Abstract
Objectives
Modular junctions are ubiquitous in contemporary hip arthroplasty. The head-trunnion junction is implicated in the failure of large diameter metal-on-metal (MoM) hips which are the currently the topic of one the largest legal actions in the history of orthopaedics (estimated costs are stated to exceed $4 billion). Several factors are known to influence the strength of these press-fit modular connections. However, the influence of different head sizes has not previously been investigated. The aim of the study was to establish whether the choice of head size influences the initial strength of the trunnion-head connection.
Materials and Methods
Ti-6Al-4V trunnions (n = 60) and two different sizes of cobalt-chromium (Co-Cr) heads (28 mm and 36 mm; 30 of each size) were used in the study. Three different levels of assembly force were considered: 4 kN; 5 kN; and 6 kN (n = 10 each). The strength of the press-fit connection was subsequently evaluated by measuring the pull-off force required to break the connection. The statistical differences in pull-off force were examined using a Kruskal–Wallis test and two-sample Mann–Whitney U test. Finite element and analytical models were developed to understand the reasons for the experimentally observed differences.
Results
36 mm diameter heads had significantly lower pull-off forces than 28 mm heads when impacted at 4 kN and 5 kN (p < 0.001; p < 0.001), but not at 6 kN (p = 0.21). Mean pull-off forces at 4 kN and 5 kN impaction forces were approximately 20% larger for 28 mm heads compared with 36 mm heads. Finite element and analytical models demonstrate that the differences in pull-off strength can be explained by differences in structural rigidity and the resulting interface pressures.
Conclusion
This is the first study to show that 36 mm Co-Cr heads have up to 20% lower pull-off connection strength compared with 28 mm heads for equivalent assembly forces. This effect is likely to play a role in the high failure rates of large diameter MoM hips.
Cite this article: A. R. MacLeod, N. P. T. Sullivan, M. R. Whitehouse, H. S. Gill. Large-diameter total hip arthroplasty modular heads require greater assembly forces for initial stability. Bone Joint Res 2016;5:338–346. DOI: 10.1302/2046-3758.58.BJR-2016-0044.R1.
Article focus
-
To evaluate whether the initial connection strength of modular total hip arthroplasty heads is influenced by the size of the head used;
-
To investigate why the observed differences occur.
Key messages
-
The larger head size used in our study (36 mm) produced lower connection strengths than the smaller head size (28 mm) for the same assembly forces;
-
The increased rigidity of the larger diameter heads is the reason for the reduction in initial connection strength.
Strengths and limitations
-
Experimental study with a large number of samples (n = 60);
-
Experimental results supported by finite element and analytical modelling;
-
Only two head sizes were examined (28 mm and 36 mm).
Introduction
Modular junctions are ubiquitous in contemporary hip arthroplasty. The head-trunnion junction is implicated in the failure of large diameter metal-on-metal (MoM) hips,1,2 which are the currently the topic of one the largest legal actions in the history of orthopaedics (the estimated cost to Johnson & Johnson is stated to exceed $4 billion3). Modularity has the advantage of allowing intra-operative correction of anatomy (length and offset) and stability, decreasing implant inventories4-6 and permitting future revision of the femoral head while retaining the femoral stem.5-7 Assembly of these components is via a press-fit connection comprising a ‘male’ taper (trunnion) and a ‘female’ ball (head).4,5 Relative motion at the trunnion-head interface, however, can produce significant amounts of metallic debris4,8 and contribute to fretting and corrosion.5,9,10 The metal debris associated with trunnion fretting and corrosion can induce biological activity responsible for adverse local tissue reactions1,9-12 and secondary inflammatory pseudotumour formation.13,14
MoM resurfacing and THA popularised the use of larger-diameter heads, going up to 60 mm.15 In THA, diameters larger than 28 mm are gaining popularity due to the reduced risk of dislocation,16-18 however, it has been suggested that 36 mm is the largest head size that should be used17 as larger head sizes have been associated with increased fretting and corrosion at the trunnion-head interface.6,8,17,19-21 Following the unexpected problems with MoM articulations, a report by SCENIHR (Scientific Committee on Emerging and Newly Identified Health Risks) stated: “These larger heads put larger loads on the taper junction and are suspected to be responsible for the problems”.22 The importance of head size regarding implant failures is clear: failure rates of MoM articulations in THA are strongly predicted by head size.15,23 This is also supported by retrieval studies which show that corrosion and fretting scores correlate strongly with increasing head offset (lever arm between the centre of the head and the centre of pressure of the trunnion) and head size.8,9,24 Even with bearing types other than MoM, studies have reported corrosion and wear at the taper junction,2,9,13,25,26 with the majority relating to larger head sizes.2 For example, evidence of significantly increased fretting and corrosion has been seen in 36 mm, compared with 28 mm, heads in metal-on-polyethylene (MoP) bearings.27
The assembly conditions are known to influence the integrity of modular junctions.6,18,25,28-30 For example, greater assembly forces have been shown to increase the contact area at the head-trunnion interface31 and reduce the rate of corrosion.32 No studies, however, have considered the effect of assembly force in relation to head size. It is not clear, therefore, if taper connections using larger-diameter heads are compromised at the point of assembly, or if the differences between head sizes are entirely due to the increased lever arm accelerating the rate of damage accumulation under physiological loading. Additionally, understanding the mechanical environment around the head-trunnion interface may help to explain differences in performance between head sizes and drive design improvements. The aim of the current study was to investigate whether head size influences initial pull-off strength at different levels of impaction force. In order to achieve this aim, we used a combination of experimental, numerical modelling and analytical methods.
Materials and Methods
Experimental Testing
A total of 60 cobalt-chromium (Co-Cr) heads (diameter 36 mm: n = 30 and diameter 28 mm: n = 30; medium offset (+0 mm)), along with 60 12/14 titanium alloy (Ti-6Al-4V) trunnions, were used in the study.32 All specimens were manufactured by an orthopaedic device manufacturer (JRI Orthopaedics, Sheffield, United Kingdom) with the angle of the male and female tapers equal to 5.67°, standard deviation (sd) 0.08°, and an engagement length (along the trunnion axis) of 16.41 mm. The trunnions were manufactured without the femoral stem for ease of testing.
A custom-made impaction rig, similar to that previously described by Rehmer, Bishop and Morlock,33 was designed using a swinging hammer (Fig. 1a). The material of the impactor tip is known to influence the assembly,33 therefore a 6.3 mm thick plastic cap (Nylon-66) was used to reduce the impulse transmitted to the prosthesis compared with a direct MoM blow.34 The impaction load vector was aligned with the taper axis using a digital inclinometer (STM – SmartTool Digital Inclinometer 360°; M-D Building Products, Inc., Oklahoma City, Oklahoma). Each trunnion specimen was secured in a steel specimen holder using two 3 mm pins. The specimen holder was tightened to the rig base with a torque of 40 Nm. Prior to impaction, a settling force equal to the weight of the impactor (1.52 kg) was used to ensure consistency between specimens.
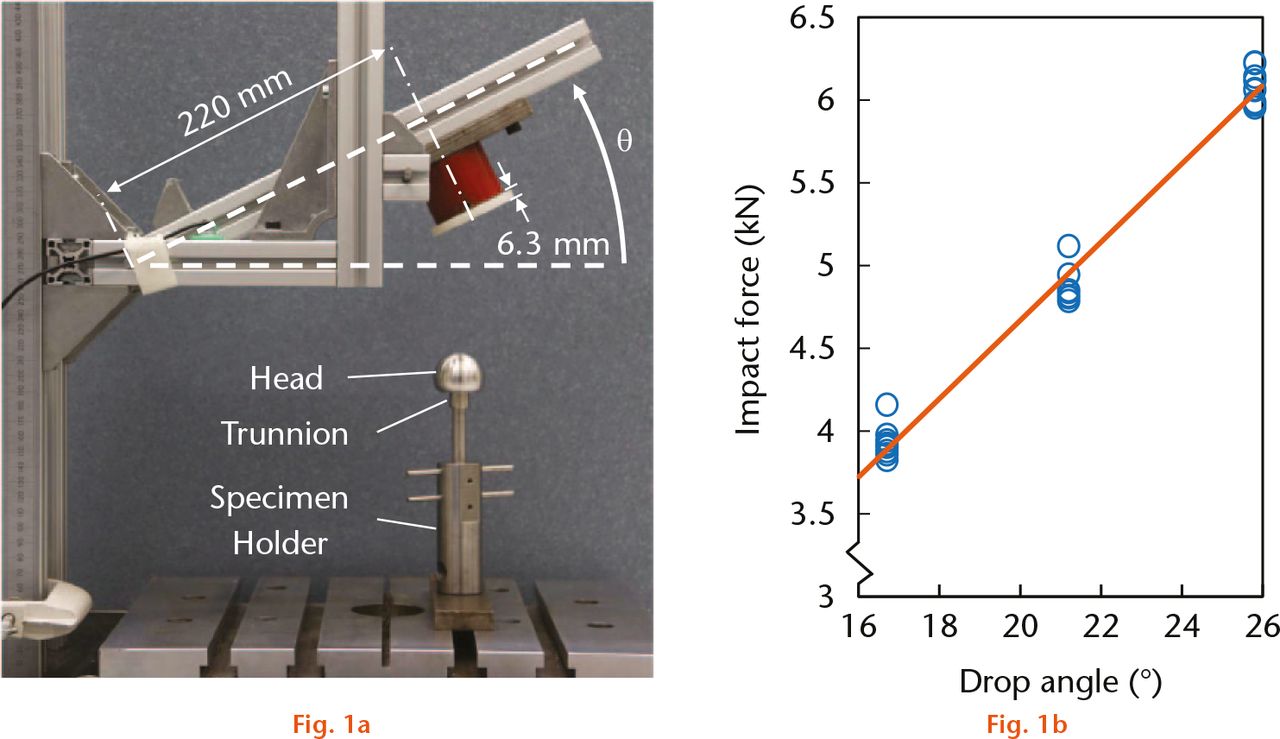
Fig.
An illustration showing, a) experimental set-up showing drop rig and test specimen and a graph showing b) the influence of drop angle and impaction force showing ten repeats for each drop angle.
The relationship between impaction rig inclination angle and measured peak impaction force is shown in Figure 1b. Peak impaction forces produced by our rig were found to be highly repeatable with linear regression of peak force versus drop height producing an R2 value of 0.990 (95% confidence interval (CI) 0.984 to 0.996). The ambient temperature in the room during testing was between 20.5°C and 21.0°C. All specimens had been stored in the room for at least 24 hours prior to testing.
The mean impaction force applied by surgeons has been reported to be approximately 4.4 kN.28 The test specimens were divided into six groups (n = 10 per group) consisting of three impaction levels (4 kN; 5 kN and 6 kN) for each head diameter. The assembled specimens were then disassembled using a materials testing machine (Series 5965; Instron, Norwood, Massachusetts). The heads were removed from the stems by applying tensile displacement at a rate of 0.008 mm/s (sd 0.0008), maintaining the alignment tolerances specified in the ISO 7206-10: 2003 standard for axisymmetric experiments.35
The surface roughness of every trunnion was assessed before and after testing using a non-contact profiler (Proscan 2000; sensor model: S11/03; resolution: 12 nm; Scantron Industrial Products Ltd, Taunton, United Kingdom). A linear profile traversing the entire engagement length was taken at the same location for both scans. The trunnions were assessed using the maximum height of the asperities within the sampling length (5 mm) compared with the mean surface height, Rp.
The statistical differences in pull-off force and Rp measurements between the head sizes were examined using a Kruskal–Wallis test and two-sample Mann–Whitney U–test using SPSS (IBM Corp. Released 2013. IBM SPSS Statistics for Windows, Version 22.0. IBM Corp., Armonk, New York). Spearman’s rank correlation coefficient was also used to identify any relationship between the roughness measures and pull-off force.
Finite element analysis
To investigate differences in internal stresses between the head sizes, an axisymmetric finite element (FE) model of the head and trunnion was created (Abaqus 6.12; Simulia Corp., Providence, Rhode Island) using dimensions provided by the manufacturer. A mesh convergence study was performed with convergence occurring at 2068 linear tetrahedral elements, with a mean element edge length at the contacting surfaces of 0.25 mm. Increasing the mesh density by a factor of 20 altered stress predictions by 0.25% and did not change pull-off force predictions. The contact interface between the trunnion and the head was represented using a surface-to-surface contact with a standard coulomb friction coefficient of 0.25.36-38 In order to introduce variability into the model, two additional frictional coefficients were evaluated (0.2 and 0.3)7,36 and used to provide a range of results. The analysis was conducted in two steps: quasi-static loading representing the peak impaction forces used in the experimental tests (4, 5 and 6 kN) followed by pull-off by displacement. The maximum tensile force during disassembly (equivalent to pull-off strength) was recorded for each head size.
Analytical modelling
In order to understand the mechanics of the problem, a simple analytical model was developed, assuming the entire surface of the trunnion and head to be in contact and the interface pressure to be evenly distributed.38,39 Using equations from MacDonald et al38 and Fessler and Fricker,39 the force arising from frictional resistance at the trunnion-head interface,
The net assembly force is equal to the impaction force minus the total frictional resistance,
The resistance of the head to press-fit assembly,
Balancing the net assembly force,
Assuming the head to act as a thick-walled cylinder surrounding the trunnion, the interface pressure,
Where rf is the distance to the interface,
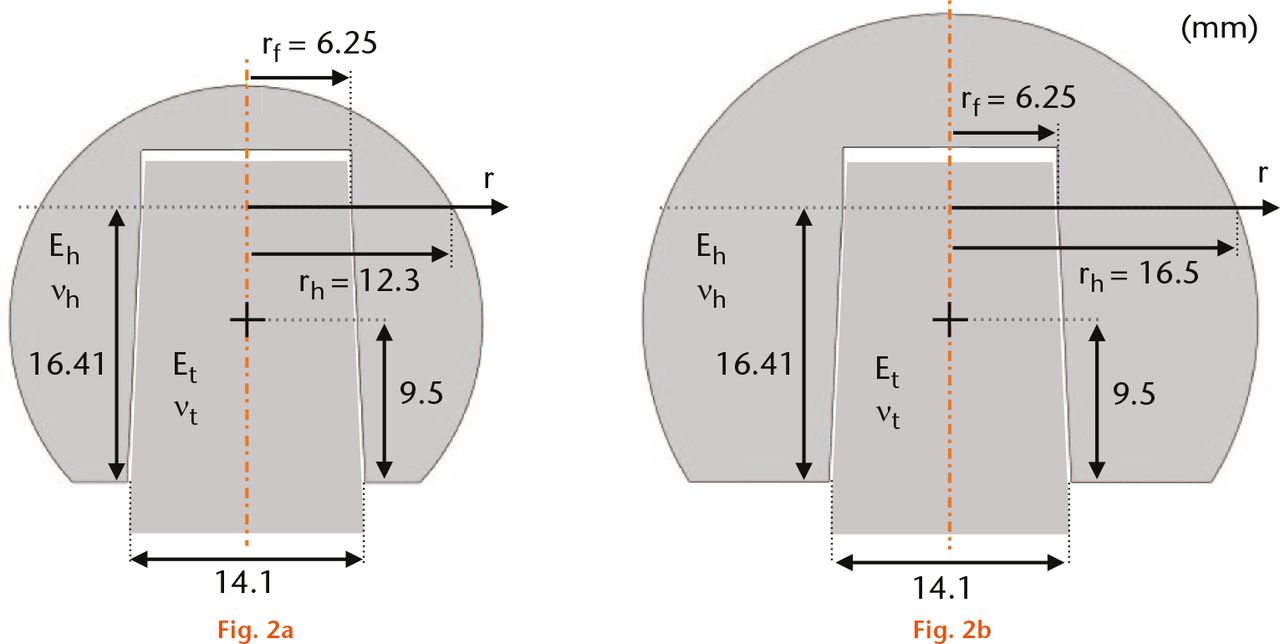
Fig.
Key dimensions for (a) 28 mm a (b) 36 mm diameter heads.
Re-arranging Equation 6 for
The radial stress,
During pull-off, extraction forces are resisted by frictional forces, τ, but a component of the interface pressure, pf, contributes:
As the radial stress at the interface,
As the frictional coefficient and contact area are equal for the two head sizes, the pull-off force is entirely dependent upon the interference generated and is linear with force, with the slope influenced by head size. In order to assess the relative rigidity of the two head sizes, the force per unit interference was calculated using Equation 7, and termed “interference rigidity”.
Results
Experimental testing
The larger-diameter heads were found to produce significantly lower pull-off forces at the 4 kN and 5 kN levels of impaction force (p < 0.001; p < 0.001) but not at 6 kN (p = 0.21) (Table I). Mean (sd) pull-off forces for the three load levels (4 kN, 5 kN, 6 kN) were: 1492.3 N (sd 101.3); 1817.5 N (sd 150.4); and 1704.0 N (sd 162.3) , respectively, for the 28 mm heads and 1235.7 N (sd 77.8); 1521.7 N (sd 79.9); 1786.7 N (sd 72.9), respectively, for the 36 mm heads (Fig. 3). For the 4 and 5 kN impaction forces, the pull-off force was, on average, 37% of the assembly force for the 28 mm heads and 31% for the 36 mm heads. Pull-off forces were 20.8% and 19.5% larger for the 28 mm heads at 4 kN and 5 kN, respectively.
Table I.
Pull-off force for the different head size groups and impaction forces
Head size = 28 mm |
Head size = 36 mm |
Mann–Whitney U test | Kruskal–Wallis test | ||||
---|---|---|---|---|---|---|---|
Pull-off force (N) |
Pull-off force (N) |
||||||
Mean | sd | Mean | sd | ||||
Impaction force (kN) | 4 | 1492.3 | 101.3 | 1235.7 | 77.8 | p < 0.001 | p < 0.001 |
5 | 1817.5 | 150.4 | 1521.7 | 79.9 | p < 0.001 | ||
6 | 1704.0 | 162.3 | 1786.7 | 72.9 | p = 0.21 |
-
sd, standard deviation; kN, kilonewtons
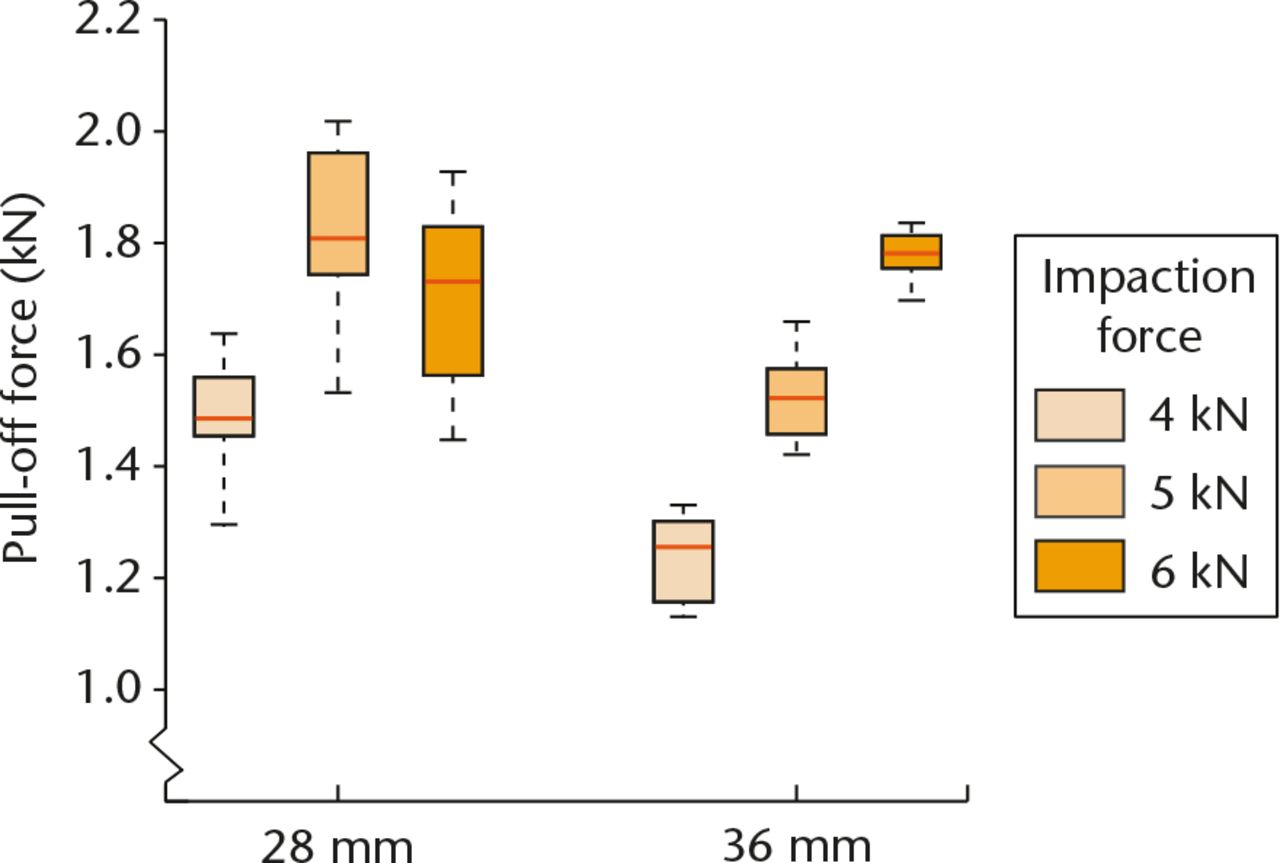
Fig. 3
Graph showing pull-off forces for different head sizes and impaction forces used in the study.
The trunnions for both head size groups were found to have similar roughness, Rp, before (p > 0.40) and after (p > 0.42) testing at all load levels (Table II). There was also no difference between head sizes considering the change in roughness before and after the tests (p > 0.81). Additionally, there was no relationship between pull-off force and initial, post-testing or change in roughness. The Spearman’s rank correlation coefficient for each measure was −0.046 (p = 0.73); –0.095 (p = 0.47); and −0.066 (p = 0.62), respectively.
Table II.
Values of roughness, Rp, pre-testing, post-testing and the change in roughness for the different head size groups and impaction forces
Head size = 28 mm |
Head size = 36 mm |
Mann–Whitney U test | Kruskal–Wallis test | ||||
---|---|---|---|---|---|---|---|
Roughness pre-test, Rp, (μm) |
Roughness pre-test, Rp, (μm) |
||||||
Mean | sd | Mean | sd | ||||
Impaction force (kN) | 4 | 16.38 | 0.636 | 15.83 | 0.975 | p = 0.16 | p = 0.40 |
5 | 16.77 | 2.380 | 15.90 | 0.832 | p = 0.34 | ||
6 | 15.95 | 1.261 | 16.35 | 1.631 | p = 0.47 | ||
Roughness post-test, Rp, (μm) |
Roughness post-test, Rp, (μm) |
||||||
Mean | sd | Mean | sd | ||||
Impaction force (kN) | 4 | 16.30 | 0.851 | 18.15 | 5.055 | p = 0.85 | p = 0.42 |
5 | 17.15 | 3.188 | 15.57 | 0.894 | p = 0.38 | ||
6 | 17.39 | 4.261 | 15.81 | 0.989 | p = 0.57 | ||
Change in roughness (post- pre), Rp, (μm) |
Change in roughness (post- pre), Rp, (μm) |
||||||
Mean | sd | Mean | sd | ||||
Impaction force (kN) | 4 | –0.075 | 0.851 | 2.315 | 5.055 | p = 0.21 | p = 0.81 |
5 | 0.378 | 3.188 | –0.328 | 0.894 | p = 0.91 | ||
6 | 1.442 | 4.261 | –0.540 | 0.989 | p = 0.79 |
-
sd, standard deviation; Rp, surface height
Finite element analysis and analytical modelling
Although the absolute values of pull-off forces predicted by the finite element (FE) simulation were larger than those in the experimental testing, (Fig. 4), the relative difference in pull-off force between head sizes matched the experiments closely at the 4 kN and 5 kN levels of impaction force. The FE predictions were 20.0%, 18.4% and 19.1% larger for 28 mm heads at the 4 kN, 5 kN and 6 kN levels of impaction force, respectively.
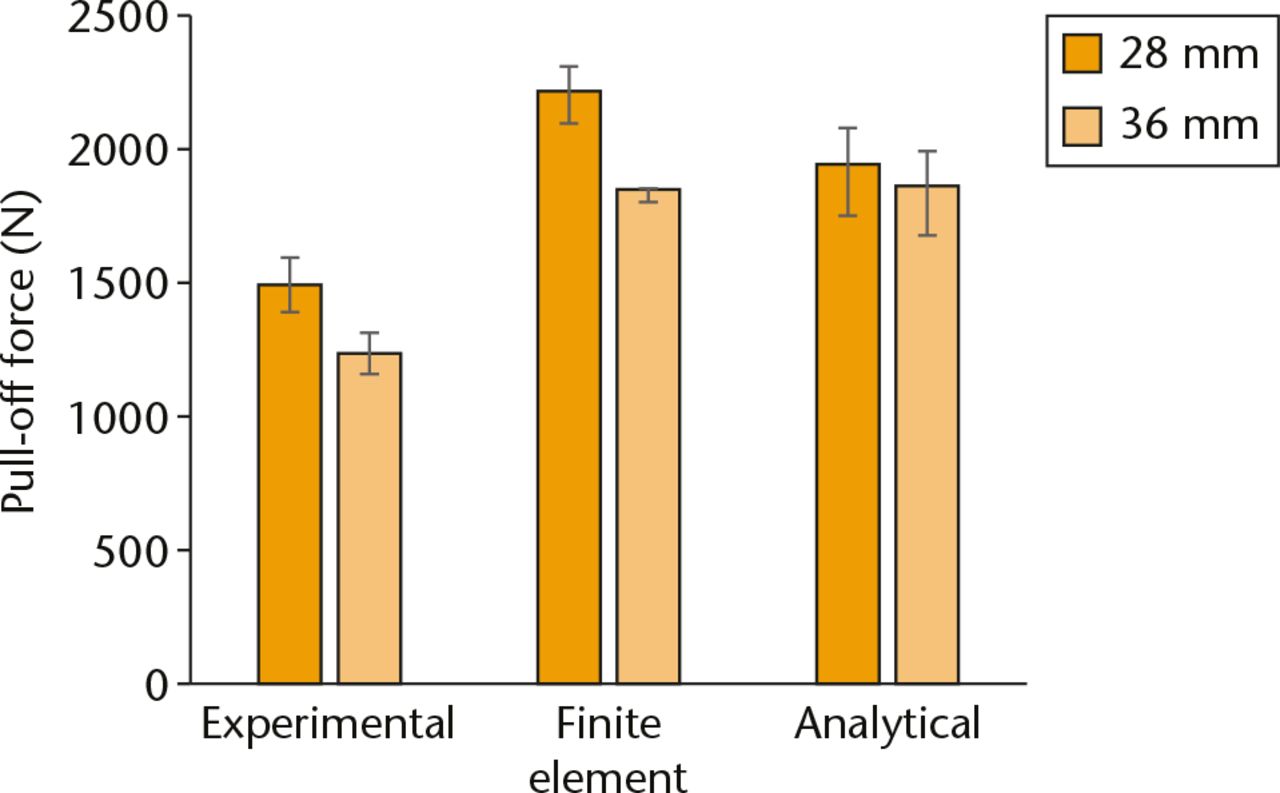
Fig. 4
Experimental and finite element pull-off forces for different head sizes at an impaction force of 4 kN. Error bars shown for the finite element and analytical model are generated by altering the coefficients of friction used.
The predicted stresses arising due to assembly forces were compared for the FE and analytical models (Fig. 5). The difference in agreement in absolute radial stress predictions between the FE and analytical models at the trunnion-head interface was 6.2% and 5.2% for 28 mm and 36 mm heads, respectively (Fig. 5c). Circumferential stress differences were 8.2% and 2.3%, respectively. Despite the similarity in stresses, the differences in pull-off strength between head sizes predicted by the analytical model were much smaller than those predicted by the FE analysis or by the experimental mechanical testing, being 4.9% larger for the 28 mm heads at all load levels (Fig. 4).
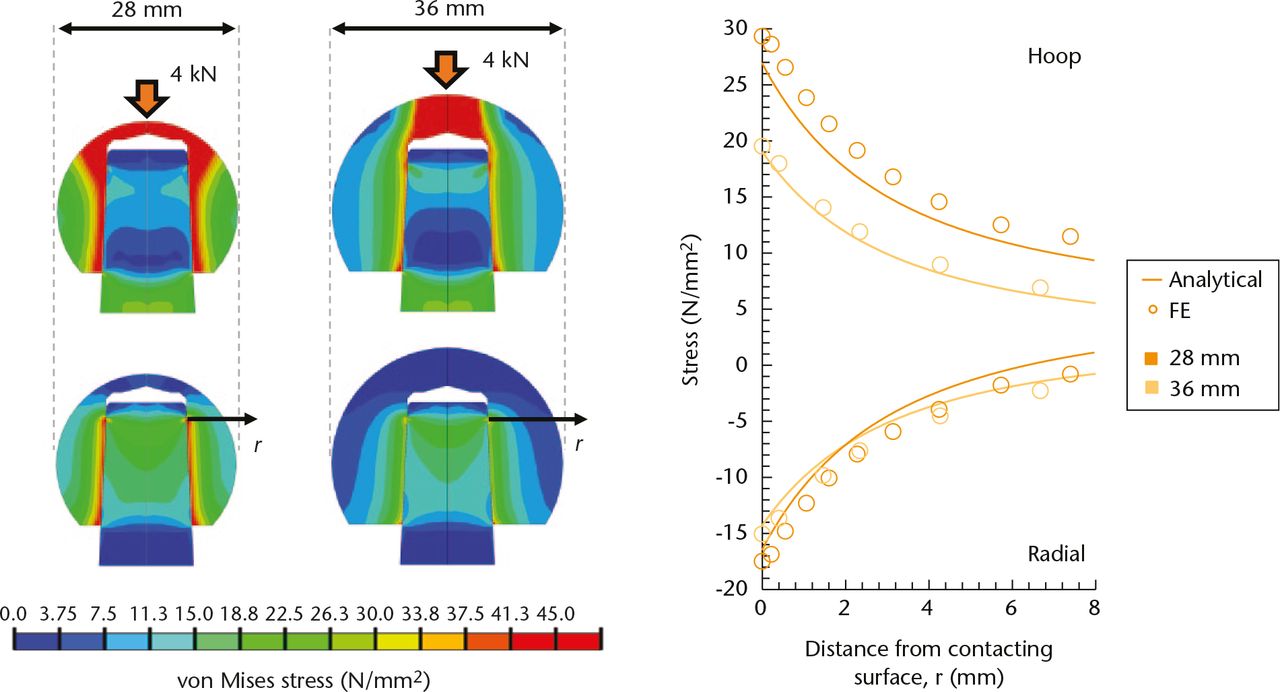
Fig. 5
Von Mises (equivalent) stress predictions of the finite element analysis: a) at an assembly load of 4 kN; b) built-in stresses after assembly; and c) comparison of radial and circumferential stress against the analytical predictions of a cylinder.
The interference rigidity calculated analytically was 3.26 × 103 kN/mm for the 28 mm heads and 3.86 × 103 kN/mm for the 36 mm heads; 18.6% larger for the 36 mm heads.
Discussion
Modular components are assembled intra-operatively by the surgeon; as a result, differences in connection strength obtained can be considerable.28 Pull-off force is generally used as a surrogate measure of in-service connection strength, and factors that are known to influence this variable include impaction force;28,41 the number of impaction strikes;28 taper design41,42 and surface roughness.29,42 It is not clear, however, whether there is any influence of the size of the head on the initial strength of press-fit achieved. The current study evaluated whether head size influences initial pull-off strength for different levels of impaction force.
Assumptions
Taper designs vary between manufacturers, and design differences can produce variation in performance.41 All design variables in the current study other than head size were kept constant; the trunnions used for each head size were identical. As 57% of all THA operations reported by the National Joint Registry (NJR) in 2015 used either 28 mm or 36 mm diameter heads,23 these two head sizes were selected for this study. The Co-Cr-titanium material combination was also selected due to its popularity (61% of THA procedures reported by the NJR).23 Additionally, a previous study found that this material combination produced the largest pull-off resistance.33 Our relatively simple, quasi-static finite element model was able to predict the increase in pull-off strength accurately for the 28 mm heads compared with the 36 mm heads, as shown by the experimental results. This indicates that the phenomenon is not dynamic, affected by the speed of impaction, nor influenced by inertial effects due to the increased mass of the larger-diameter heads. Additionally, quasi-static assembly of components (push-on) has been previously found to produce similar pull-off forces to dynamic assembly.28 Nevertheless, it is possible that dynamic effects could alter the relative difference between head sizes.
Fretting and corrosion are thought to occur due to a combination of rocking and pistoning motions taking place during physiological loading.7 These involve a combination of axial, bending and torsional forces. Previous studies have used pull-off connection strength as an indicator of resistance to loosening and interface micromotion,33,34 however, it has been suggested that the torsional resistance of modular connections may be more important to the life of the connection.38 The two measures are closely related, although the relationship between the torsional connection strength and assembly force is known to be more variable.38
Reports of post-operative complications relating to wear at modular taper junctions are becoming increasingly common.1,8,15 Taper wear appears to be unaffected by bearing surface wear,8 and is present even in ceramic bearings.2,5,21 There is increasing evidence that larger-diameter heads perform poorly with regard to fretting and corrosion at the head-trunnion interface.8,43 The assumption has been that larger-diameter heads, due to their increased lever arm to the centre of rotation, are exposed to larger torques,22 exacerbating the corrosion-fretting process.
Two previous studies have developed non-linear finite element models to understand the influence of various parameters on head-trunnion fretting, using contact stress and micromotion to predict wear rates.7,43 The studies predict that head-centre offset (the distance from the centre of the head to the centre of the stress within the trunnion) is the main factor governing trunnion wear. Interestingly, both studies also found that increased impaction force did not significantly reduce the predicted wear. This was because although contact stresses increased, micromotion between the head and trunnion decreased proportionally, meaning that the combined effect was almost negligible. If this is the case in reality, then larger impaction forces will not be able to compensate for the differences between head sizes in terms of wear rates in the long-term. Unfortunately, neither of these FE studies reported the initial connection strength, and therefore cannot be directly compared with the current study. Additionally, it is not clear whether the wear predictions of these FE studies (using a product of contact stress and micro-motion) are realistic as they do not take into account corrosion-assisted wear which may be more dependent upon connection strength.
The mean pull-off strengths measured in the present study are similar in magnitude to pull-off strengths in previous studies,28,34,41 approximately 37% and 31% of the impaction force for 28 mm and 36 mm heads, respectively.
To our knowledge, this study is the first to demonstrate that given a constant impaction force, the pull-off connection strength of larger-diameter heads is inferior to smaller heads, even prior to physiological loading. We found that the taper connection strength of 36 mm heads was around 20% lower than that of 28 mm heads at 4 kN and 5 kN impaction forces. This study also evaluated whether there were any differences between the head sizes with regard to changes in surface roughness that may have occurred during testing. We found no significant differences in roughness between the head size groups at any load level.
Our experimental, numerical and analytical models were all in agreement that, for 4 kN and 5 kN assembly loads, smaller diameter heads have larger pull-off connection strengths. The extent of the difference shown by our experimental tests was well predicted by our FE simulation with a similar increase in pull-off strength predicted for the 28 mm compared with the 36 mm heads (within 1.1% at 4 kN and 5 kN assembly loads).
The stress predictions of the FE model closely matched the analytical model with a difference of 6.2% for radial stresses at the connection interface. The largest difference between the head sizes was the circumferential stress (hoop stress). The FE and analytical models both predicted a substantially larger hoop stress for the 28 mm heads compared with the 36 mm heads (50% and 41% larger, respectively). The radial stresses for the 28 mm heads were 16% and 15% larger than those of the 36 mm heads for the FE and analytical models, respectively. It is the radial stress that is responsible for the increase in pull-off strength (Equation 11). Using the analytical model, we evaluated the interference rigidity of the two head sizes (force per unit interference at the head-trunnion interface). The interference rigidity of 28 mm heads was 18.6% higher for 36 mm heads, corresponding well with the experimentally measured pull-off force that was 20.7% lower than that of the 36 mm heads. Therefore, much of the difference between pull-off forces for the two head sizes can be explained by interference rigidity. The remainder may be as a result of localised stress concentrations caused by deformation of the trunnion and/or head. These local increases in contact pressure were observed in the FE results and could explain why the FE simulation was able to capture the true extent of the experimentally measured difference, while the analytical model was not. Some of the difference in predictions between the experimental tests and FE simulations may be due to our assumption of perfectly matching taper angles. Slight mismatches could reduce the contact area between the head and trunnion, thereby reducing the connection strength (Equation 11).
Another process that can increase the strength of the taper connection is cold welding due to plastic deformation.5 Witt et al31 demonstrated that plastic deformation can occur at assembly forces as low as 500 N. As our FE model did not include the ridged profile of the trunnion surface, local stress concentrations at the tips of the ridges were not predictable. Nevertheless, our findings suggest that the larger interface pressures generated by 28 mm heads make plastic deformation more likely to occur. This localised effect could alter the tightness of fit or frictional resistance at the contact interface, thereby influencing pull-off strength.5,39 We found no significant differences in roughness between the head size groups at any load level, indicating that the differences in pull-off strength are not due to measurable damage occurring at the trunnion-head interface.
Reduced taper diameters and lengths were introduced to expand the range of motion25 and to create universal tapers within implant ranges that would allow the use of ceramic heads,5 however, elastic deformation of the trunnion has been blamed for increasing micromotion and increasing susceptibility to fretting and corrosion.9,26 Morlock25 hypothesised that three variables could be largely responsible for the dramatic failures of MoM articulations: trunnion diameter, trunnion length and head size. Using Equation 12, a 12 mm diameter trunnion would reduce contact area by 14.3% and pull-off strength by 3.8% compared with an equivalent 14 mm diameter trunnion. Similarly, trunnion length would have a 1:1 influence on contact area and thus influence pull-off strength. Therefore, we have shown that these three variables suspected to influence the long-term survivability - trunnion diameter, trunnion length and head size - also influence the initial connection strength. The clinical importance of these variables is similar, with an approximate 2% change in connection strength per millimetre change in dimension. It should be noted that our study assumed no angular mismatch between the male and female tapers; varying degrees of angular mismatch could therefore produce different results, particularly in the case of trunnion length.
Previous studies have examined the minimum force32,44 or torque34 required to produce a drop in galvanic potential at the head-trunnion interface, thereby exposing it to corrosion. There is agreement that larger connection strengths (resulting from larger assembly forces) require larger forces to initiate corrosion. Therefore, as 28 mm heads have larger connection strengths than 36 mm heads for the same assembly force (up to 5 kN), we infer that they will also have greater corrosion resistance. Further work, however, needs to be undertaken to confirm this.
A possible reason that previous studies have overlooked the influence of assembly effects when using larger-diameter heads is because offset has been used to simulate the effect of larger-diameter heads.7,21 This may not capture the differences in connection strength that occur during assembly.
Limitations
A previous study by Kinbrum, Traynor and Collins37 was also unable to detect changes in roughness before and after testing using impaction forces of 4 kN. Although our assembly forces were larger (up to 6 kN), it is possible that our scanning resolution was not high enough to detect the surface changes. It is not clear why the pull-off force for 28 mm heads at 6 kN impaction force did not follow the linearly increasing trend seen for 36 mm heads. Both FE and analytical models suggest that the trend should continue for much larger impaction forces, however, these models were not equipped to capture any material non-linearity. Although there was no significant change in measured surface roughness of the trunnion, indicating that no damage had occurred, it is possible that damage occurred on the female taper, despite having a larger Young’s modulus, due to the confinement of the male taper. Nevertheless, the typical force applied by surgeons has been shown to be 4409 N (sd 660)28 in laboratory conditions. The lack of constraint of the head-trunnion construct in vivo makes it even less likely that an impaction force of 6 kN is regularly and reliably achieved clinically. It is also important to note that our findings relate to solid THA heads and may not apply to hollowed shell heads such as the Birmingham Hip Modular Head (Smith & Nephew plc, London, United Kingdom), the ASR XL Head (DePuy International, Leeds, United Kingdom), or modular head designs with a titanium sleeve insert.
Despite studies demonstrating that initial connection strength influences corrosion resistance in vitro,32,44 it is still unclear whether this effect influences the long-term survivorship of a hip prosthesis. Further studies should consider measuring connection strength immediately after impaction and after cyclic loading in order to determine whether connection strength is reduced over time due to physiological loading.
In conclusion, the authors note that as far as they are aware, this is the first study to demonstrate that the head-trunnion connection strength of larger-diameter heads is inferior to that of smaller head sizes at the point of assembly. Heads of 36 mm diameter require approximately 20% greater assembly forces (6 kN vs 5 kN) to provide a similar initial pull-off strength to that achieved by 28 mm heads. Any reduction in the initial taper connection strength is likely to compound other effects such as increased centre offset. Although initial stability may not necessarily translate into long-term in vivo stability, the lower connection strength may help to explain the greater extent of fretting and corrosion associated with large diameter heads. If a link between initial and long-term stability is definitively found, these differences would need to be accounted for clinically during THA. It should be noted that this effect is likely to be even more pronounced for head diameters larger than 36 mm.
Funding Statement
JRI donated materials used in the performance of the tests described in the manuscript.
H.S. Gill reports funding received from Smith & Nephew which is related to this article. Professor Gill is a member of the Editorial Board of The Bone & Joint Journal, and a member of Executive Committees of the British and European Orthopaedic Research Societies.
M. Whitehouse reports several fees and grants received from DePuy, Heraeus, Stryker and HQIP, none of which are related to this article.
ICMJE conflict of interest
None declared.
References
1 Esposito CI , WrightTM, GoodmanSB, BerryDJ, Clinical, Biological and Bioengineering Study Groups from Carl T. Brighton Workshop. What is the trouble with trunnions?Clin Orthop Relat Res2014;472:3652-3658.CrossrefPubMed Google Scholar
2 Carli A , PolitisA, ZukorD, HukO, AntoniouJ. Clinically significant corrosion at the head-neck taper interface in total hip arthroplasty: a systematic review and case series. Hip Int2015;25:7-14.CrossrefPubMed Google Scholar
3 Feeley J . J&J to Pay as Much as $420 Million More in ASR Hip Accord. www.bloomberg.com/news/articles/2015-02-23/j-j-to-pay-as-much-as-420-million-more-in-asr-hip-accord (date last accessed 21 July 2016). Google Scholar
4 Lieberman JR , RimnacCM, GarvinKL, KleinRW, SalvatiEA. An analysis of the head-neck taper interface in retrieved hip prostheses. Clin Orthop Relat Res1994;300:162-167.PubMed Google Scholar
5 Hussenbocus S , KosugeD, SolomonLB, HowieDW, OskoueiRH. Head-neck taper corrosion in hip arthroplasty. Biomed Res Int2015;2015:758123.CrossrefPubMed Google Scholar
6 Wassef AJ , SchmalzriedTP. Femoral taperosis: an accident waiting to happen?Bone Joint J2013;95-B(11 Suppl A):3-6.CrossrefPubMed Google Scholar
7 Donaldson FE , CoburnJC, SiegelKL. Total hip arthroplasty head-neck contact mechanics: a stochastic investigation of key parameters. J Biomech2014;47:1634-1641.CrossrefPubMed Google Scholar
8 Langton DJ , SidaginamaleR, LordJK, NargolAV, JoyceTJ. Taper junction failure in large-diameter metal-on-metal bearings. Bone Joint Res2012;1:56-63.CrossrefPubMed Google Scholar
9 Goldberg JR , GilbertJL, JacobsJJ, et al.. A multicenter retrieval study of the taper interfaces of modular hip prostheses. Clin Orthop Relat Res2002;401:149-161.CrossrefPubMed Google Scholar
10 McKellop HA , SarmientoA, BrienW, ParkSH. Interface corrosion of a modular head total hip prosthesis. J Arthroplasty1992;7:291-294.CrossrefPubMed Google Scholar
11 Purdue PE , KoulouvarisP, NestorBJ, SculcoTP. The central role of wear debris in periprosthetic osteolysis. HSS J2006;2:102-113.CrossrefPubMed Google Scholar
12 Del Balso C , TeeterMG, TanSC, LantingBAHJ, HowardJL. Taperosis: does head length affect fretting and corrosion in total hip arthroplasty?Bone Joint J2015;97-B:911-916.CrossrefPubMed Google Scholar
13 Whitehouse MR , EndoM, ZacharaS, et al.. Adverse local tissue reactions in metal-on-polyethylene total hip arthroplasty due to trunnion corrosion. Bone Joint J2015;97-B:1024-1030.CrossrefPubMed Google Scholar
14 Langton DJ , JamesonSS, JoyceTJ, et al.. Early failure of metal-on-metal bearings in hip resurfacing and large-diameter total hip replacement: A consequence of excess wear. J Bone Joint Surg [Br]2010;92-B:38-46.CrossrefPubMed Google Scholar
15 Smith AJ , DieppeP, VernonK, et al.. Failure rates of stemmed metal-on-metal hip replacements: analysis of data from the National Joint Registry of England and Wales. Lancet2012;379:1199-1204.CrossrefPubMed Google Scholar
16 Cross MB , NamD, MaymanDJ. Ideal femoral head size in total hip arthroplasty balances stability and volumetric wear. HSS J2012;8:270-274.CrossrefPubMed Google Scholar
17 Morlock MM , BishopN, PerkaC. Is bigger really better?Orthopaedic Proceedings2012; 94-B(SUPP XL):115. Google Scholar
18 Cooper HJ , Della ValleCJ. Large diameter femoral heads: is bigger always better?Bone Joint J2014;96-B(Suppl A):23-26.CrossrefPubMed Google Scholar
19 Matthies AK , RacasanR, BillsP, et al.. Material loss at the taper junction of retrieved large head metal-on-metal total hip replacements. J Orthop Res2013;31:1677-1685.CrossrefPubMed Google Scholar
20 Nassif NA , NawabiDH, StonerK, et al.. Taper design affects failure of large-head metal-on-metal total hip replacements. Clin Orthop Relat Res2014;472:564-571.CrossrefPubMed Google Scholar
21 Panagiotidou A , MeswaniaJ, OsmanK, et al.. The effect of frictional torque and bending moment on corrosion at the taper interface: an in vitro study. Bone Joint J2015;97-B:463-472. Google Scholar
22 No authors listed. Scientific Committee on Emerging and Newly Identified Health Risks (SCENIHR). Opinion on: The safety of Metal-on-Metal joint replacements with a particular focus on hip implants. European Commission. http://ec.europa.eu/health/scientific_committees/emerging/docs/scenihr_o_042.pdf (date last accessed 17 May 2016). Google Scholar
23 No authors listed. National Joint Registry for England, Wales, Northern Ireland and the Isle of Man. 12th Annual Report. http://www.njrcentre.org.uk/njrcentre/Portals/0/Documents/England/Reports/12th%20annual%20report/NJR%20Online%20Annual%20Report%202015.pdf (date last accessed 17 May 2016). Google Scholar
24 Hexter A , PanagiotidouA, SinghJ, SkinnerJ, HartA. Corrosion at the head-trunnion taper interface in large diameter head metal-on-metal total hip arthroplasty: a comparison of five manufacturers. Bone Joint J2013;95-B(Supp 12):3. Google Scholar
25 Morlock MM . The taper disaster–how could it happen?Hip Int2015;25:339-346. Google Scholar
26 Tan SC , TeeterMG, Del BalsoC, HowardJL, LantingBA. Effect of taper design on trunnionosis in metal on polyethylene total hip arthroplasty. J Arthroplasty2015;30:1269-1272.CrossrefPubMed Google Scholar
27 Dyrkacz RMR , BrandtJM, OjoOA, TurgeonTR, WyssUP. The influence of head size on corrosion and fretting behaviour at the head-neck interface of artificial hip joints. J Arthroplasty2013;28:1036-1040.CrossrefPubMed Google Scholar
28 Heiney JP , BattulaS, VrabecGA, et al.. Impact magnitudes applied by surgeons and their importance when applying the femoral head onto the Morse taper for total hip arthroplasty. Arch Orthop Trauma Surg2009;129:793-796.CrossrefPubMed Google Scholar
29 Jauch SY , MilesAW, GillHS. Does the Surface Finish, the Taper Angle Difference and the Assembly Force Effect the Taper Strength Between Stem and Ball Head of a Modular Hip Implant?ORS Meeting, 2014http://www.ors.org/Transactions/60/1785.pdf (date last accessed 19 May 2016). Google Scholar
30 Jauch SY , HuberG, HoenigE, et al.. Influence of material coupling and assembly condition on the magnitude of micromotion at the stem-neck interface of a modular hip endoprosthesis. J Biomech2011;44:1747-1751.CrossrefPubMed Google Scholar
31 Witt F , GührsJ, MorlockMM, BishopNE. Quantification of the contact area at the head-stem taper interface of modular hip prostheses. PLoS One2015;10:e0135517.CrossrefPubMed Google Scholar
32 Mroczkowski ML , HertzlerJS, HumphreySM, JohnsonT, BlanchardCR. Effect of impact assembly on the fretting corrosion of modular hip tapers. J Orthop Res2006;24:271-279.CrossrefPubMed Google Scholar
33 Rehmer A , BishopNE, MorlockMM. Influence of assembly procedure and material combination on the strength of the taper connection at the head-neck junction of modular hip endoprostheses. Clin Biomech (Bristol, Avon)2012;27:77-83.CrossrefPubMed Google Scholar
34 Jauch SY , ColesLG, NgLV, MilesAW, GillHS. Low torque levels can initiate a removal of the passivation layer and cause fretting in modular hip stems. Med Eng Phys2014;36:1140-1146.CrossrefPubMed Google Scholar
35 No authors listed. ISO 7206-10:2003 Implants for surgery – Partial and total hip-joint prostheses – Part 10: Determination of resistance to static load of modular femoral heads. http://www.iso.org/iso/catalogue_detail.htm?csnumber=31211 (date last accessed 21 July 2016). Google Scholar
36 Swaminathan V , GilbertJL. Fretting corrosion of CoCrMo and Ti6Al4V interfaces. Biomaterials2012;33:5487-5503.CrossrefPubMed Google Scholar
37 Kinbrum A , TraynorA, CollinsS. Mathematical calculation to quantify pull-off strength relationship to push-on strength. Orthopaedic Proceedings2014;96-B(SUPPL 11 81). Google Scholar
38 Macdonald W , AspenbergA, JacobssonCM, CarlssonLV. Friction in orthopaedic zirconia taper assemblies. Proc Inst Mech Eng H2000;214:685-692.CrossrefPubMed Google Scholar
39 Fessler H , FrickerDC. Friction in femoral prosthesis and photoelastic model cone taper joints. Proc Inst Mech Eng H1989;203:1-14.CrossrefPubMed Google Scholar
40 Bower AF . Applied Mechanics of Solids. Boca Raton: CRC Press, 2010. Google Scholar
41 Pennock AT , SchmidtAH, BourgeaultCA. Morse-type tapers: factors that may influence taper strength during total hip arthroplasty. J Arthroplasty2002;17:773-778.CrossrefPubMed Google Scholar
42 Brock TM , SidaginamaleR, RushtonS, et al.. Shorter, rough trunnion surfaces are associated with higher taper wear rates than longer, smooth trunnion surfaces in a contemporary large head metal-on-metal total hip arthroplasty system. J Orthop Res2015;33:1868-1874.CrossrefPubMed Google Scholar
43 Elkins JM , CallaghanJJ, BrownTD. Stability and trunnion wear potential in large-diameter metal-on-metal total hips: a finite element analysis. Clin Orthop Relat Res2014;472:529-542.CrossrefPubMed Google Scholar
44 Goldberg JR , GilbertJL. In vitro corrosion testing of modular hip tapers. J Biomed Mater Res B Appl Biomater2003;64:78-93.CrossrefPubMed Google Scholar