Abstract
Aims
Trained immunity confers non-specific protection against various types of infectious diseases, including bone and joint infection. Platelets are active participants in the immune response to pathogens and foreign substances, but their role in trained immunity remains elusive.
Methods
We first trained the innate immune system of C57BL/6 mice via intravenous injection of two toll-like receptor agonists (zymosan and lipopolysaccharide). Two, four, and eight weeks later, we isolated platelets from immunity-trained and control mice, and then assessed whether immunity training altered platelet releasate. To better understand the role of immunity-trained platelets in bone and joint infection development, we transfused platelets from immunity-trained mice into naïve mice, and then challenged the recipient mice with Staphylococcus aureus or Escherichia coli.
Results
After immunity training, the levels of pro-inflammatory cytokines (tumour necrosis factor alpha (TNF-α), interleukin (IL)-17A) and chemokines (CCL5, CXCL4, CXCL5, CXCL7, CXCL12) increased significantly in platelet releasate, while the levels of anti-inflammatory cytokines (IL-4, IL-13) decreased. Other platelet-secreted factors (e.g. platelet-derived growth factor (PDGF)-AA, PDGF-AB, PDGF-BB, cathepsin D, serotonin, and histamine) were statistically indistinguishable between the two groups. Transfusion of platelets from trained mice into naïve mice reduced infection risk and bacterial burden after local or systemic challenge with either S. aureus or E. coli.
Conclusion
Immunity training altered platelet releasate by increasing the levels of inflammatory cytokines/chemokines and decreasing the levels of anti-inflammatory cytokines. Transfusion of platelets from immunity-trained mice conferred protection against bone and joint infection, suggesting that alteration of platelet releasate might be an important mechanism underlying trained immunity and may have clinical implications.
Cite this article: Bone Joint Res 2022;11(2):73–81.
Article focus
-
Does immunity training alter platelet releasate at different timepoints (two, four, and eight weeks) after training?
-
Does transfusion of platelets from immunity-trained mice confer protection against bone and joint infection in a naïve mouse?
Key messages
-
Immunity training increases inflammatory cytokines/chemokines and suppresses anti-inflammatory cytokines carried by platelets.
-
Transfusion of platelets from immunity-trained mice confers significant protection against bone and joint infection in a naïve mouse.
Strengths and limitations
-
The present study was the first to demonstrate that immunity training alters platelet releasate. Up to eight weeks after immunity training with two toll-like receptor agonists, platelets are polarized towards a pro-inflammatory phenotype. Transfusion of ‘trained platelets’ into naïve mice reduces the risk of bone and joint infection after bacterial challenge, suggesting that alteration of platelet releasate could be an important mechanism for trained immunity.
-
The main limitation of this study was that we used only representative pathogens (S. aureus and E. coli) and training substances (zymosan and lipopolysaccharide) to train the immune system of model mice. Thus, our results might be different from those obtained with other pathogens and training substances.
Introduction
Bone and joint infection is a devastating disease in orthopaedics, leads to severe health problems, and is considered one of the main causes of disability or even death. Moreover, this type of infection has a high recurrence rate and is difficult to treat, as most antibiotics struggle to penetrate the hard bone to reach the infected area, and some bacteria can escape the host’s immune system and antibiotics by remaining dormant in host cells, replicating intracellularly, and breaking out when the host’s immune system is compromised.1-3
The innate immune response is the cornerstone of host immunity during the acute phase of a response following exposure to pathogens.4 Immediately after microbial invasion, the innate immune system is activated in a conservative and non-specific fashion.5 For these reasons, the innate immune system, rather than the adaptive immune system, plays a more crucial role in preventing infections like bone and joint infection.6 One patient population at increased risk of postoperative bone and joint infection consists of those with compromised innate immunity.7,8 Boosting the innate immunity of this patient group before orthopaedic surgery might be valuable in clinical practice.
A newly emerging concept of innate immune memory, termed ‘trained immunity’ and defined as the ability of the innate immune system to form immune memory and provide long-lasting protection against foreign invaders, was recently discovered in plants, invertebrates, and mammals.9-11 Immunity training with immunologically active substances, such as heat-killed Candida albicans, β-glucans, zymosan, and Bacillus Calmette-Guérin vaccine, enhances the ability of the innate immune system to respond more effectively to future challenges by unrelated microbes.12,13 Recently, researchers in the field of musculoskeletal infection have demonstrated that immunity training can effectively reduce periprosthetic infection in a mouse model.14 To the best of current knowledge, trained immunity involves functional alterations of immune cells, including monocytes, natural killer (NK) cells, and neutrophils.15-17 Unlike adaptive immunity, trained immunity is mainly driven by epigenetic reprogramming downstream of Toll-like receptor (TLR) signaling.10,18
One group of blood cells that expresses various types of functional TLRs is platelets, and megakaryocytes, their precursor cells, which express TLR2 and TLR4.19,20 Thus, it is conceivable that platelets and megakaryocytes could be affected during immunity training. To the best of our knowledge, however, no previous study has evaluated the role of platelets in trained immunity. Platelets have been shown to modulate immune responses during inflammation and infection by megakaryocytic release of various ‘pre-packaged’ bioactive substances.21,22 Releasable substances carried by platelets are stored in three distinct types of intracellular granules: α-granules (chemokines, cytokines, growth factors); δ-granules (small molecules including serotonin, glutamate, histamine); and lysosomes (enzymes for extracellular matrix degradation).23-25 Platelets may play an especially important role in surgical infections, because they are abundant in surgical wounds and are activated.26 In the present study, we tested the hypothesis that immune training changes levels of releasable factors from platelets, and investigated whether transfusion of isolated platelets from immunity-trained mice into naïve mice should confer protection against bacterial challenge.
Methods
All animal experiments were conducted in accordance with protocols approved by the Institutional Animal Care and Use Committee (IACUC) of our hospital, and adhered to China’s regulations on experimental animal usage, which were consistent with Animal Research: Reporting of In Vivo Experiments (ARRIVE) guidelines.
Animals, housing conditions, and immunity training
Female C57BL/6 mice were housed in a controlled environment (20°C to 23°C, 50% of humidity, 12-hour/12-hour light-dark cycle) and in specific pathogen-free conditions. Mice had ad libitum food and tap water and were allowed to acclimate to the laboratory conditions for at least one week before testing. All operations were performed under sterile conditions. At ten weeks of age, the mice were intravenously injected with 0.1 mg of zymosan (TLR2 agonist) and 0.1 mg of lipopolysaccharide (TLR4 agonist) for immunity training according to a previously reported protocol.14 Control mice received normal saline.
Preparation of platelets and haematological analysis
Using a standardized protocol,27 platelets were isolated from control mice (no immunity training, indicated as 0 weeks) and mice euthanized at either two, four, or eight weeks after immunity training. In order to inhibit the activation of platelets and the coagulation cascade, blood was drawn from the inferior vena cava into syringes containing 10% (by volume) citrate-dextrose anticoagulant (Sigma-Aldrich, USA). The blood sample was first centrifuged at 100× g for six minutes to collect upper layer and buffy coat. Platelets were washed twice with modified Tyrode-HEPES buffer (134 mM NaCl, 2.9 mM KCl, 0.34 mM Na2HPO4, 12 mM NaHCO3, 20 mM HEPES, 1 mM MgCl2, 5 mM D-glucose, pH 7.4), and then centrifuged at 800× g for ten minutes. The platelet pellet was resuspended to a desired concentration in Tyrode-HEPES buffer containing prostacyclin (0.5 μM) and apyrase (0.02 U/ml), and the mixture was maintained at 37°C for 30 minutes before using in experiments. Platelet and white cell counts were performed using an automated analyzer (Beckman Coulter Diagnostics, USA).
Preparation of platelet releasate after in vitro activation
For analyses of chemokines and cytokines in releasate of platelets from control mice (zero weeks) and mice euthanized at either two, four, or eight weeks after immunity training, we made a 500 µl solution containing 2 × 108 platelets, thrombin (10 U/ml), 20 mM CaCl2, and 2 mM MgCl2 (final concentration). This combination produced a rapid release of α-granules, δ-granules, and lysosomes.28 After incubation of samples at 37°C for 20 minutes, the samples were centrifuged at 5,000× g for ten minutes, and the supernatant was aspirated and stored at -80°C for later analysis.
Assessment of platelet releasate
In the releasate, we measured the levels of platelet-related chemokines, cytokines, enzymes, and other small molecules.24,29,30 Levels of chemokine ligand (CCL)5, CXCL5, interleukin (IL)-4, IL-13, IL-17A, and tumour necrosis factor alpha (TNF-α) were measured using a ProcartaPlex️ kit (ThermoFisher, USA), according to the manufacturer’s instructions. Levels of the three main platelet-derived growth factor (PDGF) isoforms (AA, AB, and BB) were assessed, as were levels of serotonin, histamine, CXCL4, CXCL7, CXCL12, and cathepsin D using commercially available ELISA kits obtained from Abcam (UK).
Preparation of bacterial inoculum
We chose Staphylococcus aureus (BAA-1556; ATCC, USA) and Escherichia coli (BAA-196, ATCC) to serve as representative species of gram-positive and gram-negative bacteria in this study.31 Fresh bacterial cultures were prepared 24 hours before surgery and grown on tryptic soy agar (TSA). After washing the collected bacteria three times in sterile normal saline, we adjusted the concentration to 107 colony-forming units (CFUs) per ml according to a standard optical density curve.
Surgical procedures and bacterial inoculation
At 24 hours before and after surgery, samples of washed platelets obtained from control, two-, four-, and eight-week mice were transfused via tail vein injection; 1 × 109 washed platelets were injected per mouse, each injection. Anaesthesia of mice was maintained with volatile isoflurane (2.5%) after an intraperitoneal injection of an anaesthesia cocktail of ketamine (80 mg/kg) and xylazine (7.5 mg/kg). We disinfected the surgical site with povidone-iodine and ethanol, and used a medial parapatellar approach to access the articular surface of the distal femur. The femoral medullary canal was manually reamed with a 22-gauge needle. A J-shaped Kirschner wire (K-wire) (0.88 mm in diameter and 12 mm in length) was inserted reversely into the femoral canal from the joint space (Figure 1). To mimic intraoperative contamination, 1 × 104 CFUs of bacteria were applied to the K-wire immediately before insertion. To mimic haematogenous infection, 1 × 107 CFUs of bacteria were injected into the tail vein immediately after wound closure.
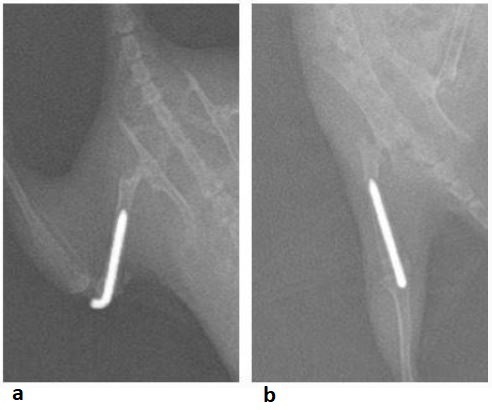
Fig. 1
Representative radiographs showing a surgically inserted Kirschner wire in the femoral canal in: a) lateral and b) anteroposterior (AP) views.
Bacterial cultures and species identification
Four weeks after K-wire surgery, mice were euthanized with carbon dioxide, and the joints with implants were harvested for bacterial culturing. The knee joint capsule, femur, and intramedullary K-wire were harvested separately and placed into three sterile tubes (each containing 3 ml of sterile normal saline). The femur and joint capsule were homogenized with a tissue grinder (Hoder, China). K-wires were sonicated to disrupt the biofilm and release bacteria, if any existed. The samples were then centrifuged, the TSA culture plates were inoculated with 30 µl of the sample supernatant, and the plates were incubated at 37°C for 48 hours. The culture plates were photographed using a digital camera.
Bacterial colonies from the tissue samples were analyzed and quantified using ImageJ (National Institutes of Health (NIH), USA). We defined bone and joint infection as a definite diagnosis if the sum of three colony counts (bone, joint capsule, and K-wire) exceeded 20 colonies (we set this threshold a priori) in cultured samples from a given mouse. Bacterial species were identified by 16S ribosomal DNA sequencing (MicroSeq 500 microbial identification system; ThermoFisher), following the manufacturer’s instructions. All bacteria isolated from the joint samples in this study were identified as the identical species in the initial inoculum.
Primary and secondary study outcomes
Our primary study outcome was the rate of bone and joint infection at four weeks after surgery, which was determined by the bacterial culturing of femur, K-wire, and joint capsules at four weeks. Our secondary outcome was the bacterial burden of the joint, which was determined by counting the number of colonies in cultured samples.
Sample size calculation, randomization, and blinding
The sample size was determined at a Type I error at 0.05, a power of 80%, and a superiority margin at 0. According to the previously reported data using this mouse model,14 in order to detect a 40% difference between treatment group and control group, at least 16 mice would be required. With this sample size, we could expect > 99% power to detect a potentially statistically significant difference. Considering the possible loss of mice by extra-experimental factors, we overpowered the starting sample size to 20 mice per group. A total of 100 mice were used. Block randomization was performed using RandoMice (version 1.0.9)32 to randomly assign the mice into each group. Except for one of the authors, who prepared the platelets for transfusion (TG), all other researchers in this study were blinded to the group assignment information.
Statistical analysis
SPSS version 26.0 (IBM, USA) was used for statistical analyses; significance was set as p < 0.05. Categorical and continuous variables are expressed as means (standard deviations (SDs)) and counts (percentages). Differences in the rate of bone and joint infection between any two groups were evaluated statistically using Fisher’s exact test. For comparing colony counts and factor levels in platelet releasate between any two groups, we used the non-parametric Mann-Whitney U test.
Results
Alterations in cytokine and chemokine levels in platelet releasate after immunity training
Enzyme-linked immunosorbent assay (ELISA) analyses of cytokines and chemokines in platelet releasate after immunity training showed that levels of TNF-α and IL-17A increased significantly compared to levels in platelet releasate from control mice not exposed to immunity training (Figure 2a). In contrast to these two pro-inflammatory cytokines, the levels of anti-inflammatory cytokines IL-4 and IL-13 decreased significantly after training (Figure 2a). In the two-, four-, and eight-week groups respectively, the mean levels of the chemokines CCL5 (1.84 (SD 0.47), 1.93 (SD 0.56), 2.13 (SD 0.77)), CXCL4 (2.77 (SD 0.25), 2.34 (SD 0.19), 2.51 (SD 0.23)), CXCL5 (1.56 (SD 0.31), 1.43 (SD 0.44), 1.77 (SD 0.23)), CXCL7 (2.15 (SD 0.56), 1.87 (SD 0.39), 2.00 (SD 0.41)), and CXCL12 (3.12 (SD 0.77), 2.87 (SD 0.64), 3.21 (SD 0.94)) also increased, but to different extents compared with those of the controls (Figure 2b). Other assessed factors in the platelet releasate (PDGF AA, PDGF AB, PDGF BB, cathepsin D, serotonin, and histamine) of immunity-trained mice did not change significantly compared to control levels (all p-values > 0.05; Figure 2c).
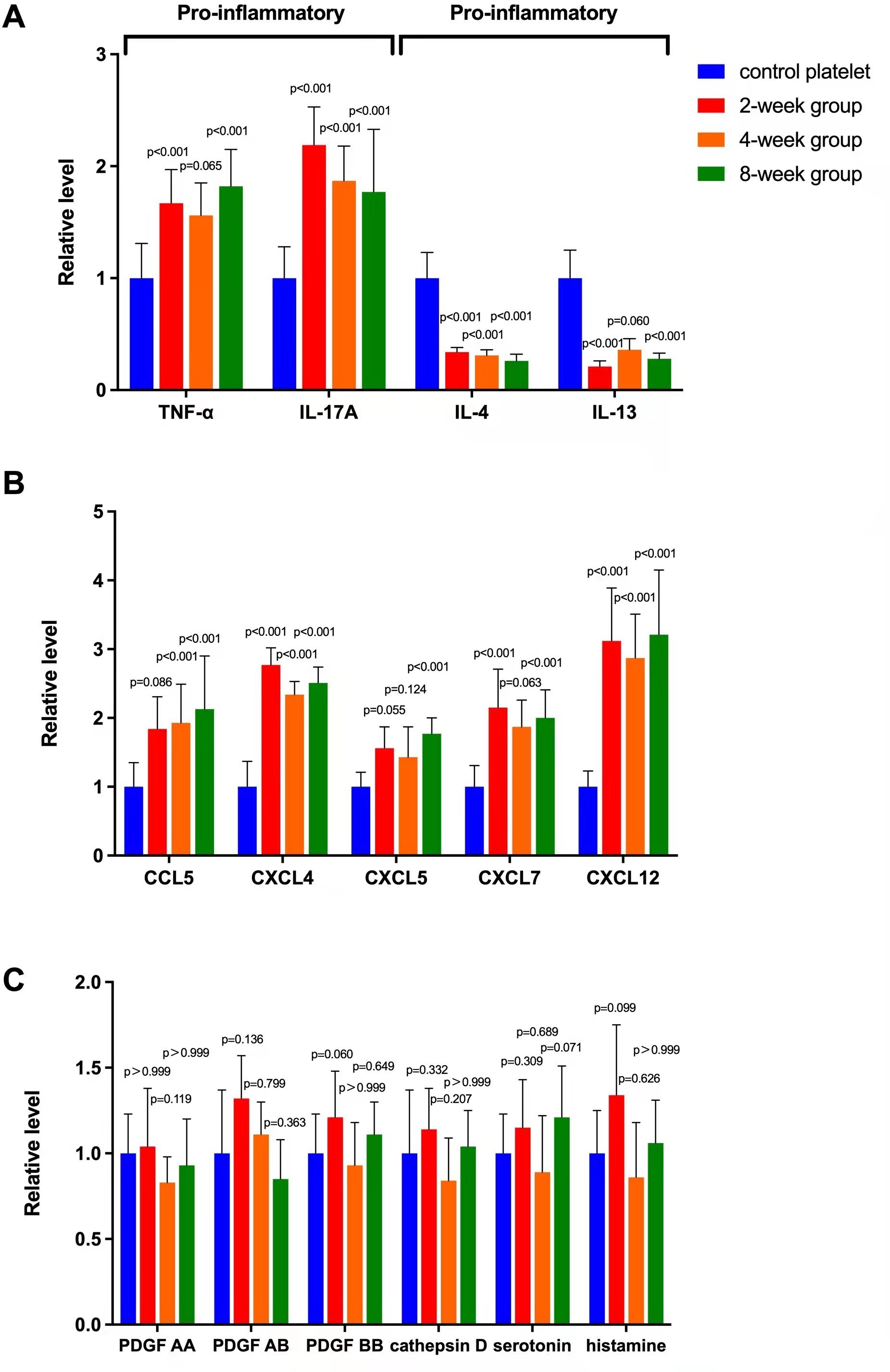
Fig. 2
Changes in levels of cytokines, chemokines, and other factors in platelet releasate after immunity training in a mouse model. a) Mean fold change by enzyme-linked immunoabsorbent assay analysis of pro-inflammatory (tumour necrosis factor alpha (TNF-α), interleukin (IL)-17A) and anti-inflammatory (IL-4, IL-13) cytokines in platelet releasate at different timepoints (two, four, and eight weeks) after an intravenous injection of saline (control), 0.1 mg of zymosan, and 0.1 mg of lipopolysaccharide in mice. Values are normalized to control levels. b) Mean fold change of chemokines (chemokine ligand (CCL)5, CXCL4, CXCL5, CXCL7, CXCL12) in the same immunity training and analysis protocol. c) Mean fold change in three isoforms of platelet-derived growth factor (PDGF), serotonin and histamine, and cathepsin D in platelet releasate under the same protocol. p < 0.05 versus platelet releasate levels in controls. Error bars in graphs here, and in all subsequent graphs, are standard deviations.
Transfusion of platelets from immunity-trained mice prevented bone and joint infection
As shown in Table I, mice that received a transfusion of trained platelets 24 hours before and after surgery had a reduced risk of bone and joint infection compared with those that received no transfusion after local inoculation with S. aureus (no transfusion: 100% (20/20); control platelet. As demonstrated in Figure 3, we observed a consistent pattern of bacterial burdens defined as colony counts of cultures (femur, joint capsule, K-wire).
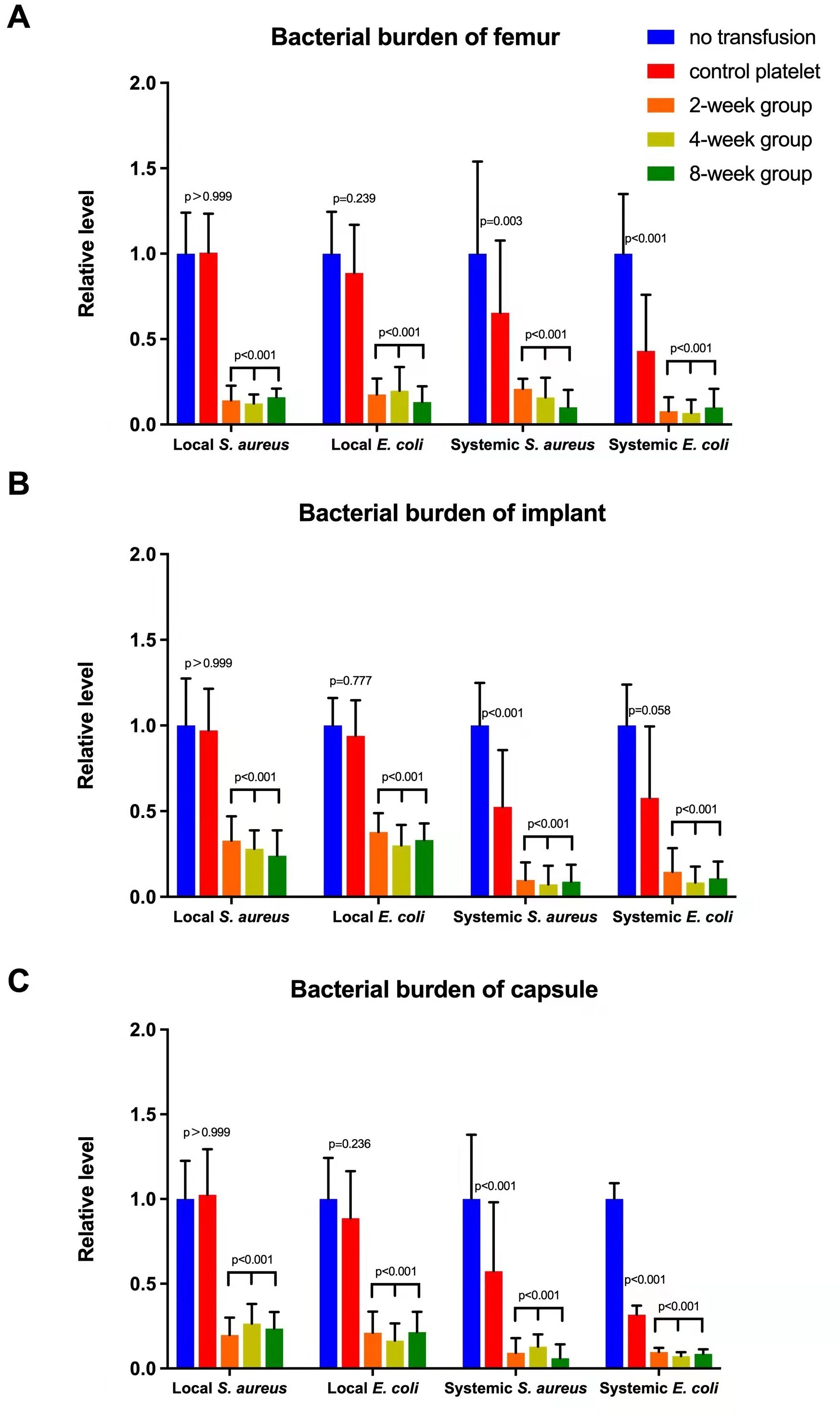
Fig. 3
Bacterial burden after bacterial challenge. Mean fold change of colony-forming units in cultures derived from surgical site samples after local inoculation or systemic injection with the indicated pathogens. Cultures were obtained from the a) femur, b) implant, and c) joint capsule. Values are normalized to no transfusion levels. p < 0.05 versus no transfusion. E. coli, Escherichia coli; S. aureus, Staphylococcus aureus.
Table I.
Bone and joint infections in mice transfused with platelets from immunity-trained mice after local or systemic challenge with bacteria. Values are presented as n (%).
Treatment* | Infection† | No infection | p-value |
---|---|---|---|
Local inoculation of S. aureus | |||
No transfusion | 20 (100) | 0 (0) | N/A |
Control | 19 (95) | 1 (5) | 1.000 |
2 wks | 11 (55) | 9 (45) | 0.001 |
4 wks | 9 (45) | 11 (55) | < 0.001 |
8 wks | 10 (50) | 10 (50) | < 0.001 |
Local inoculation of E. coli | |||
No transfusion | 17 (85) | 3 (15) | N/A |
Control | 18 (90) | 2 (10) | 1.000 |
2 wks | 8 (40) | 12 (60) | 0.008 |
4 wks | 10 (50) | 10 (50) | 0.041 |
8 wks | 8 (40) | 12 (60) | 0.008 |
Systemic injection of S. aureus | |||
No transfusion | 18 (90) | 2 (10) | N/A |
Control | 11 (55) | 9 (45) | 0.031 |
2 wks | 4 (20) | 16 (80) | <0.001 |
4 wks | 3 (15) | 17 (85) | <0.001 |
8 wks | 2 (10) | 18 (90) | <0.001 |
Systemic injection of E. coli | |||
No transfusion | 16 (80) | 4 (20) | N/A |
Control | 9 (45) | 11 (55) | 0.048 |
2 wks | 2 (10) | 18 (90) | <0.001 |
4 wks | 2 (10) | 18 (90) | <0.001 |
8 wks | 3 (15) | 17 (85) | <0.001 |
-
*
Experimental treatment consisted of platelets harvested from mice that were immunity-trained with zymosan and lipopolysaccharide for 0 (Control), two, four, or eight weeks.
-
†
Refers to all types of infections; positive culture results from bone, Kirschner wire, or joint capsule samples. Bacterial species were positively identified by 16S ribosomal DNA sequencing as identical to those at time of systemic or local injection.
-
‡
Chi-squared test.
-
E. coli, Escherichia coli; N/A, not applicable; S. aureus, Staphylococcus aureus.
Discussion
Training the innate immune system with vaccines and other immunity-stimulating substances is a promising approach to combat infectious disease.16,33 For instance, a randomized clinical trial demonstrated that Bacillus Calmette-Guérin vaccine not only enhanced the immune response to malarial infection, but also effectively reduced parasitaemia in human subjects after controlled malarial infection.34 Similarly, inoculation with zymosan or lipopolysaccharide can significantly enhance the immune response against bone and joint infection for up to eight weeks in a preclinical mouse model of intra-articular implants.14 To date, the mechanisms known to underlie trained immunity mainly involve functional alterations of immune cells, including monocytes, natural killer (NK) cells, and neutrophils.15-17 To the best of our knowledge, no previous study has evaluated the role of platelets in trained immunity.
Platelets play active roles in antibacterial defence, ranging from effecting complex interactions with bacteria to directly neutralizing pathogens and modulating immune responses.35 One of the most important mechanisms for platelets’ antibacterial actions is to release various bioactive substances pre-packaged from precursor megakaryocytes in response to bacterial invasion.21,22 In the present study, the immunity training-induced alteration in platelet releasate occurs mainly through α-granules (chemokines, cytokines, and growth factors) instead of δ-granules (small molecules) and lysosomes (degradative enzymes).23-25
The fundamental role of chemokines in immune defence is to direct immune cells from the wider circulation to a target site during bacterial invasion.36 Platelet-derived chemokine CXCL4 plays a critical role in bacterial clearance by recruiting neutrophils and monocytes for phagocytosis and enhancing these cells’ kill function.37-39 In this study, after transfusion of trained platelets, lymphocytes gathered on the surface of articular cartilage, while there was no such recruitment of immune cells after having been transfused with control platelet, as shown in Supplementary Figure a. In addition, CXCL4 is able to bind bacteria and induce a humoral immune response to CXCL4-coated bacteria.40 Importantly, the chemokine CCL5 can form heteromers with other chemokines and synergistically amplify inflammation.41 The possible roles of other chemokines that were elevated in platelet releasate are less straightforward, and further study is needed to elucidate whether they might be involved in mitigating against BJI.
Platelets carry both anti- and pro-inflammatory cytokines, contributing to a balanced inflammatory response.42 In the present study, we found that immunity training polarized platelets towards a pro-inflammatory phenotype, thereby elevating the levels of the pro-inflammatory cytokines TNF-α and IL-17A and lowering the levels of the anti-inflammatory cytokines IL-4 and IL-13. IL-17A can promote chemokine secretion by local cells and thus can recruit more neutrophils from circulation.43 It was discovered decades ago that TNF-α is crucial for bacterial clearance.44 Patients receiving anti-TNF-α therapy are at a substantial risk of developing bacterial infection.45 IL-4 and IL-13 share a common reporter IL4R-alpha, and thus have overlapping biological functions.46 Most current knowledge on the functions of IL-4 and IL-13 relates to their role in allergic hypersensitivity.47 Whether lowered levels of IL-4 and IL-13 are related to BJI mitigation remains unclear.
Interestingly, we found that the untrained platelets had an effect on infection after systemic challenge, but no effect after local challenge. We believed that the effect of untrained platelets is limited in local bacterial challenges because the burden of this challenge is stronger than systemic challenge. Bacteria always exist in the form of aggregates and tend to adhere to the surface of endophytes to form biofilms that are difficult to remove, which decreased the effect of untrained platelets in local challenge. In addition, the beginning of the reaction of untrained platelets took place in blood rather than local tissue. Thus, some active agents against bacterial invasion in blood are much higher than local, which causes these differences.
The current study had several limitations. First, we used only S. aureus and E. coli as representative pathogens for gram-positive and gram-negative bacteria, respectively. Many other pathogens can also cause bone and joint infection, and the efficacy of transfusion of platelets from immunity-trained mice might be more or less different for other pathogens. However, S. aureus and E. coli are among the most commonly isolated organisms in surgical-site infections.48 Second, we used classic agents for trained immunity: zymosan and lipopolysaccharide. Other substances (e.g. Bacillus Calmette-Guérin and measles vaccines) could also be used for immunity training. The impact of training with other substances on platelet releasate might be different. Future studies are needed to clarify this issue.
In conclusion, immunity training altered platelet releasates, specifically producing increased levels of inflammatory cytokines/chemokines and decreased levels of anti-inflammatory cytokines. Transfusion of platelets from immunity-trained mice conferred protection against bone and joint infection, suggesting that orchestrating changes in small signalling molecules released from platelets might be an important part of the mechanism underlying trained immunity.
References
1. Gao T , Lin J , Zhang C , Zhu H , Zheng X . Is intracellular Staphylococcus aureus associated with recurrent infection in a rat model of open fracture? Bone Joint Res . 2020 ; 9 ( 2 ): 71 – 76 . Crossref PubMed Google Scholar
2. Yu K , Song L , Kang HP , Kwon H-K , Back J , Lee FY . Recalcitrant methicillin-resistant Staphylococcus aureus infection of bone cells: intracellular penetration and control strategies . Bone Joint Res . 2020 ; 9 ( 2 ): 49 – 59 . Crossref PubMed Google Scholar
3. Ramalhete R , Brown R , Blunn G , et al. A novel antimicrobial coating to prevent periprosthetic joint infection . Bone Joint Res . 2020 ; 9 ( 12 ): 848 – 856 . Crossref PubMed Google Scholar
4. Diacovich L , Gorvel JP . Bacterial manipulation of innate immunity to promote infection . Nat Rev Microbiol . 2010 ; 8 ( 2 ): 117 – 128 . Crossref PubMed Google Scholar
5. Berthelot JM , Sibilia J . Trained immunity and autoimmune disease: did Eve sin before Adam? Joint Bone Spine . 2019 ; 86 ( 3 ): 293 – 295 . Crossref PubMed Google Scholar
6. Lord JM , Midwinter MJ , Chen Y-F , et al. The systemic immune response to trauma: an overview of pathophysiology and treatment . Lancet . 2014 ; 384 ( 9952 ): 1455 – 1465 . Crossref PubMed Google Scholar
7. Lenguerrand E , Whitehouse MR , Beswick AD , et al. Risk factors associated with revision for prosthetic joint infection following knee replacement: an observational cohort study from England and Wales . Lancet Infect Dis . 2019 ; 19 ( 6 ): 589 – 600 . Crossref PubMed Google Scholar
8. Kapadia BH , Berg RA , Daley JA , Fritz J , Bhave A , Mont MA . Periprosthetic joint infection . Lancet . 2016 ; 387 ( 10016 ): 386 – 394 . Crossref PubMed Google Scholar
9. Mina MJ , Metcalf CJE , de Swart RL , Osterhaus A , Grenfell BT . Long-term measles-induced immunomodulation increases overall childhood infectious disease mortality . Science . 2015 ; 348 ( 6235 ): 694 – 699 . Crossref PubMed Google Scholar
10. Butkeviciute E , Jones CE , Smith SG . Heterologous effects of infant BCG vaccination: potential mechanisms of immunity . Future Microbiol . 2018 ; 13 : 1193 – 1208 . Crossref PubMed Google Scholar
11. Sharrock J , Sun JC . Innate immunological memory: from plants to animals . Curr Opin Immunol . 2020 ; 62 : 69 – 78 . Crossref PubMed Google Scholar
12. Angulo M , Reyes-Becerril M , Cepeda-Palacios R , Angulo C , et al. Oral administration of Debaryomyces hansenii CBS8339-β-glucan induces trained immunity in newborn goats . Dev Comp Immunol . 2020 ; 105 : 103597 . Crossref PubMed Google Scholar
13. Ciarlo E , Heinonen T , Théroude C , et al. Trained immunity confers broad-spectrum protection against bacterial infections . J Infect Dis . 2020 ; 222 ( 11 ): 1869 – 1881 . Crossref PubMed Google Scholar
14. Zhu H , Lin J , Wei H , Bao B , Gao T , Zheng X , et al. Does training innate immunity confer broad-spectrum protection against bone and joint infection in a mouse model? Clin Orthop Relat Res . 2020 ; 478 ( 11 ): 2670 – 2681 . Crossref PubMed Google Scholar
15. Messina NL , Zimmermann P , Curtis N . The impact of vaccines on heterologous adaptive immunity . Clin Microbiol Infect . 2019 ; 25 ( 12 ): 1484 – 1493 . Crossref PubMed Google Scholar
16. Netea MG , Joosten LAB , Latz E , et al. Trained immunity: a program of innate immune memory in health and disease . Science . 2016 ; 352 ( 6284 ): aaf1098 . Crossref PubMed Google Scholar
17. Arts RJW , Moorlag S , Novakovic B , et al. BCG vaccination protects against experimental viral infection in humans through the induction of cytokines associated with trained immunity . Cell Host Microbe . 2018 ; 23 ( 1 ): 89 – 100 . Crossref PubMed Google Scholar
18. Khader SA , Divangahi M , Hanekom W , et al. Targeting innate immunity for tuberculosis vaccination . J Clin Invest . 2019 ; 129 ( 9 ): 3482 – 3491 . Crossref PubMed Google Scholar
19. D’Atri LP , Rodríguez CS , Miguel CP , et al. Activation of toll-like receptors 2 and 4 on CD34+ cells increases human megakaryo/thrombopoiesis induced by thrombopoietin . J Thromb Haemost . 2019 ; 17 ( 12 ): 2196 – 2210 . Crossref PubMed Google Scholar
20. Blair P , Rex S , Vitseva O , et al. Stimulation of Toll-like receptor 2 in human platelets induces a thromboinflammatory response through activation of phosphoinositide 3-kinase . Circ Res . 2009 ; 104 ( 3 ): 346 – 354 . Crossref PubMed Google Scholar
21. Koupenova M , Clancy L , Corkrey HA , Freedman JE . Circulating platelets as mediators of immunity, inflammation, and thrombosis . Circ Res . 2018 ; 122 ( 2 ): 337 – 351 . Crossref PubMed Google Scholar
22. Thomas MR , Storey RF . The role of platelets in inflammation . Thromb Haemost . 2015 ; 114 ( 3 ): 449 – 458 . Crossref PubMed Google Scholar
23. Smith CCT , Prichard BNC , Cooper MB . Platelet alpha- and beta-secretase activities: a preliminary study in normal human subjects . Platelets . 2009 ; 20 ( 1 ): 29 – 34 . Crossref PubMed Google Scholar
24. Rendu F , Brohard-Bohn B . The platelet release reaction: granules’ constituents, secretion and functions . Platelets . 2001 ; 12 ( 5 ): 261 – 273 . Google Scholar
25. Badran Z , Abdallah M-N , Torres J , Tamimi F . Platelet concentrates for bone regeneration: cCurrent evidence and future challenges . Platelets . 2018 ; 29 ( 2 ): 105 – 112 . Google Scholar
26. Nurden AT , Nurden P , Sanchez M , Andia I , Anitua E . Platelets and wound healing . Front Biosci . 2008 ; 13 : 3532 – 3548 . Crossref PubMed Google Scholar
27. Aurbach K , Spindler M , Haining EJ , Bender M , Pleines I . Blood collection, platelet isolation and measurement of platelet count and size in mice-a practical guide . Platelets . 2019 ; 30 ( 6 ): 698 – 707 . Crossref PubMed Google Scholar
28. Vélez P , Izquierdo I , Rosa I , García Á . A 2D-DIGE-based proteomic analysis reveals differences in the platelet releasate composition when comparing thrombin and collagen stimulations . Sci Rep . 2015 ; 5 : 8198 . Crossref PubMed Google Scholar
29. Bakogiannis C , Sachse M , Stamatelopoulos K , Stellos K . Platelet-derived chemokines in inflammation and atherosclerosis . Cytokine . 2019 ; 122 : 154157 . Crossref PubMed Google Scholar
30. Amable PR , Carias RBV , Teixeira MVT , et al. Platelet-rich plasma preparation for regenerative medicine: optimization and quantification of cytokines and growth factors . Stem Cell Res Ther . 2013 ; 4 ( 3 ): 67 . Crossref PubMed Google Scholar
31. Zhu H , Bao B , Wei H , et al. Is EDTA irrigation effective in reducing bacterial infection in a rat model of contaminated intra-articular knee implants? Clin Orthop Relat Res . 2020 ; 478 ( 5 ): 1111 – 1121 . Crossref PubMed Google Scholar
32. van Eenige R , Verhave PS , Koemans PJ , Tiebosch I , Rensen PCN , Kooijman S . RandoMice, a novel, user-friendly randomization tool in animal research . PLoS One . 2020 ; 15 ( 8 ): e0237096 . Crossref PubMed Google Scholar
33. Zhidong H , Douglas BL , Xiao-Yong F . Statins as adjunctive therapy against tuberculosisthe balance between statin-induced anti-tb effect and trained immunity suppression . J Infect Dis . 2019 ; 24 : 5686380 . Google Scholar
34. Walk J , de Bree LCJ , Graumans W , et al. Outcomes of controlled human malaria infection after BCG vaccination . Nat Commun . 2019 ; 10 ( 1 ): 874 . Crossref PubMed Google Scholar
35. Page MJ , Pretorius E . A champion of host defense: A generic large-scale cause for platelet dysfunction and depletion in infection . Semin Thromb Hemost . 2020 ; 46 ( 3 ): 302 – 319 . Crossref PubMed Google Scholar
36. Rajarathnam K , Schnoor M , Richardson RM , Rajagopal S . How do chemokines navigate neutrophils to the target site: Dissecting the structural mechanisms and signaling pathways . Cell Signal . 2019 ; 54 : 69 – 80 . Crossref PubMed Google Scholar
37. Yue L , Pang Z , Li H , et al. CXCL4 contributes to host defense against acute Pseudomonas aeruginosa lung infection . PLoS One . 2018 ; 13 ( 10 ): e0205521 . Crossref PubMed Google Scholar
38. Hwaiz R , Rahman M , Zhang E , Thorlacius H . Platelet secretion of CXCL4 is Rac1-dependent and regulates neutrophil infiltration and tissue damage in septic lung damage . Br J Pharmacol . 2015 ; 172 ( 22 ): 5347 – 5359 . Crossref PubMed Google Scholar
39. Fox JM , Kausar F , Day A , et al. CXCL4/platelet factor 4 is an agonist of CCR1 and drives human monocyte migration . Sci Rep . 2018 ; 8 ( 1 ): 9466 . Crossref PubMed Google Scholar
40. Krauel K , Pötschke C , Weber C , et al. Platelet factor 4 binds to bacteria, [corrected] inducing antibodies cross-reacting with the major antigen in heparin-induced thrombocytopenia . Blood . 2011 ; 117 ( 4 ): 1370 – 1378 . Crossref PubMed Google Scholar
41. von Hundelshausen P , Agten SM , Eckardt V , et al. Chemokine interactome mapping enables tailored intervention in acute and chronic inflammation . Sci Transl Med . 2017 ; 9 ( 384 ): 384 . Crossref PubMed Google Scholar
42. Boshtam M , Asgary S , Kouhpayeh S , Shariati L , Khanahmad H . Aptamers against pro- and anti-inflammatory cytokines: a review . Inflammation . 2017 ; 40 ( 1 ): 340 – 349 . Crossref PubMed Google Scholar
43. Qiao S , Zhang H , Zha X , et al. Endogenous IL-17A mediated neutrophil infiltration by promoting chemokines expression during chlamydial lung infection . Microb Pathog . 2019 ; 129 : 106 – 111 . Crossref PubMed Google Scholar
44. Malaviya R , Ikeda T , Ross E , Abraham SN , et al. Mast cell modulation of neutrophil influx and bacterial clearance at sites of infection through TNF-alpha . Nature . 1996 ; 381 ( 6577 ): 77 – 80 . Crossref PubMed Google Scholar
45. Strangfeld A , Listing J . Infection and musculoskeletal conditions: bacterial and opportunistic infections during anti-TNF therapy . Best Pract Res Clin Rheumatol . 2006 ; 20 ( 6 ): 1181 – 1195 . Crossref PubMed Google Scholar
46. Mueller TD , Zhang J-L , Sebald W , Duschl A . Structure, binding, and antagonists in the IL-4/IL-13 receptor system . Biochim Biophys Acta . 2002 ; 1592 ( 3 ): 237 – 250 . Crossref PubMed Google Scholar
47. Junttila IS . Tuning the cytokine responses: An update on interleukin (IL)-4 and IL-13 receptor complexes . Front Immunol . 2018 ; 9 : 888 . Crossref PubMed Google Scholar
48. Owens CD , Stoessel K . Surgical site infections: epidemiology, microbiology and prevention . J Hosp Infect . 2008 ; 70 Suppl 2 : 3 – 10 . Crossref PubMed Google Scholar
Author contributions
T. Gao: Investigation, Writing – original draft.
J. Lin: Investigation.
H. Wei: Formal analysis.
B. Bao: Formal analysis.
H. Zhu: Conceptualization.
X. Zheng: Conceptualization, Writing – review & editing.
Funding statement
The author(s) disclose receipt of the following financial or material support for the research, authorship, and/or publication of this article: the National Natural Science Foundation of China (Grant 82002324, Hongyi Zhu; Grant 81974331, Xianyou Zheng); the Clinical Research Project of Shanghai Municipal Health Commission (Grant 20194Y0254, Hongyi Zhu).
ICMJE COI statement
The authors declare that they have no competing interests.
Acknowledgements
We thank Dr Changqing Zhang, MD, PhD for constructive suggestion on the study design.
Ethical review statement
Each author certifies that his or her institution approved the animal protocol for this investigation and that all investigations were conducted in conformity with ethical principles of research.
Open access funding
The authors confirm that the open access fee for this study was self-funded.
Supplementary material
Figure showing histological analyses of the knee joint four weeks after transfusion, and ARRIVE checklist.