Abstract
Objectives
The purpose of this study was to investigate whether the femoral head–neck contour, characterised by the alpha angle, varies with the stage of physeal maturation using MRI evaluation of an asymptomatic paediatric population.
Methods
Paediatric volunteers with asymptomatic hips were recruited to undergo MRI of both hips. Femoral head physes were graded from 1 (completely open) to 6 (completely fused). The femoral head–neck contour was evaluated using the alpha angle, measured at the 3:00 (anterior) and 1:30 (anterosuperior) positions and correlated with physeal grade, with gender sub-analysis performed.
Results
A total of 43 asymptomatic paediatric volunteers (26 male, 17 female) with mean age 13.0 years (eight to 18) were included with review of bilateral hip MRIs. Correlation between the physeal grade and alpha angle was moderate in males at both the 3:00 (r = 0.477, p < 0.001) and 1:30 (r = 0.509, p < 0.001) positions, whereas there was no significant correlation in females. A significant difference was found between the alpha angles of all the physeal grades (3:00, p = 0.030, 1:30, p = 0.005), but only in males, with the angle increasing with higher grades. For physeal grading, the inter-reader reliability was substantial (intraclass correlation coefficient (ICC) = 0.694), and the intra-reader reliability was also substantial (ICC = 0.788).
Conclusion
The femoral head–neck contour varies and correlates with the stage of physeal development, but only in males, with the alpha angle increasing with progressive physeal maturation. This suggests that gender differences exist in the natural physiological growth, development or remodelling of femoral head–neck junction. In males, pre-physeal fusion may be a critical period of vulnerability for development of morphologic abnormalities of the femoral head–neck junction.
Cite this article: Bone Joint Res 2015;4:17–22
Article focus
To use MRI for evaluating and grading the proximal femoral physis in an asymptomatic paediatric population
To determine whether the femoral head–neck contour, characterised by the alpha angle, correlates with the stage of physeal maturation
To determine if any gender differences exist in the contour of the femoral head–neck junction prior to, and during physeal maturation
Key messages
The femoral head–neck contour does vary as a function of physeal maturation, but only in males, in whom it is positively correlating with the alpha angle.
This suggests that gender differences exist in the natural physiological growth, development or remodelling of femoral head–neck junction.
Pre-physeal fusion may be the critical period of variability in development of the femoral head–neck junction, after which the permanent, final primary morphology is established. This suggests that the cam deformity develops during that time.
Strengths and limitations
MRI provides direct visualisation of the femoral head physis and head–neck contour.
The physis grading scheme has substantial inter- and intra-rater reliability
A prospective, longitudinal study with larger sample size and wider age range would provide further understanding of the development of the femoral head–neck junction.
Introduction
The contour of the femoral head–neck junction is of importance as dysmorphisms, namely the cam deformity, can predispose to femoroacetabular impingement (FAI). The cam deformity is the result of excess osteochondral bulk at the anterosuperior femoral head–neck junction. The presence of a cam deformity results in reduced clearance during range of movement, and subsequent repeated abutment, between the femur and acetabular rim. This leads to hip pain and early labral and cartilage damage, which may predispose to osteoarthritis.1 Because of the relatively high prevalence of the cam deformity in the normal population,2,3 understanding the natural developmental evolution of the head–neck junction, and possibly of the cam deformity, is critical in order to better delineate arthritic disease causation. It has been shown that adult individuals with a cam deformity have an abnormal extension of the femoral epiphysis into the neck postulating a developmental aetiology of the deformity.4 In a paediatric study, the cam deformity was rarely present in children prior to physeal closure, with increased activity level being a risk factor for its development.5 It has also been suggested that an alteration of the growth plate, rather than reactive bone formation, is what produces the cam deformity in athletes.6A recently published study by our group on asymptomatic paediatric volunteers also found a higher prevalence of cam deformity in more skeletally mature subjects compared with younger subjects with open physes.7
However, because of the cross-sectional nature of these studies, it is unclear whether the cam deformity formation is a secondary reaction to physical stress or whether it represents a variation of physeal development. More importantly, it is unknown whether the femoral head–neck contour is predetermined and fixed, or whether it is dynamic and changes over time. Furthermore, it is unclear whether the potential critical dynamic period is during physeal maturation. The purpose of this study was to investigate whether the femoral head–neck contour, characterised by the alpha angle, correlates with the stage of physeal maturation using MRI evaluation of an asymptomatic paediatric population.
Materials and Methods
Ethics approval was obtained from the Institutional Research Ethics Board with informed consent obtained from all subjects. Between July and December of 2010, volunteers were recruited at a single tertiary-level paediatric hospital from an outpatient orthopaedic clinic, being seen for injuries of the upper extremity; all had asymptomatic lower extremities. Exclusion criteria included a history of > 1 previous fracture or vertebral fracture, hip or knee pain, lower extremity pathology or surgery, known congenital or developmental musculoskeletal disorder or any form of arthritis. The current research project is a retrospective study using a subject cohort that compared alpha angle values before and after physeal closure.7
All subjects underwent MRI of bilateral hips on a 1.5T MRI scanner (GE HDxt v15, General Electric Medical Systems, Milwaukee, Wisconsin) with a phased array surface coil placed anteriorly over the pelvis and with spine phased array coils situated posteriorly. Subjects were supine with their feet held in neutral position. The MRI protocol included an axially-acquired, three-dimensional, isotropic, T1-weighted spoiled gradient echo sequence with the following parameters: field of view 40 cm, slice thickness 1 mm, acquisition matrix 384 x 256, TR/TE/flip angle = 11.4 ms/4.0 ms/205°, 1 average. Multiplanar reformation (MPR) was carried out by a senior MRI technologist with 13 years’ experience to generate radial images, using the centre of the femoral neck as the axis of rotation, with images 2 mm thick generated at 15° intervals. Acquisition and MPR images were sent to PACS (PACS System Siemens Syngo Workflow version 30B; Siemens AG, Erlangen, Germany) for review, anonymised with respect to age and gender, with the only identifier being a pre-assigned subject number known only to the research coordinator. Neither sedation nor contrast agent was administered.
To assess the femoral head–neck contour,8,9 the alpha angle of Nötzli10 was used to determine the presence or absence of a cam deformity. Specifically, it measures the degree of femoral deformity, reflecting the insufficient anterosuperior head–neck offset and/or femoral head asphericity.10 The angle was drawn using the previously published technique starting with a best-fit circle drawn around the perimeter of the femoral head.10 The first arm of the angle is the long axis of the femoral neck, defined as the line drawn between the centre of the femoral neck at its narrowest point and the centre of the best-fit circle. The second arm of the angle is drawn from the centre of the best-fit circle anteriorly to the point where the head extends beyond the margin of the circle. Specific images were selected on which to measure the alpha angle. A clock-face nomenclature was adopted for localisation around the femoral head–neck junction, with the anterior and superior locations designated 3 o’clock (3:00) and 12 o’clock (12:00), respectively. Radial MRI images, at the 3:00 (anterior head–neck junction) and the 1:30 (anterosuperior head–neck junction) clock-face positions were used to measure the respective alpha angle by a single reader. Figure 1 provides a diagrammatic representation of the two imaging planes with examples of their respective images generated at each location. Three blinded radiologists, two sub-specialising in musculoskeletal imaging (ten and eight years’ experience) and one in paediatric imaging (eight years’ experience), reviewed all anonymised radial MR images of the hips, assigning a grade to the physes based on their degree of maturity. One musculoskeletal imaging reader performed the grading on two occasions, each six weeks apart. The grading system was adapted from a previously published scheme,11 with physes categorised from 1 (completely unfused) to 6 (completely fused) and is presented in Table I. Open physes are composed of cartilage, which have high water content. As a result they are seen as a broad band of heterogeneous hypointense signals on T1-weighted images. On the other hand, closed physes have undergone endochondral ossification, with replacement of the cartilage resulting in loss of the hypointense band on T1-weighted images. In fused physes, the only visible remnant may be a thin, faintly hypointense line, blending in with the normal cancellous bone marrow space.12 Figure 2 presents examples of all six grades of physeal status.
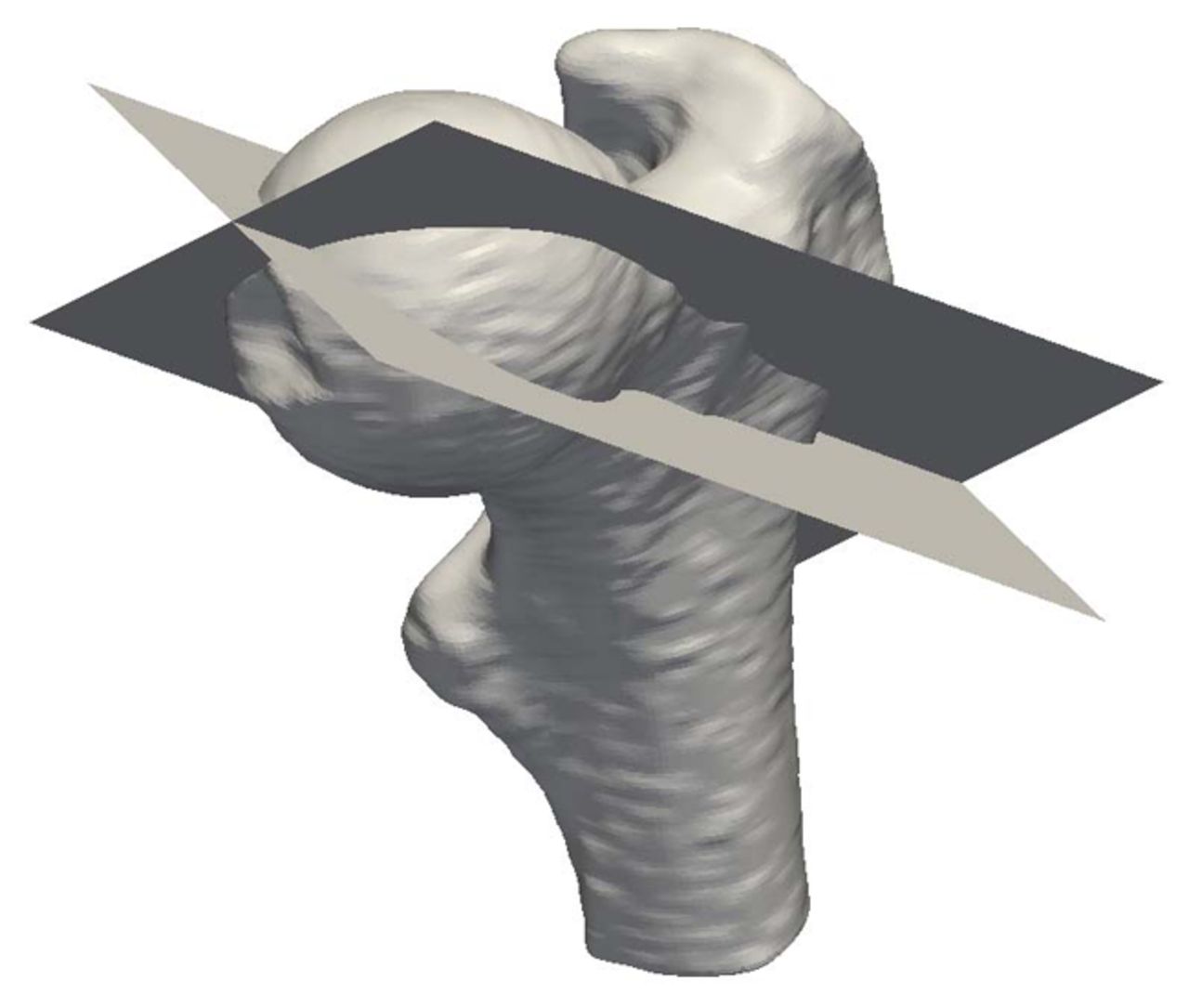
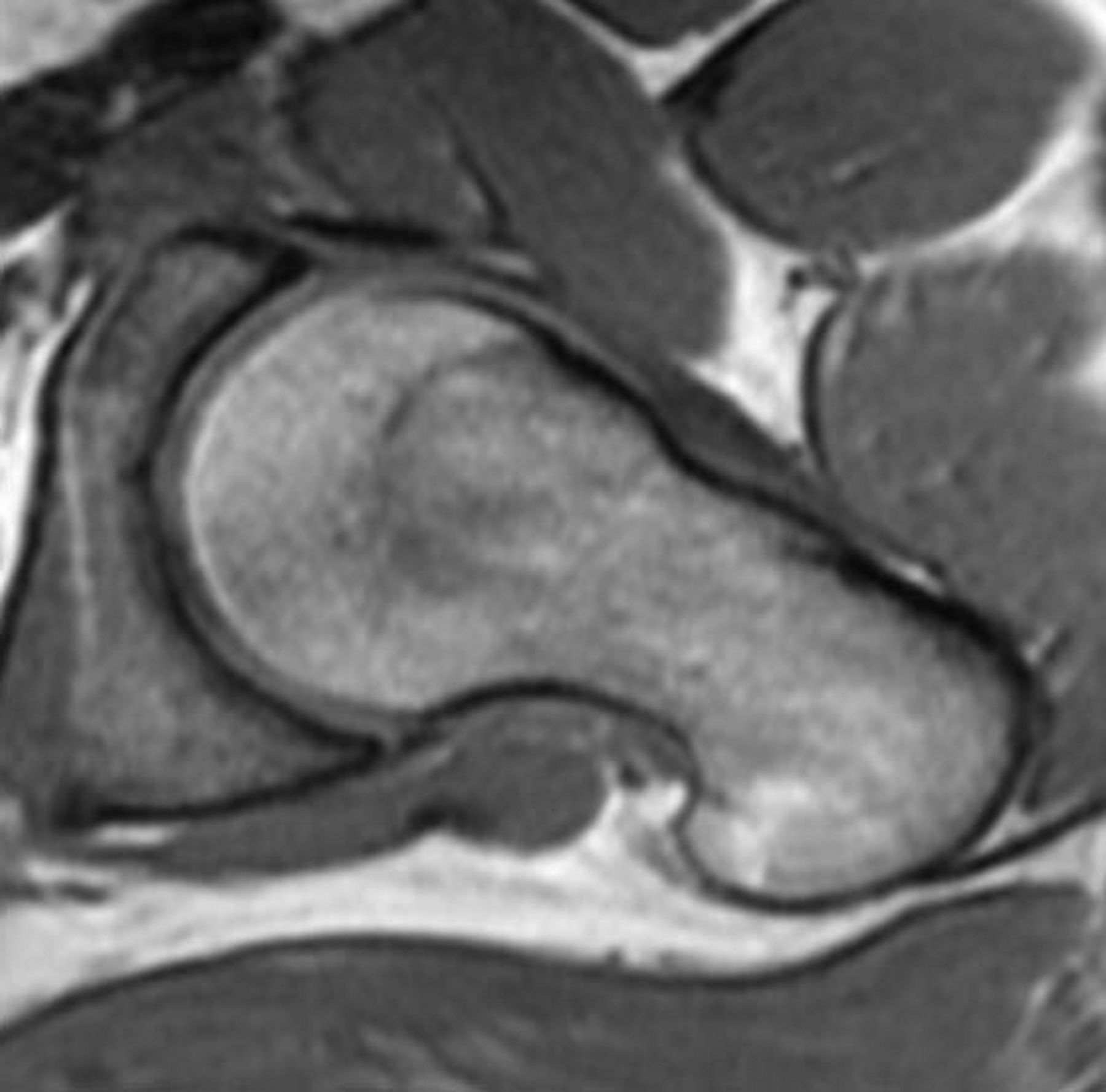
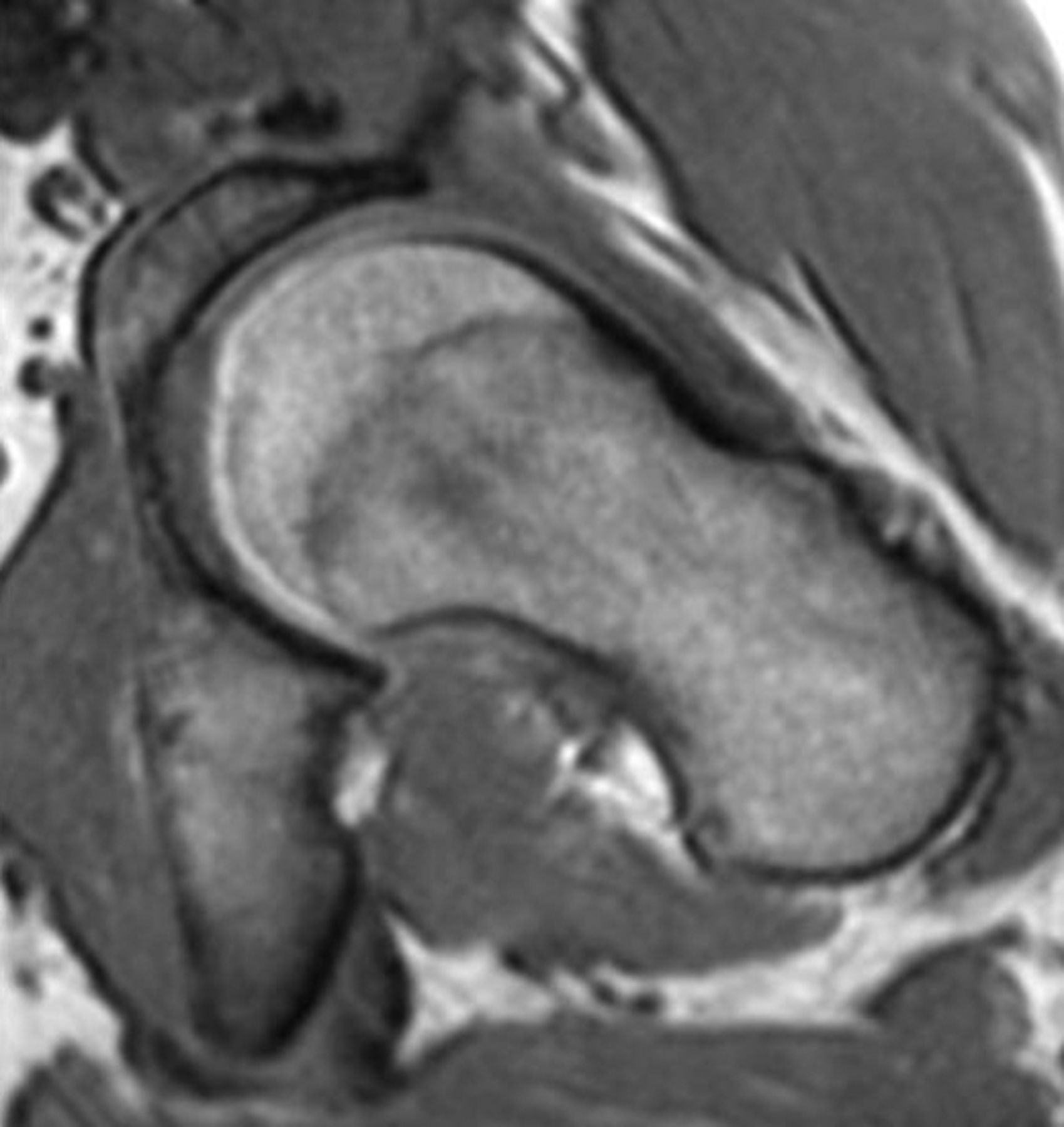
Figs. 1a - 1c
a) A 3D surface rendered image of a proximal femur, with the 3:00 (anterior) and 1:30 (anterosuperior) planes superimposed. MRI images representative of b) the 3:00 and c) 1:30 imaging planes.
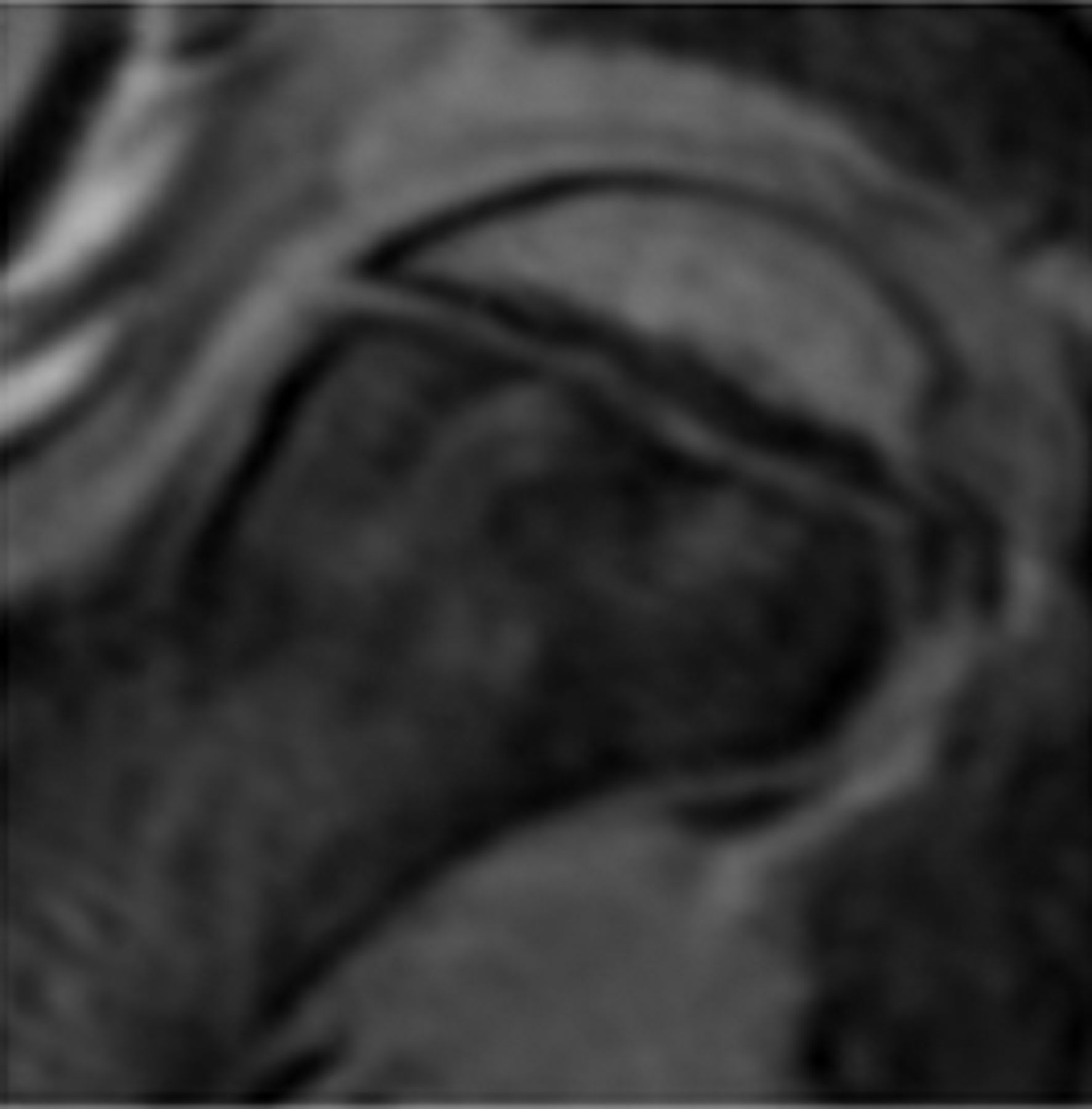
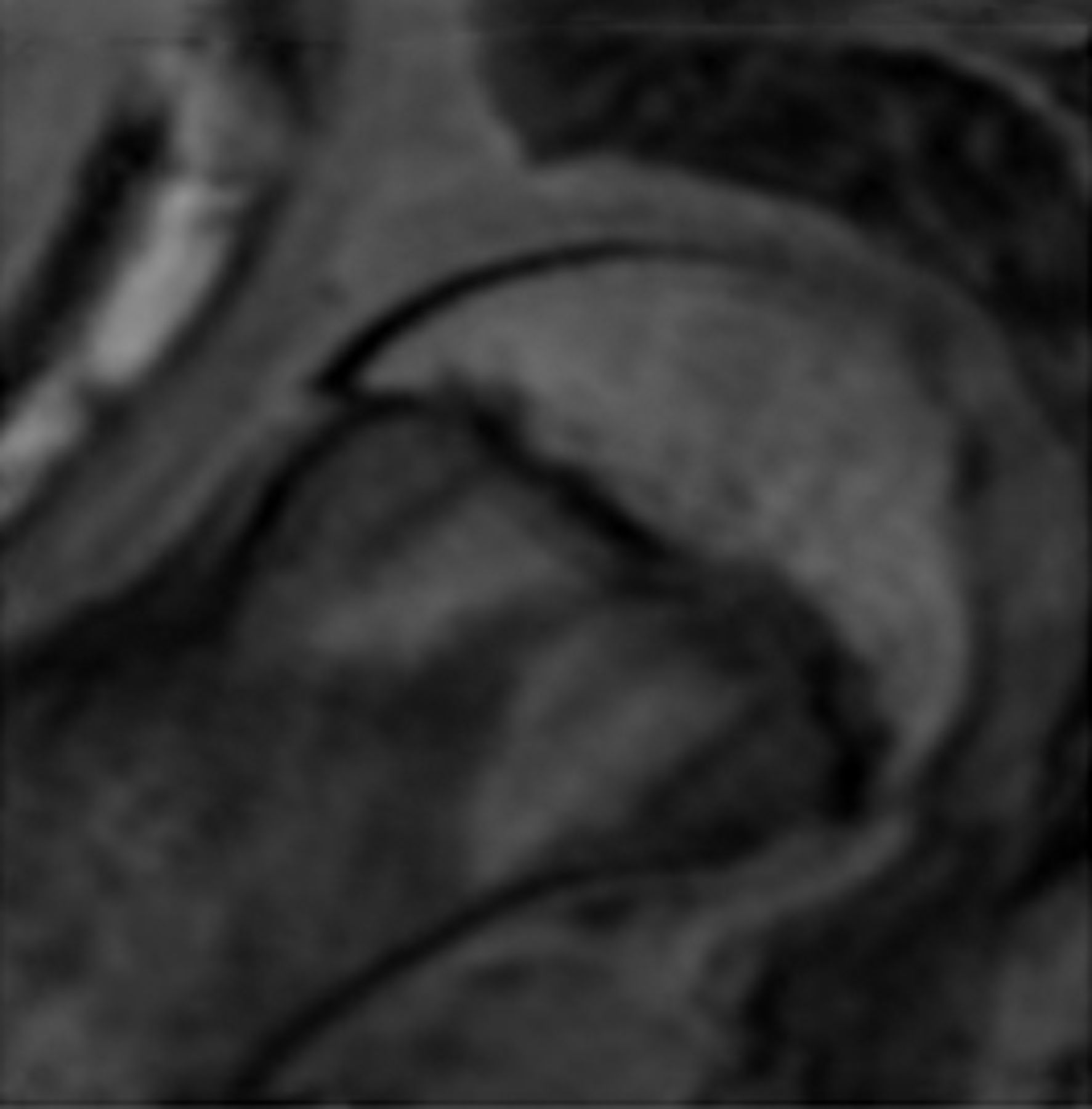
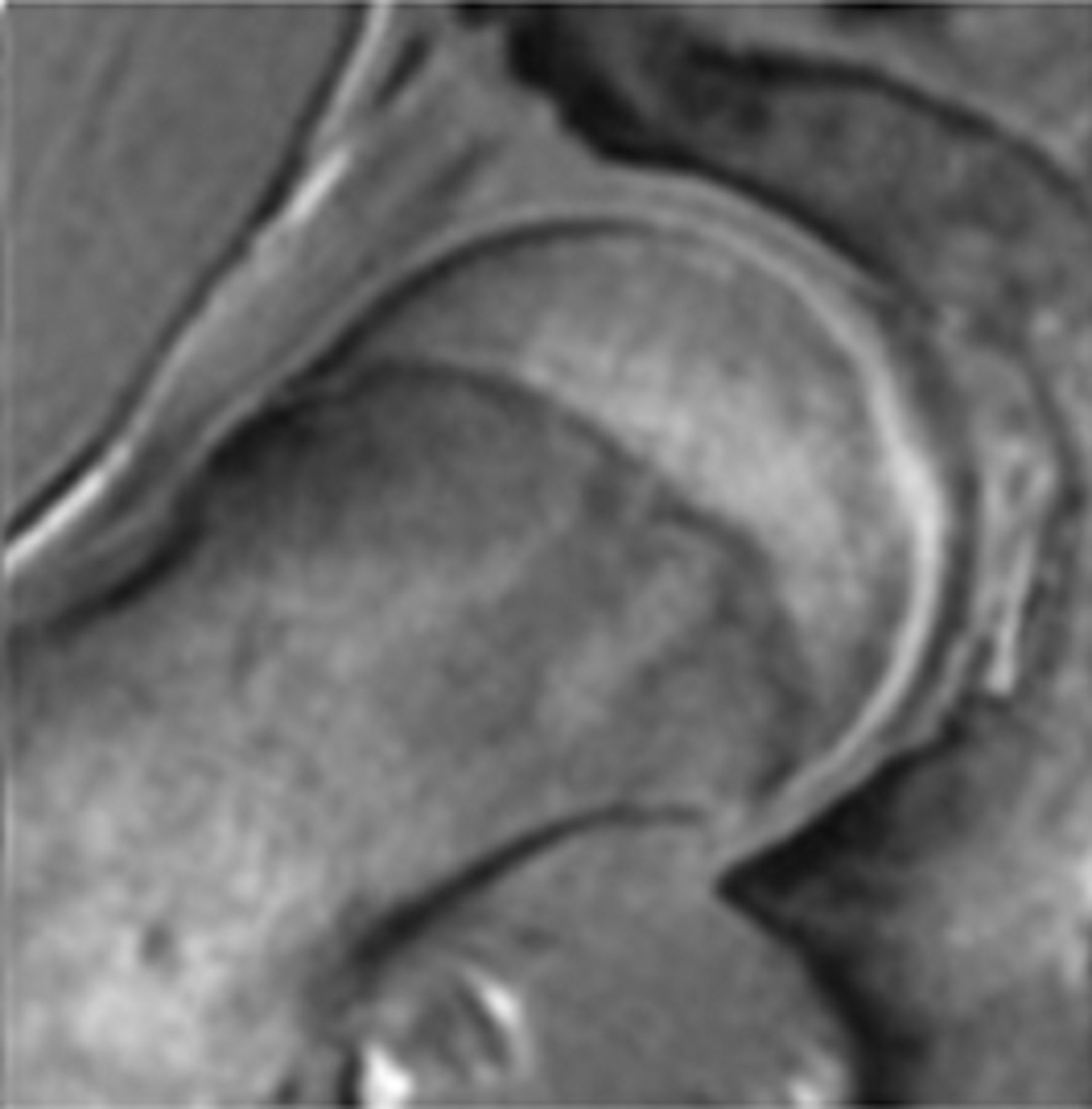
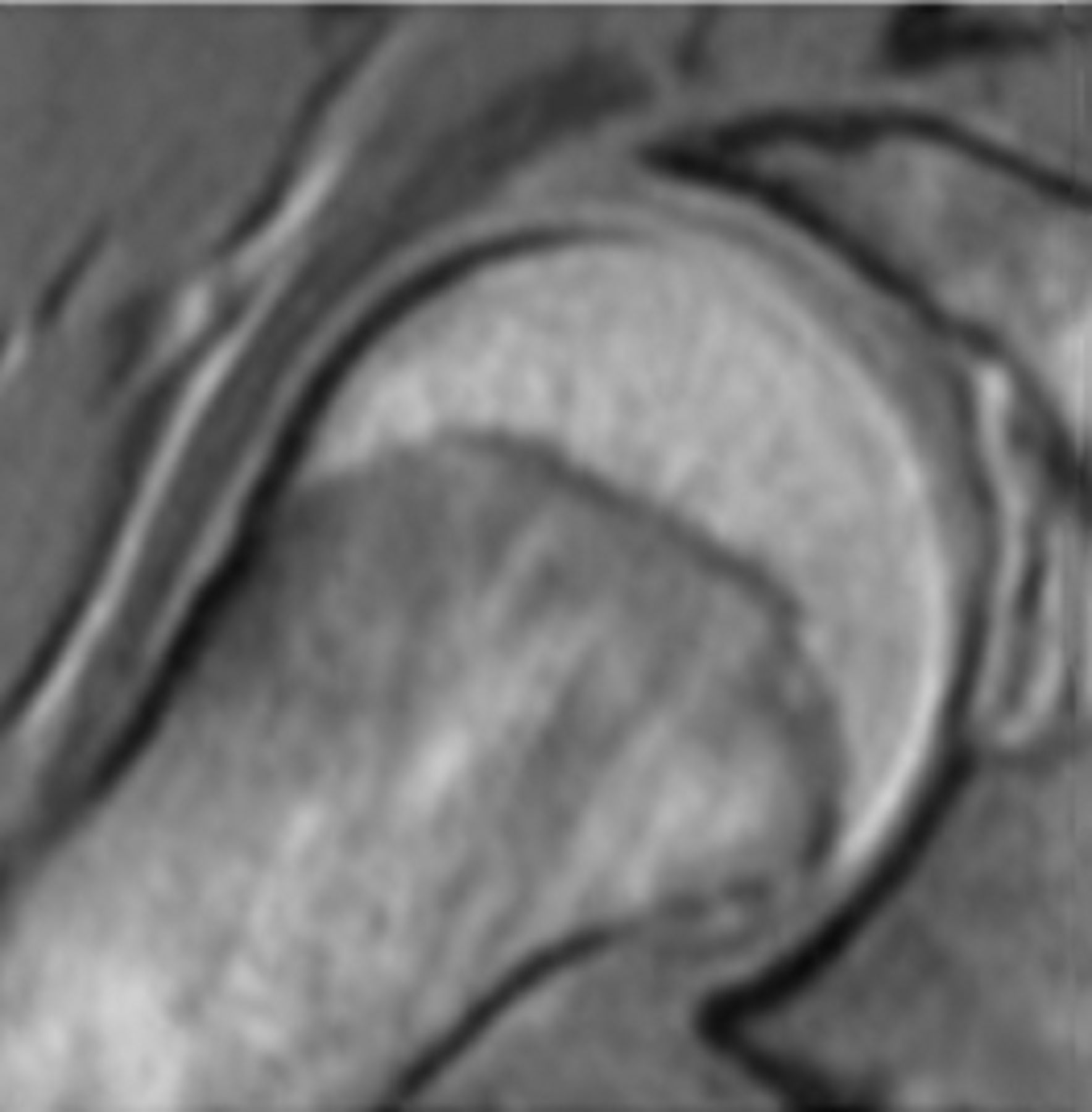
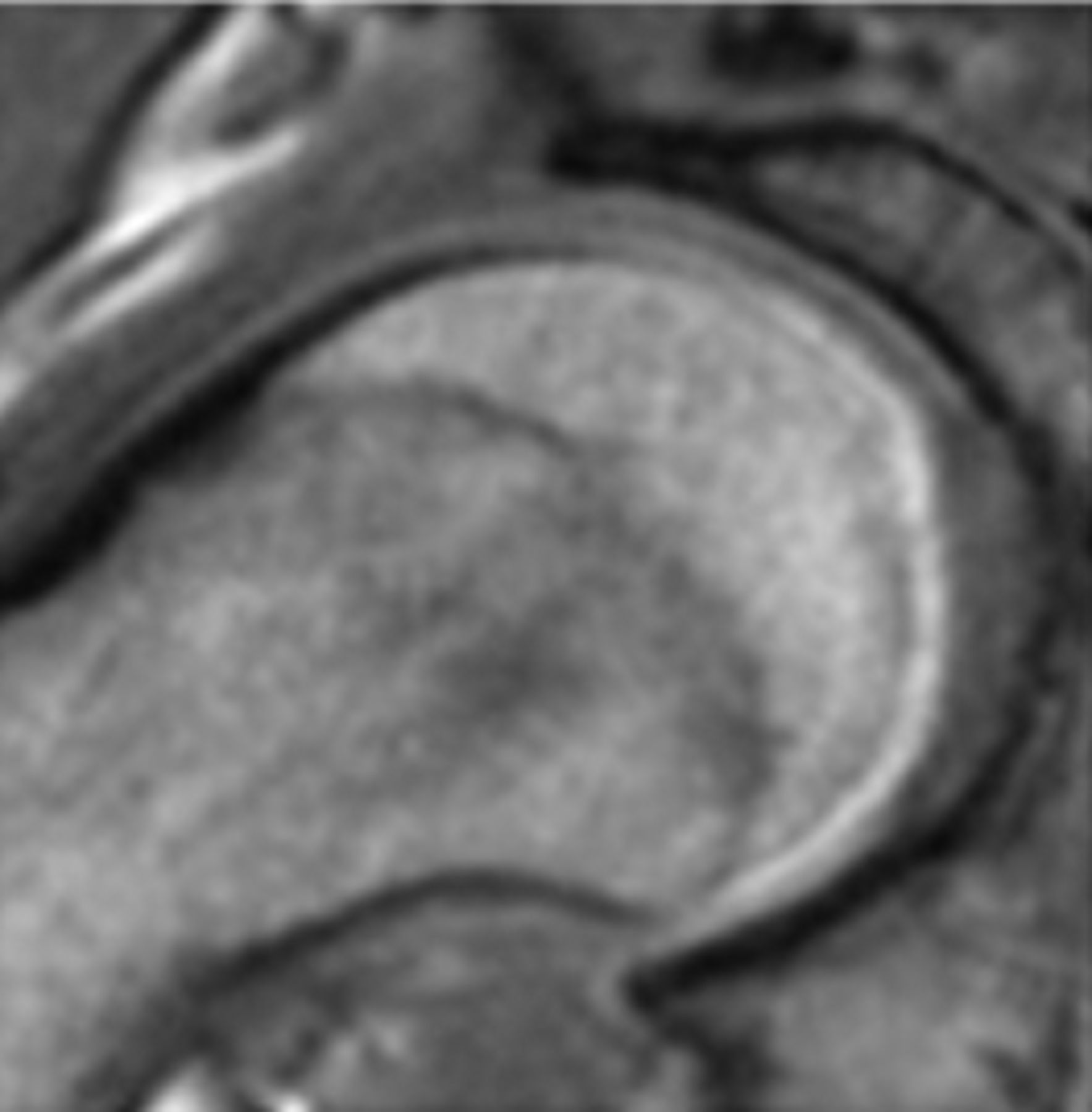
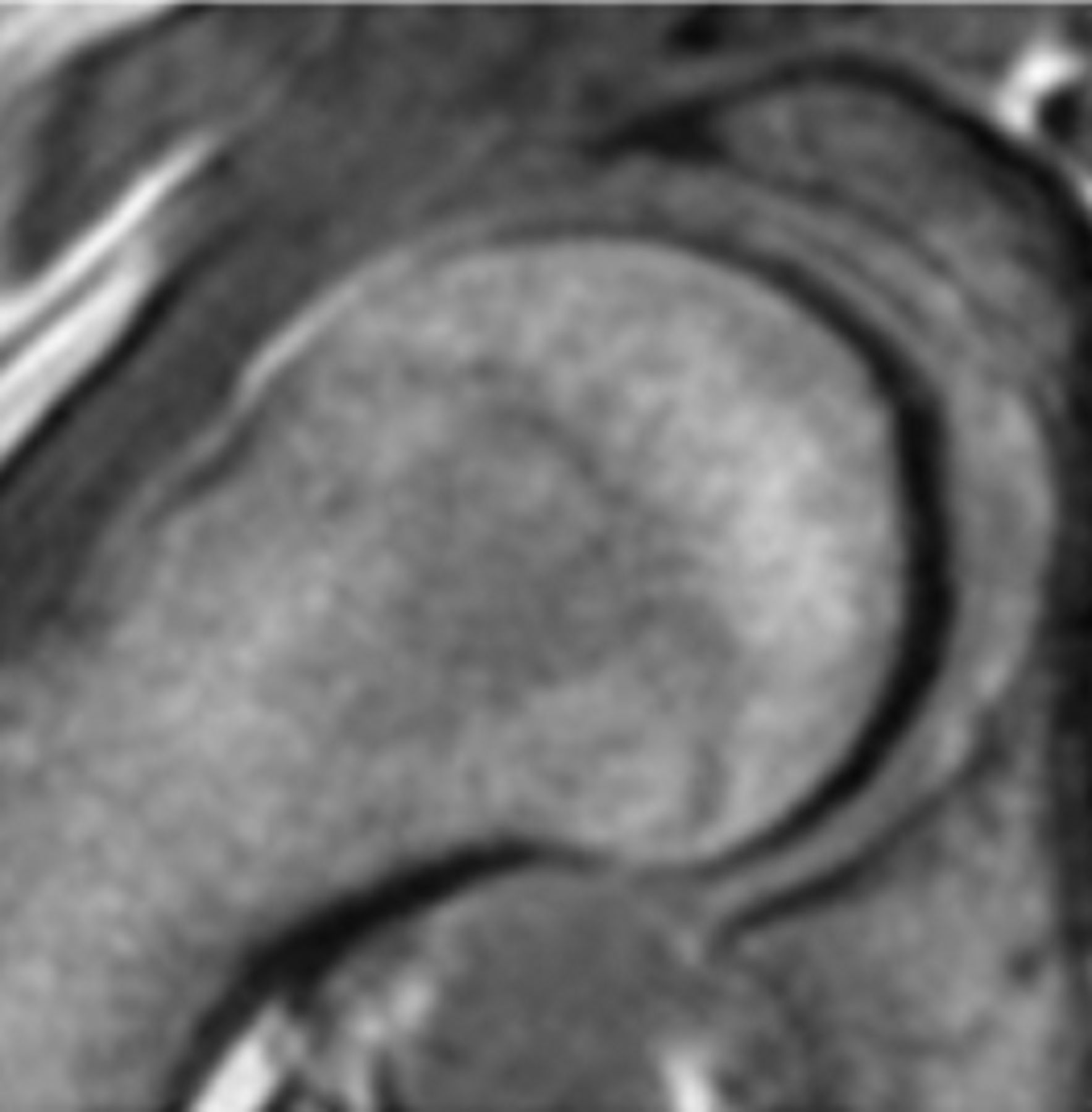
Figs. 2a - 2f
Representative MRI images showing the six physeal grades in study subjects; a) grade 1, b) grade 2, c) grade 3, d) grade 4, e) grade 5, and f) grade 6.
Table I
Table I: MRI grading scheme of femoral head physis
Physeal grade | Fusion status |
---|---|
Grade 1 | Completely unfused (0%) |
Grade 2 | Early fusion (1% to 25%) |
Grade 3 | Moderate fusion (26% to 50%) |
Grade 4 | High fusion (> 50%) |
Grade 5 | Residual unfused physis (< 5 mm open on any slice) |
Grade 6 | Completely fused (100%) |
Statistical analysis
The correlation between the alpha angle and physeal grade and between physeal grade and age for each gender, was determined using Spearman’s rank correlation. The alpha angles (anterior, 3:00 and anterosuperior, 1:30 position values) between the grades were compared for both genders using analysis of variance (ANOVA). Inter- and intra-rater reliabilities were determined using the intraclass correlation coefficient (ICC). All analyses were performed using SPSS software package (version 20; SPSS, Chicago, Illinois), with statistical significance defined as p < 0.05.
Results
Between July and December of 2010, 43 subjects (26 males; mean age 14 years, 10 to 18 and 17 females; mean age 11 years, 8 to 16) were recruited, yielding a total of 86 hips for MRI analysis. Note that one subject from the original study cohort of 44 participants had their MRI data inadvertently deleted from PACS and the backup data were not retrievable.
The physeal grades for all hips were tabulated by gender and presented for a single reader (Table II). For both genders combined, the hips were classified as 23 grade 1, 16 grade 2, nine grade 3, 12 grade 4, 22 grade 5 and four grade 6. Figure 2 presents MRI images of six subjects representative of the varying grades of physeal maturation.
Table II
Femoral head physeal grading of 86 hips, with gender sub-classification
Physeal grade | |||||||
---|---|---|---|---|---|---|---|
1 | 2 | 3 | 4 | 5 | 6 | ||
Gender (n) | |||||||
Male | 9 | 8 | 7 | 8 | 18 | 2 | |
Female | 14 | 8 | 2 | 4 | 4 | 2 | |
Total | 23 | 16 | 9 | 12 | 22 | 4 |
The mean alpha angle values at the 3:00 (anterior) and 1:30 (anterosuperior) positions for both genders, categorised by grade are presented in Table III. Comparing the alpha angle and physeal grade for both genders combined, the correlation was low to moderate at both the 3:00 (r = 0.378, p < 0.001) and 1:30 (r = 0.398, p < 0.001) positions. However, with gender subclassification, the correlation between the physeal grade and alpha angle was stronger in males, being moderate at both the 3:00 (r = 0.477, p < 0.001) and 1:30 (r = 0.509, p < 0.001) positions. In females, there was no significant correlation at the 3:00 (r = 0.052) and 1:30 (r = 0.100) positions. Figure 3 demonstrates scatter plots of the alpha angles (1:30 position) as a function of the physeal grade for male and female patients. Correlation between physeal grade and age was strong in both males (r = 0.794, p < 0.001) and females (r = 0.828, p < 0.001).
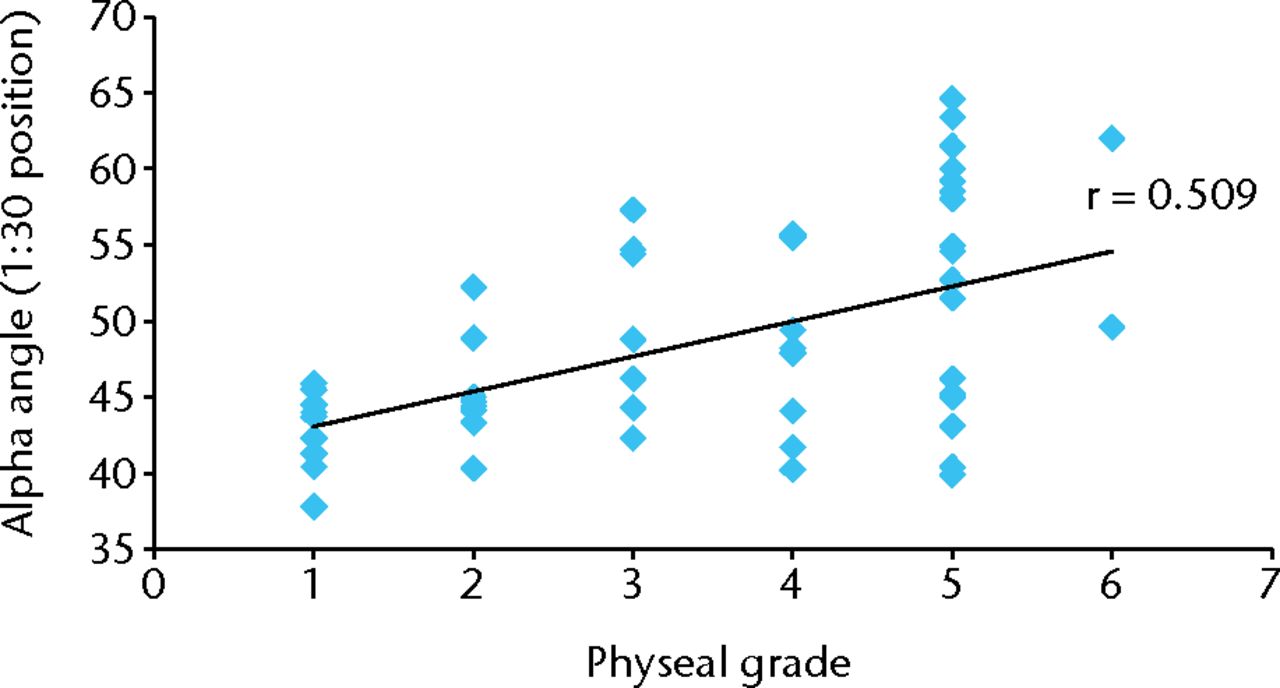
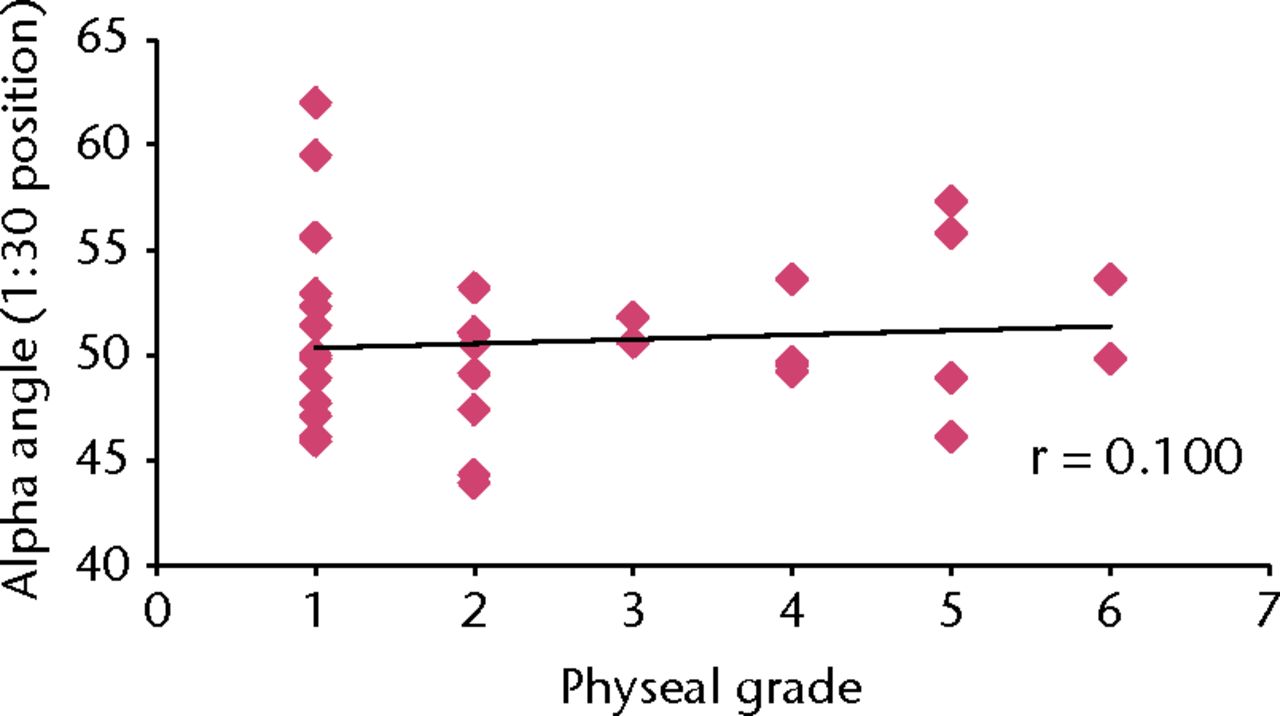
Figs. 3a - 3b
Scatter plot of alpha angle values (1:30 position) versus physeal grade for a) male (r = 0.509) and b) female patients (r = 0.100), with superimposed trend lines (r, Spearman’s rank correlation coefficient.
Table III
Mean alpha angle for each physeal grade, subcategorised by gender and measurement location
Male patients | Female patients | ||||
---|---|---|---|---|---|
1:30 | 3:00 | 1:30 | 3:00 | ||
Grade 1 | 42.82° (37.80 to 45.90) | 37.99° (35.30 to 40.50) | 46.08° (40.90 to 57.00) | 37.64° (32.00 to 48.80) | |
Grade 2 | 45.36° (40.30 to 52.20) | 39.13° (35.40 to 43.40) | 43.81° (38.90 to 48.20) | 37.64° (36.00 to 40.00) | |
Grade 3 | 49.71° (42.30 to 57.30) | 40.31° (34.60 to 45.10) | 46.20° (45.60 to 46.80) | 37.20° (35.50 to 38.90) | |
Grade 4 | 47.84° (40.20 to 55.70) | 42.15° (36.00 to 54.30) | 45.50° (44.20 to 48.60) | 36.78° (32.00 to 40.40) | |
Grade 5 | 52.43° (39.90 to 64.60) | 44.64° (37.90 to 58.30) | 47.03° (41.10 to 52.30) | 39.85° (35.50 to 43.80) | |
Grade 6 | 55.80° (49.60 to 62.00) | 43.35° (41.10 to 45.60) | 46.70° (44.80 to 48.60) | 35.80° (33.10 to 38.50) |
A significant difference was found between the mean alpha angles of the six physeal grades at both the 3:00 (p = 0.028) and 1:30 (p = 0.005) positions, but only in males, with the angle increasing with higher grades. In females there was no significant difference between the alpha angles of the various grades at either the 3:00 (p = 0.840) or 1:30 positions (p = 0.820), with the alpha angles remaining fairly constant across all grades. Representative MRI images of a grade 1 physis (completely open) with a normal alpha angle and a grade 6 physis (closed) with an elevated angle constituting a cam deformity are presented in Figure 4.
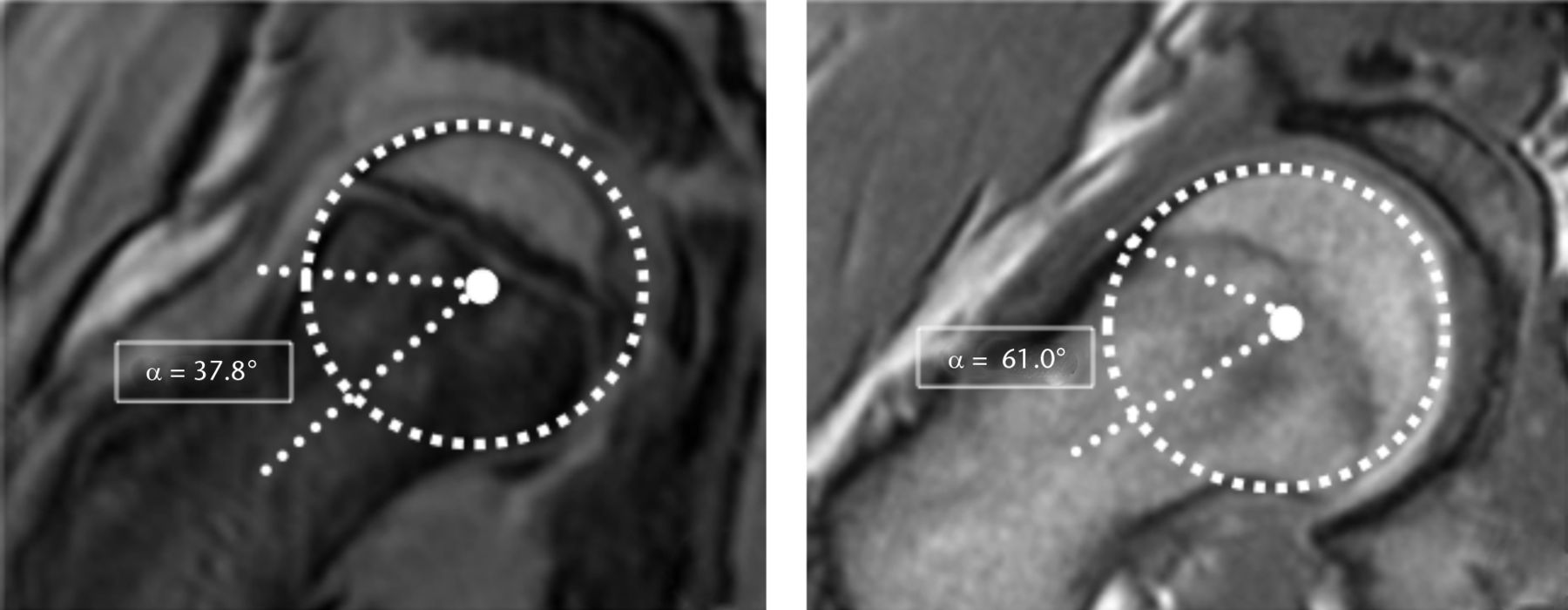
Fig. 4
MRI images at the 1:30 position of a) a ten year old boy with grade 1 physis (completely unfused) with normal alpha angle, 37.8° and b) a16 year old boy with grade 6 physis (completely fused) and elevated alpha angle, 61.0°, constituting a cam deformity.
Both the inter- and intra-reader reliabilities for physeal grading were substantial, with ICC values of 0.694 and 0.788, respectively.
Discussion
Knowledge of the natural development of the femoral head–neck contour is essential in order to understand the evolution of the cam deformity. Given that cam-type FAI is an established cause of hip pain and early osteoarthritis,1 understanding the aetiology of this deformity is important in order to better identify causation. It is unclear whether the deformity is a secondary reaction to physical stress or whether it is a primary lesion related to events occurring during the critical period of physeal development. The stress may be from acute trauma, chronic overloading from sporting activities, infection or metabolic conditions affecting the physical integrity or vascularity of the physis, leading to changes in alignment, growth and remodelling.4,5,7 Given the uncertainty regarding the development of the femoral head–neck junction, whether it is static or dynamic and if gender differences exist, we sought to investigate whether the stage of physeal maturation correlates with the alpha angle using MRI evaluation of an asymptomatic paediatric population.
Moderate correlations between alpha angle and physeal grade were found at the anterosuperior (1:30) and anterior (3:00) positions in males, while only very low correlations were seen in females. Also found only in male subjects, were significantly different mean alpha angles between each grade, with a definite trend of the alpha values increasing with progressive grades. These findings suggest that in males, the femoral head–neck contour is not static – it varies as a function of the stage of physeal maturation, as indicated by progressively increasing alpha angles. This lends support to the theory that the cam deformity may evolve during the dynamic period of physeal maturation and prior to skeletal maturity, based on significantly increased alpha angles seen post physeal closure, specifically in males.6,7,13 Furthermore, this suggests that gender differences exist in the natural physiological growth, development or remodelling of the femoral head–neck junction, with the shape and contour being relatively static in females and dynamic in males over the period that includes puberty. It may be that in females, a different pattern of physeal growth and bone remodelling exists with proportional, symmetric growth of the femoral head and neck, such that the alpha angle does not change as a function of time or physeal grade. In a study evaluating 80 asymptomatic, young, female volunteer hips with MRI, no definite cam-type deformities were identified.14 Collectively, these findings, combined with inherently higher alpha angles in normal males, may account for the higher prevalence of cam deformities and cam-FAI in males.2,3 Activity scores (Habitual Activity Estimation Scale) have been reported on this cohort in another published study, showing that the level of activity was no different between those with open and closed physes. However, those with a cam deformity did have higher HAES scores.7 This suggests that higher activity levels may be associated with, or may be a causative factor for, the development of contour abnormalities at the femoral head–neck junction.
The physeal grading scheme applied in this study was based on the estimated proportion of the cross-sectional area fused as determined on MRI images. This system was able to stratify the subjects across all grades. There was a strong correlation between age and physeal grade for both males and females, as was expected given physeal maturation is known to be gradual and to progress with age. Substantial inter- and intra-rater reliabilities were found for the physeal grading. Collectively, the analyses suggest that the grading system can be applied reliably to the proximal femoral physis and may have future applications in both the clinical and research settings.
This study has limitations. With regards to the subjects, there were unequal numbers of males and females, and they were not age matched. There were also unequal numbers of subjects in each of the grades, with particularly few in grade 6. A larger gender- and age-matched study with larger sample size and wider age range, including older subjects, would allow for more comprehensive investigation. This study was a cross-sectional analysis, as opposed to a prospective longitudinal study that could serially follow subjects from open to closed physis, across all grades. This would allow for the identification of a narrower, critical time period during which the cam deformity begins to evolve, and for potential correlation with any temporally associated physiological, metabolic, or physical activity stressors on the subject at that time. The MRI sequence employed was a T1-weighted protocol as this class of sequence excels when evaluating anatomy and for delineation of osseous margins. A T2-weighted sequence with fat suppression would have had a higher sensitivity to water and made the cartilage containing physes more conspicuous. The MRI sequence was also gradient echo based, which inherently has greater blooming susceptibility artefacts, exaggerating or confounding the boundaries between the cartilaginous and mineralised/ossified zones in the physes and adjacent cancellous bone, respectively. Fast spin echo based sequences would have less of this susceptibility artefact, although they have significantly longer scan times in order to achieve the same quality of scan, hence increasing the chance of movement artefact from subject restlessness. Collectively, these additional sequences when combined with the T1-weighted imaging performed in the current study, may lead to more accurate visualisation and assessment of the physes.
In conclusion, the results of this study demonstrate that the femoral head–neck contour, characterised by the alpha angle, does vary as a function of physeal maturation, but only in males. This suggests that gender differences exist in the natural physiological growth, development or remodelling of the femoral head–neck junction. Furthermore, the shape of the head–neck junction is dynamic in males, with an ability, or susceptibility perhaps, to have variable growth and remodelling patterns prior to skeletal maturity. Pre-physeal fusion may be the critical period of variability in development of the femoral head–neck junction, after which the permanent, final primary morphology is established. This dynamic period may also potentially be a time of vulnerability whereby the proximal femur may be at risk of insult leading to development of cam morphology. There may be yet undetermined static genetic and gender specific, and/or additive dynamic biomechanical, physiological and lifestyle factors, which exert influence on the growth and final shape of the bone. Thus, the time between the commencement of proximal femoral physis maturation and fusion, should be the period that future human clinical, and possibly animal, studies should focus on in order to elucidate the risk factors and pathophysiology of cam deformity development.
1 Ganz R , ParviziJ, BeckM, et al.Femoroacetabular impingement: a cause for osteoarthritis of the hip. Clin Orthop Relat Res2003;417:112–120.CrossrefPubMed Google Scholar
2 Hack K , Di PrimioG, RakhraK, BeauléPE. Prevalence of cam-type femoroacetabular impingement morphology in asymptomatic volunteers. J Bone Joint Surg [Am]2010;92-A:2436–2444.CrossrefPubMed Google Scholar
3 Gosvig KK , JacobsenS, Sonne-HolmS, GebuhrP. The prevalence of cam-type deformity of the hip joint: a survey of 4151 subjects of the Copenhagen Osteoarthritis Study. Acta Radiol2008;49:436–441.CrossrefPubMed Google Scholar
4 Siebenrock KA , WahabKH, WerlenS, et al.Abnormal extension of the femoral head epiphysis as a cause of cam impingement. Clin Orthop Relat Res2004;418:54–60.CrossrefPubMed Google Scholar
5 Siebenrock KA , FernerF, NoblePC, et al.The cam-type deformity of the proximal femur arises in childhood in response to vigorous sporting activity. Clin Orthop Relat Res2011;469:3229–3240.CrossrefPubMed Google Scholar
6 Siebenrock KA , BehningA, MamischTC, SchwabJM. Growth plate alteration precedes cam-type deformity in elite basketball players. Clin Orthop Relat Res2013;471:1084–1091.CrossrefPubMed Google Scholar
7 Carsen S , MorozPJ, RakhraK, et al.The Otto Aufranc Award. On the etiology of the cam deformity: a cross-sectional pediatric MRI study. Clin Orthop Relat Res2014;472:430–436.CrossrefPubMed Google Scholar
8 Beaulé PE , ZaragozaE, MotamediK, CopelanN, DoreyFJ. Three-dimensional computed tomography of the hip in the assessment of femoroacetabular impingement. J Orthop Res2005;23:1286–1292.CrossrefPubMed Google Scholar
9 Beck M , KalhorM, LeunigM, GanzR. Hip morphology influences the pattern of damage to the acetabular cartilage: femoroacetabular impingement as a cause of early osteoarthritis of the hip. J Bone Joint Surg [Br]2005;87-B:1012–1018.CrossrefPubMed Google Scholar
10 Nötzli HP , WyssTF, StoecklinCH, et al.The contour of the femoral head-neck junction as a predictor for the risk of anterior impingement. J Bone Joint Surg [Br]2002;84-B:556–560.CrossrefPubMed Google Scholar
11 Dvorak J , GeorgeJ, JungeA, HodlerJ. Age determination by magnetic resonance imaging of the wrist in adolescent male football players. Br J Sports Med2007;41:45–52.CrossrefPubMed Google Scholar
12 Thapa MM , IyerRS, KhannaPC, ChewFS. MRI of pediatric patients: Part 1, normal and abnormal cartilage. AJR Am J Roentgenol2012;198:W450–W455.CrossrefPubMed Google Scholar
13 Siebenrock KA , KaschkaI, FrauchigerL, WerlenS, SchwabJM. Prevalence of cam-type deformity and hip pain in elite ice hockey players before and after the end of growth. Am J Sports Med2013;41:2308–2313.CrossrefPubMed Google Scholar
14 Leunig M , JüniP, WerlenS, et al.Prevalence of cam and pincer-type deformities on hip MRI in an asymptomatic young Swiss female population: a cross-sectional study. Osteoarthritis Cartilage2013;21:544–550.CrossrefPubMed Google Scholar
Funding statement:
The project was funded internally by CHEO Bone Health and Research Group, TOH Department of Surgery Research Fund, and The Discovery Fund.
Author contributions:
A. Vo: Manuscript writing, Study design, Data collection, Data analysis, Statistical input.
P. Beaule: Manuscript writing, Study design, Data analysis.
M. Sampaio: Manuscript review, Data collection, MRI interpretation.
C. Rotaru: Manuscript review, Data collection, MRI interpretation.
K. Rakhra: Senior and corresponding author, Manuscript writing, Study design, Data analysis, Statistical input, MRI interpretation.
ICMJE Conflict of Interest:
None declared
©2015 The British Editorial Society of Bone & Joint Surgery. This is an open-access article distributed under the terms of the Creative Commons Attributions licence, which permits unrestricted use, distribution, and reproduction in any medium, but not for commercial gain, provided the original author and source are credited.