim syndrome. A clinical presentation of dysplasia of the hip . J Bone Joint Surg Br . 1991 ; 73-B ( 3 ): 423 – 429 . Crossref PubMed Google Scholar
14. Domb BG , Ashberg L , Lodhia P , Chandrasekaran S , Suarez-Ahedo C , Domb BG . Relationship between central acetabular osteophytes and femoral head articular damage – a cross-sectional study . J Hip Preserv Surg . 2016 ; 3 ( suppl_1 ). Google Scholar
15. Byrd JWT . Operative hip arthroscopy . New York, NY : Springer , 2013 . Google Scholar
16. Brannon JK . Hip arthroscopy: intra-articular saucerization of the acetabular cotyloid fossa . Orthopedics . 2012 ; 35 ( 2 ): e262-6 . Crossref PubMed Google Scholar
17. Noguchi Y , Miura H , Takasugi S-ichiro , Iwamoto Y . Cartilage and labrum degeneration in the dysplastic hip generally originates in the anterosuperior weight-bearing area: an arthroscopic observation . Arthroscopy . 1999 ; 15 ( 5 ): 496 – 506 . Crossref PubMed Google Scholar
18. Portinaro NMA , Boniforti FG , Gargan MF . Embryology of the human hip . HIP International . 1999 ; 9 ( 1 ): 36 – 40 . Crossref PubMed Google Scholar
19. Strayer LM Jr . Embryology of he human hip joint . Clin Orthop Relat Res . 1971 ; 74: 221 – 240 . PubMed Google Scholar
20. Watanabe RS . Embryology of the human hip . Clin Orthop Relat Res . 1974 ; 98 : 8 – 26 . Crossref PubMed Google Scholar
21. Perumal V , Woodley SJ , Nicholson HD . Ligament of the head of femur: a comprehensive review of its anatomy, embryology, and potential function . Clin Anat . 2016 ; 29 ( 2 ): 247 – 255 . Crossref PubMed Google Scholar
22. Andersen H . Histochemical studies of the development of the human hip joint . Acta Anat . 1962 ; 48 : 258 – 292 . Crossref PubMed Google Scholar
23. Uhthoff HK . [Embryology of the human hip with special reference to the development of the labra] . Z Orthop Ihre Grenzgeb . 1990 ; 128 ( 4 ): 341 – 343 . [in German] Crossref PubMed Google Scholar
24. Iwanaga T , Shikichi M , Kitamura H , Yanase H , Nozawa-Inoue K . Morphology and functional roles of synoviocytes in the joint . Arch Histol Cytol . 2000 ; 63 ( 1 ): 17 – 31 . Crossref PubMed Google Scholar
25. Decker RS , Koyama E , Pacifici M . Articular cartilage: structural and developmental Intricacies and questions . Curr Osteoporos Rep . 2015 ; 13 ( 6 ): 407 – 414 . Crossref PubMed Google Scholar
26. Usami Y , Gunawardena AT , Iwamoto M , Enomoto-Iwamoto M . Wnt signaling in cartilage development and diseases: lessons from animal studies . Lab Invest . 2016 ; 96 ( 2 ): 186 – 196 . Crossref PubMed Google Scholar
27. Wilkinson JM , Zeggini E . The Genetic Epidemiology of Joint Shape and the Development of Osteoarthritis . Calcif Tissue Int . 2020. (Epub ahead of print) PMID: 32393986. Crossref PubMed Google Scholar
28. Verbruggen SW , Nowlan NC . Ontogeny of the human pelvis . Anat Rec . 2017 ; 300 ( 4 ): 643 – 652 . Crossref PubMed Google Scholar
29. Rális Z , McKibbin B . Changes in shape of the human hip joint during its development and their relation to its stability . J Bone Joint Surg Br . 1973 ; 55-B ( 4 ): 780 – 785 . PubMed Google Scholar
30. Delaere O , Dhem A . Prenatal development of the human pelvis and acetabulum . Acta Orthop Belg . 1999 ; 65 ( 3 ): 255 – 260 . PubMed Google Scholar
31. Avisse C , Gomes H , Delvinquiere V , et al. Anatomic study of the pre- and neonatal hip. physiopathologic considerations on dysplasia and congenital dislocation of the hip . Surg Radiol Anat . 1997 ; 19 ( 3 ): 155 – 159 . Crossref PubMed Google Scholar
32. Köhnlein W , Ganz R , Impellizzeri FM , Leunig M . Acetabular morphology: implications for joint-preserving surgery . Clin Orthop Relat Res . 2009 ; 467 ( 3 ): 682 – 691 . Crossref PubMed Google Scholar
33. Maruyama M , Feinberg JR , Capello WN , D’Antonio JA . The Frank Stinchfield Award: morphologic features of the acetabulum and femur: anteversion angle and implant positioning . Clin Orthop Relat Res . 2001 ; 393 : 52 – 65 . PubMed Google Scholar
34. Tannenbaum EP , Zhang P , Maratt JD , et al. A computed tomography study of gender differences in acetabular version and morphology: implications for femoroacetabular impingement . Arthroscopy . 2015 ; 31 ( 7 ): 1247 – 1254 . Crossref PubMed Google Scholar
35. Dudda M , Kim Y-J , Zhang Y , et al. Morphologic differences between the hips of Chinese women and white women: could they account for the ethnic difference in the prevalence of hip osteoarthritis? Arthritis Rheum . 2011 ; 63 ( 10 ): 2992 – 2999 . Crossref PubMed Google Scholar
36. Nakahara I , Takao M , Sakai T , Nishii T , Yoshikawa H , Sugano N . Gender differences in 3D morphology and bony impingement of human hips . J Orthop Res . 2011 ; 29 ( 3 ): 333 – 339 . Crossref PubMed Google Scholar
37. Jaremko JL , Wang CC , Dulai S . Reliability of indices measured on infant hip MRI at time of spica cast application for dysplasia . Hip Int . 2014 ; 24 ( 4 ): 405 – 416 . Crossref PubMed Google Scholar
38. Thorp LE . Hip anatomy. hip Arthrosc . Hip Jt. Preserv. Surg . 2015 : 3 – 15 . Google Scholar
39. Pun SY , Hingsammer A , Millis MB , Kim Y-J . Is increased acetabular cartilage or fossa size associated with pincer femoroacetabular impingement? Clin Orthop Relat Res . 2017 ; 475 ( 4 ): 1013 – 1023 . Crossref PubMed Google Scholar
40. Rissech C , Sañudo JR , Malgosa A . The acetabular point: a morphological and ontogenetic study . J Anat . 2001 ; 198 ( Pt 6 ): 743 – 748 . Crossref PubMed Google Scholar
41. Govsa F , Ozer MA , Ozgur Z . Morphologic features of the acetabulum . Arch Orthop Trauma Surg . 2005 ; 125 ( 7 ): 453 – 461 . Crossref PubMed Google Scholar
42. Lazennec JY , Laudet CG , Guérin-Surville H , Roy-Camille R , Saillant G . Dynamic anatomy of the acetabulum: an experimental approach and surgical implications . Surg Radiol Anat . 1997 ; 19 ( 1 ): 23 – 30 . Crossref PubMed Google Scholar
43. Gupta V , Choudhry R , Tuli A , Choudhry S . Unusual facets on the acetabulum in dry adult human coxal bones a morphological and radiological study . Surg Radiol Anat . 2001 ; 23 ( 4 ): 263 – 267 . Google Scholar
44. Bardakos NV , Villar RN . The ligamentum teres of the adult hip . J Bone Joint Surg Br . 2009 ; 91-B ( 1 ): 8 – 15 . Crossref PubMed Google Scholar
45. Dehao BW , Bing TK , Young JLS . Understanding the ligamentum teres of the hip: a histological study . Acta Ortop Bras . 2015 ; 23 ( 1 ): 29 – 33 . Crossref PubMed Google Scholar
46. Keene GS , Villar RN . Arthroscopic anatomy of the hip: an in vivo study . Arthroscopy . 1994 ; 10 ( 4 ): 392 – 399 . Crossref PubMed Google Scholar
47. Perumal V , Woodley SJ , Nicholson HD . The morphology and morphometry of the fovea capitis femoris . Surg Radiol Anat . 2017 ; 39 ( 7 ): 791 – 798 . Crossref PubMed Google Scholar
48. Fu Z , Peng M , Peng Q . Anatomical study of the synovial plicae of the hip joint . Clin Anat . 1997 ; 10 ( 4 ): 235 – 238 . Crossref PubMed Google Scholar
49. Bencardino JT , Kassarjian A , Vieira RLR , Schwartz R , Mellado JM , Kocher M . Synovial plicae of the hip: evaluation using Mr arthrography in patients with hip pain . Skeletal Radiol . 2011 ; 40 ( 4 ): 415 – 421 . Crossref PubMed Google Scholar
50. Beck M , Leunig M , Ellis T , Sledge JB , Ganz R . The acetabular blood supply: implications for periacetabular osteotomies . Surg Radiol Anat . 2003 ; 25 ( 5-6 ): 361 – 367 . Crossref PubMed Google Scholar
51. Saxler G , Löer F , Skumavc M , Pförtner J , Hanesch U . Localization of SP- and CGRP-immunopositive nerve fibers in the hip joint of patients with painful osteoarthritis and of patients with painless failed total hip arthroplasties . Eur J Pain . 2007 ; 11 ( 1 ): 67 – 67 . Crossref PubMed Google Scholar
52. Sarban S , Baba F , Kocabey Y , Cengiz M , Isikan UE . Free nerve endings and morphological features of the ligamentum capitis femoris in developmental dysplasia of the hip . J Pediatr Orthop B . 2007 ; 16 ( 5 ): 351 – 356 . Crossref PubMed Google Scholar
53. Leunig M , Beck M , Stauffer E , Hertel R , Ganz R . Free nerve endings in the ligamentum capitis femoris . Acta Orthop Scand . 2000 ; 71 ( 5 ): 452 – 454 . Crossref PubMed Google Scholar
54. Buckberry JL , Chamberlain AT . Age estimation from the auricular surface of the ilium: a revised method . Am J Phys Anthropol . 2002 ; 119 ( 3 ): 231 – 239 . Crossref PubMed Google Scholar
55. Rougé-Maillart C , Telmon N , Rissech C , Malgosa A , Rougé D . The determination of male adult age at death by central and posterior Coxal Analysis—A preliminary study . J Forensic Sci . 2004 ; 49 ( 2 ): 1 – 7 . Google Scholar
56. Rissech C , Estabrook GF , Cunha E , Malgosa A . Using the acetabulum to estimate age at death of adult males . J Forensic Sci . 2006 ; 51 ( 2 ): 213 – 229 . Crossref PubMed Google Scholar
57. San-Millán M , Rissech C , Turbón D . Application of the recent SanMillán-Rissech acetabular adult aging method in a North American sample . Int J Legal Med . 2019 ; 133 ( 3 ): 909 – 920 . Crossref PubMed Google Scholar
58. San-Millán M , Rissech C , Turbón D . New approach to age estimation of male and female adult skeletons based on the morphological characteristics of the acetabulum . Int J Legal Med . 2017 ; 131 ( 2 ): 501 – 525 . Crossref PubMed Google Scholar
59. Eymard F , Pigenet A , Citadelle D , et al. Knee and hip intra-articular adipose tissues (IAATs) compared with autologous subcutaneous adipose tissue: a specific phenotype for a central player in osteoarthritis . Ann Rheum Dis . 2017 ; 76 ( 6 ): 1142 – 1148 . Crossref PubMed Google Scholar
60. Garcia J , Wright K , Roberts S , et al. Characterisation of synovial fluid and infrapatellar fat pad derived mesenchymal stromal cells: the influence of tissue source and inflammatory stimulus . Sci Rep . 2016 ; 6 ( 1 ): 24295 . Crossref PubMed Google Scholar
61. Smith M D . The normal synovium . Open Rheumatol J . 2011 ; 5 : 100 – 106 . Crossref PubMed Google Scholar
62. Wilkinson LS , Edwards JC . Microvascular distribution in normal human synovium . J Anat . 1989 ; 167 : 129-36 . PubMed Google Scholar
63. Rodeo SA . Cell therapy in orthopaedics . Bone Joint J . 2019 ; 101-B ( 4 ): 361 – 364 . Crossref PubMed Google Scholar
64. Wilkinson LS , Worrall JG , Sinclair HD , Edwards JC . Immunohistological reassessment of accessory cell populations in normal and diseased human synovium . Br J Rheumatol . 1990 ; 29 ( 4 ): 259 – 263 . Crossref PubMed Google Scholar
65. Biedert RM , Stauffer E , Friederich NF . Occurrence of free nerve endings in the soft tissue of the knee joint. A histologic investigation . Am J Sports Med . 1992 ; 20 ( 4 ): 430 – 433 . Crossref PubMed Google Scholar
66. Gray AJ , Villar RN . The ligamentum teres of the hip: an arthroscopic classification of its pathology . Arthroscopy . 1997 ; 13 ( 5 ): 575 – 578 . Crossref PubMed Google Scholar
67. Blaszkiewicz M , Willows JW , Johnson CP , Townsend KL . The importance of peripheral nerves in adipose tissue for the regulation of energy balance . Biology . 2019 ; 8 ( 1 ): 10 . Crossref PubMed Google Scholar
68. Harasymowicz NS , Clement ND , Azfer A , Burnett R , Salter DM , Simpson AHWR . Regional differences between Perisynovial and infrapatellar adipose tissue depots and their response to class II and class III obesity in patients with osteoarthritis . Arthritis Rheumatol . 2017 ; 69 ( 7 ): 1396 – 1406 . Crossref PubMed Google Scholar
69. Sharma AM , Staels B . Review: Peroxisome proliferator-activated receptor gamma and adipose tissue--understanding obesity-related changes in regulation of lipid and glucose metabolism . J Clin Endocrinol Metab . 2007 ; 92 ( 2 ): 386 – 395 . Crossref PubMed Google Scholar
70. Distel E , Cadoudal T , Durant S , Poignard A , Chevalier X , Benelli C . The infrapatellar fat pad in knee osteoarthritis: an important source of interleukin-6 and its soluble receptor . Arthritis Rheum . 2009 ; 60 ( 11 ): 3374 – 3377 . Crossref PubMed Google Scholar
71. Koh Y-G , Choi Y-J . Infrapatellar fat pad-derived mesenchymal stem cell therapy for knee osteoarthritis . Knee . 2012 ; 19 ( 6 ): 902 – 907 . Crossref PubMed Google Scholar
72. Sakaguchi Y , Sekiya I , Yagishita K , Muneta T . Comparison of human stem cells derived from various mesenchymal tissues: superiority of synovium as a cell source . Arthritis Rheum . 2005 ; 52 ( 8 ): 2521 – 2529 . Crossref PubMed Google Scholar
73. Thier S , Baumann F , Weiss C , Fickert S . Feasibility of arthroscopic autologous chondrocyte implantation in the hip using an injectable hydrogel . Hip Int . 2018 ; 28 ( 4 ): 442 – 449 . Crossref PubMed Google Scholar
74. Bretschneider H , Trattnig S , Landgraeber S , et al. Arthroscopic matrix-associated, injectable autologous chondrocyte transplantation of the hip: significant improvement in patient-related outcome and good transplant quality in MRI assessment . Knee Surg Sports Traumatol Arthrosc . 2020 ; 28 ( 4 ): 1317-1324 . Crossref PubMed Google Scholar
75. Fontana A , Bistolfi A , Crova M , Rosso F , Massazza G . Arthroscopic treatment of hip chondral defects: autologous chondrocyte transplantation versus simple debridement--a pilot study . Arthroscopy . 2012 ; 28 ( 3 ): 322 – 329 . Crossref PubMed Google Scholar
76. Murata Y , Uchida S , Utsunomiya H , et al. Synovial mesenchymal stem cells derived from the Cotyloid fossa synovium have higher self-renewal and differentiation potential than those from the Paralabral synovium in the hip joint . Am J Sports Med . 2018 ; 46 ( 12 ): 2942 – 2953 . Crossref PubMed Google Scholar
77. Hindle P , Khan N , Biant L , Péault B . The infrapatellar fat pad as a source of perivascular stem cells with increased chondrogenic potential for regenerative medicine . Stem Cells Transl Med . 2017 ; 6 ( 1 ): 77 – 87 . Crossref PubMed Google Scholar
78. Liu Y , Buckley CT , Almeida HV , Mulhall KJ , Kelly DJ . Infrapatellar fat pad-derived stem cells maintain their chondrogenic capacity in disease and can be used to engineer cartilaginous grafts of clinically relevant dimensions . Tissue Eng Part A . 2014 ; 20 ( 21-22 ): 3050 – 3062 . Crossref PubMed Google Scholar
79. Ipplito E , Ishii Y , Ponseti IV . Histologic, histochemical, and ultrastructural studies of the hip joint capsule and ligamentum teres in congenital dislocation of the hip . Clin Orthop Relat Res . 1980 ( 146 ): 246 – 258 . PubMed Google Scholar
80. Varich L , Pathria M , Resnick D , et al. Patterns of central acetabular osteophytosis in osteoarthritis of the hip . Invest Radiol . 1993 ; 28 ( 12 ): 1120 – 1127 . Crossref PubMed Google Scholar
81. Kulkarni P , Kõks S , Harsulkar A , Martson A . AB1323 OSTEOPHYTOSIS process suggest involvement of mast cells, revealed by whole transcriptome analysis of osteophytes from knee osteoarthritis patients . Annals of the Rheumatic Diseases . 2019 ; 78 : 1 – 2125 . Google Scholar
82. Haneda M , Rai MF , O'Keefe RJ , Brophy RH , Clohisy JC , Pascual-Garrido C . Inflammatory response of articular cartilage to femoroacetabular impingement in the hip . Am J Sports Med . 2020 ; 48 ( 7 ): 1 – 10 . Crossref PubMed Google Scholar
83. Sampatchalit S , Chen L , Haghighi P , Trudell D , Resnick DL . Changes in the acetabular fossa of the hip: Mr arthrographic findings correlated with anatomic and histologic analysis using cadaveric specimens . AJR Am J Roentgenol . 2009 ; 193 ( 2 ): W127 – W133 . Crossref PubMed Google Scholar
84. Tannast M , Hanke MS , Zheng G , Steppacher SD , Siebenrock KA . What are the radiographic reference values for acetabular under- and overcoverage? Clin Orthop Relat Res . 2015 ; 473 ( 4 ): 1234 – 1246 . Crossref PubMed Google Scholar
85. Diesel CV , Ribeiro TA , Coussirat C , Scheidt RB , Macedo CAS , Galia CR . Coxa profunda in the diagnosis of pincer-type femoroacetabular impingement and its prevalence in asymptomatic subjects . Bone Joint J . 2015 ; 97-B ( 4 ): 478 – 483 . Crossref PubMed Google Scholar
86. Hanke MS , Steppacher SD , Zurmühle CA , Siebenrock KA , Tannast M . Hips with Protrusio Acetabuli are at increased risk for failure after femoroacetabular impingement surgery: a 10-year followup . Clin Orthop Relat Res . 2016 ; 474 ( 10 ): 2168 – 2180 . Crossref PubMed Google Scholar
87. Nepple JJ , Lehmann CL , Ross JR , Schoenecker PL , Clohisy JC . Coxa profunda is not a useful radiographic parameter for diagnosing pincer-type femoroacetabular impingement . J Bone Joint Surg Am . 2013 ; 95-A ( 5 ): 417 – 423 . Google Scholar
88. Morville P . On the anatomy and pathology of the hip-joint . Acta Orthop Scand . 1936 ; 7 ( 2 ): 107 – 142 . Google Scholar
89. Hanke MS , Steppacher SD , Anwander H , Werlen S , Siebenrock KA , Tannast M . What MRI findings predict failure 10 years after surgery for femoroacetabular impingement? Clin Orthop Relat Res . 2017 ; 475 ( 4 ): 1192 – 1207 . Crossref PubMed Google Scholar
90. Madadi F , Yazdanshenas H , Madadi F , Bazargan-Hejazi S . Double acetabular wall--a misleading point for hip arthroplasty: an anatomical, radiological, clinical study . Int Orthop . 2013 ; 37 ( 6 ): 1007 – 1011 . Crossref PubMed Google Scholar
91. Gupta A , Redmond JM , Hammarstedt JE , Stake CE , Liu Y , Domb BG . Arthroscopic decompression of central acetabular impingement with notchplasty . Arthrosc Tech . 2014 ; 3 ( 5 ): e555 – 558 . Crossref PubMed Google Scholar
92. Lodhia P , Gui C , Martin TJ , et al. Arthroscopic central acetabular decompression: clinical outcomes at minimum 2-year follow-up using a matched-pair analysis . Arthroscopy . 2016 ; 32 ( 10 ): 2092 – 2101 . Crossref PubMed Google Scholar
93. Leunig M , Nho SJ , Turchetto L , Ganz R . Protrusio acetabuli: new insights and experience with joint preservation . Clin Orthop Relat Res . 2009 ; 467 ( 9 ): 2241 – 2250 . Crossref PubMed Google Scholar
94. Dunlop CCR , Jones CW , Maffulli N . Protrusio acetabuli . Bull Hosp Jt Dis . 2005 ; 62 ( 3-4 ): 105 – 114 . Crossref PubMed Google Scholar
95. Kubicki SL , Richardson ML , Martin T , Rohren E , Wei W , Amini B . The acetabular fossa hot spot on 18F-FDG PET/CT: epidemiology, natural history, and proposed etiology . Skeletal Radiol . 2015 ; 44 ( 1 ): 107 – 114 . Crossref PubMed Google Scholar
96. Li M , Zhang C , Yang Y . Effects of mechanical forces on osteogenesis and osteoclastogenesis in human periodontal ligament fibroblasts: A systematic review of in vitro studies . Bone Joint Res . 2019 ; 8 ( 1 ): 19 – 31 . Crossref PubMed Google Scholar
97. Greenwald AS , O'Connor JJ . The transmission of load through the human hip joint . J Biomech . 1971 ; 4 ( 6 ): 507 – 528 . Crossref PubMed Google Scholar
98. Vandenbussche E , Massin P , Augereau B , Lavaste F . Cadaver analysis of anterior and posterior acetabular horn displacement . Rev Chir Orthop Reparatrice Appar Mot . 1999 . Google Scholar
99. Daniel M , Iglič A , Kralj-Iglič V . The shape of acetabular cartilage optimizes hip contact stress distribution . J Anat . 2005 ; 207 ( 1 ): 85 – 91 . Crossref PubMed Google Scholar
100. Zhang H , Zhou J , Guan J , Ding H , Wang Z , Dong Q . How to restore rotation center in total hip arthroplasty for developmental dysplasia of the hip by recognizing the pathomorphology of acetabulum and Harris fossa? J Orthop Surg Res . 2019 ; 14 ( 1 ): 339 . Crossref PubMed Google Scholar
101. Field RE , Rajakulendran K . The labro-acetabular complex . J Bone Joint Surg Am . 2011 ; 93-A ( Suppl 2 ): 22 – 27 . Crossref PubMed Google Scholar
102. Ferguson SJ , Bryant JT , Ganz R , Ito K . The acetabular labrum seal: a poroelastic finite element model . Clin Biomech . 2000 ; 15 ( 6 ): 463 – 468 . Crossref PubMed Google Scholar
103. Philippon MJ , Rasmussen MT , Turnbull TL , et al. Structural properties of the native ligamentum teres . Orthop J Sports Med . 2014 ; 2 ( 12 ): 232596711456196 . Crossref PubMed Google Scholar
104. Jo S , Hooke AW , An K-N , Trousdale RT , Sierra RJ . Contribution of the ligamentum teres to hip stability in the presence of an intact capsule: a cadaveric study . Arthroscopy . 2018 ; 34 ( 5 ): 1480 – 1487 . Crossref PubMed Google Scholar
105. Martin HD , Hatem MA , Kivlan BR , Martin RL . Function of the ligamentum teres in limiting hip rotation: a cadaveric study . Arthroscopy . 2014 ; 30 ( 9 ): 1085 – 1091 . Crossref PubMed Google Scholar
106. Labusca L , Zugun-Eloae F . The unexplored role of intra-articular adipose tissue in the homeostasis and pathology of articular joints . Front Vet Sci . 2018 ; 5 : 35 . Crossref PubMed Google Scholar
107. Lecka-Czernik B . Marrow fat metabolism is linked to the systemic energy metabolism . Bone . 2012 ; 50 ( 2 ): 534 – 539 . Crossref PubMed Google Scholar
108. Gandhi R , Takahashi M , Syed K , Davey JR , Mahomed NN . Relationship between body habitus and joint leptin levels in a knee osteoarthritis population . J Orthop Res . 2010 . Crossref PubMed Google Scholar
109. Berry PA , Jones SW , Cicuttini FM , Wluka AE , Maciewicz RA . Temporal relationship between serum adipokines, biomarkers of bone and cartilage turnover, and cartilage volume loss in a population with clinical knee osteoarthritis . Arthritis Rheum . 2011 ; 63 ( 3 ): 700 – 707 . Crossref PubMed Google Scholar
110. Fowler-Brown A , Kim DH , Shi L , et al. The mediating effect of leptin on the relationship between body weight and knee osteoarthritis in older adults . Arthritis Rheumatol . 2015 ; 67 ( 1 ): 169 – 175 . Crossref PubMed Google Scholar
111. Zhao X , Huang P , Li G , Lv Z , Hu G , Xu Q . Activation of the leptin pathway by high expression of the long form of the leptin receptor (Ob-Rb) accelerates chondrocyte senescence in osteoarthritis . Bone Joint Res . 2019 ; 8 ( 9 ): 425 – 436 . Crossref PubMed Google Scholar
112. Conde J , Scotece M , López V , et al. Adiponectin and leptin induce VCAM-1 expression in human and murine chondrocytes . PLoS One . 2012 ; 7 ( 12 ): e52533 . Crossref PubMed Google Scholar
113. Lago R , Gomez R , Otero M , et al. A new player in cartilage homeostasis: adiponectin induces nitric oxide synthase type II and pro-inflammatory cytokines in chondrocytes . Osteoarthritis Cartilage . 2008 ; 16 ( 9 ): 1101 – 1109 . Crossref PubMed Google Scholar
114. Vina ER , Kwoh CK . Epidemiology of osteoarthritis: literature update . Curr Opin Rheumatol . 2018 ; 30 ( 2 ): 160 – 167 . Crossref PubMed Google Scholar
115. Neogi T , Zhang Y . Epidemiology of osteoarthritis . Rheum Dis Clin North Am . 2013 ; 39 ( 1 ): 1 – 19 . Crossref PubMed Google Scholar
116. Hashimoto S , Rai MF , Gill CS , Zhang Z , Sandell LJ , Clohisy JC . Molecular characterization of articular cartilage from young adults with femoroacetabular impingement . J Bone Joint Surg Am . 2013 ; 95-A ( 16 ): 1457 – 1464 . Crossref PubMed Google Scholar
117. Steppacher SD , Lerch TD , Gharanizadeh K , et al. Size and shape of the lunate surface in different types of pincer impingement: theoretical implications for surgical therapy . Osteoarthritis Cartilage . 2014 ; 22 ( 7 ): 951 – 958 . Crossref PubMed Google Scholar
118. Slullitel PAI , Speirs AD , Dobransky J , et al. 2019 . 'Characterising the acetabular articular Cartilage/Cotyloid fossa ratio in the young adult hip: differentiating between dysplasia and impingement' . ISHA Annual Scientific Meeting , Madrid, Spain ; October 17th-19th . Google Scholar
119. Trinh TQ , Leunig M , Larson CM , et al. Lateral Center-Edge angle is not predictive of acetabular articular cartilage surface area: anatomic variation of the lunate fossa . Am J Sports Med . 2020 : 1 – 7 . Crossref PubMed Google Scholar
120. Yamasaki T , Yasunaga Y , Terayama H , Hamaki T , Deie M , Ochi M . Multiple drillings of the acetabular fossa induce early joint remodeling after rotational acetabular osteotomy for hip dysplasia . Arch Orthop Trauma Surg . 2008 ; 128 ( 9 ): 909 – 913 . Crossref PubMed Google Scholar
121. Yasunaga Y , Ikuta Y , Shigenobu T , Nakamura S , Yamamoto S , Nakashiro J . Rotational acetabular osteotomy for hip dysplasia: spontaneous medial enlargement of the acetabulum . Acta Orthop Scand . 2001 ; 72 ( 1 ): 8 – 12 . Crossref PubMed Google Scholar
122. Shimogaki K , Yasunaga Y , Ochi M . A histological study of articular cartilage after rotational acetabular osteotomy for hip dysplasia . J Bone Joint Surg Br . 2005 ; 87-B ( 7 ): 1019 – 1023 . Crossref PubMed Google Scholar
123. Hiranuma S , Higuchi F , Inoue A , Miyazaki M . Changes in the interposed capsule after Chiari osteotomy: an experimental study on rabbits with acetabular dysplasia . J Bone Jt Surg . 1992 ; 74-B ( 3 ): 463 – 467 . Google Scholar
124. Botser IB , Martin DE , Stout CE , Domb BG . Tears of the ligamentum teres: prevalence in hip arthroscopy using 2 classification systems . Am J Sports Med . 2011 ; 39 Suppl: 117S – 125S . Crossref PubMed Google Scholar
125. Martin RL , Martin HD . Function and clinical relevance of the ligamentum teres . Curr Orthop Pract . 2015 ; 26 ( 4 ): 425 – 428 . Google Scholar
126. Phillips AR , Bartlett G , Norton M , Fern D . Hip stability after ligamentum teres resection during surgical dislocation for CAM impingement . Hip Int . 2012 ; 22 ( 3 ): 329 – 334 . Crossref PubMed Google Scholar
127. O’Donnell JM , Devitt BM , Arora M . The role of the ligamentum teres in the adult hip: redundant or relevant? A review . J Hip Preserv Surg . 2018 ; 5 ( 1 ): 15 – 22 . Crossref PubMed Google Scholar
128. Goodman SB , Adler SJ , Fyhrie DP , Schurman DJ . The acetabular teardrop and its relevance to acetabular migration . Clin Orthop Relat Res . 1988 ; 236 : 199 – 204 . PubMed Google Scholar
129. Bonnin MP , Archbold PHA , Basiglini L , Fessy MH , Beverland DE . Do we medialise the hip centre of rotation in total hip arthroplasty? influence of acetabular offset and surgical technique . Hip Int . 2012 ; 22 ( 4 ): 371 – 378 . Crossref PubMed Google Scholar
130. Meermans G , Doorn JV , Kats J-J . Restoration of the centre of rotation in primary total hip arthroplasty . Bone Joint J . 2016 ; 98-B ( 12 ): 1597 – 1603 . Crossref PubMed Google Scholar
131. Terrier A , Levrero Florencio F , Rüdiger HA . Benefit of cup medialization in total hip arthroplasty is associated with femoral anatomy . Clin Orthop Relat Res . 2014 ; 472 ( 10 ): 3159 – 3165 . Crossref PubMed Google Scholar
132. Shimodaira H , Tensho K , Akaoka Y , et al. The acetabular fossa may not be located at the true center of the acetabulum . J Bone Joint Surg Am . 2018 ; 100-A ( 5 ): e27 . Google Scholar
Author contributions
P. A. Slullitel: Contributed to the study concept and design, Acquired and analyzed the data, Drafted the manuscript.
D. Coutu: Contributed to the concept and design of the study, Acquired and analyzed the data, Drafted the manuscript.
M. A. Buttaro: Contributed to the concept and design of the study, Acquired and analyzed the data, Drafted the manuscript.
P. E. Beaule: Contributed to the concept and design of the study, Acquired and analyzed the data, Drafted the manuscript.
G. Grammatopoulos: Contributed to the concept and design of the study, Acquired and analyzed the data, Drafted the manuscript.
Funding statement
No benefits in any form have been received or will be received from a commercial party related directly or indirectly to the subject of this article.
ICMJE COI statement
This review work did not require institutional ethics committee approval and the authors have no conflicts of interest to disclose that are related to the submitted work.
Acknowledgements
We would like to acknowledge Dr. Franco L. De Cicco for collaborating with the illustrations.
Follow P. A. Slullitel @pslull
Follow M. A. Buttaro @drmartinbuttaro
Follow the authors @ortho_uottawa
© 2020 Author(s) et al. This is an open-access article distributed under the terms of the Creative Commons Attribution Non-Commercial No Derivatives (CC BY-NC-ND 4.0) licence, which permits the copying and redistribution of the work only, and provided the original author and source are credited. See https://creativecommons.org/licenses/by-nc-nd/4.0/.