Abstract
Objectives
There remains conflicting evidence regarding cortical bone strength following bisphosphonate therapy. As part of a study to assess the effects of bisphosphonate treatment on the healing of rat tibial fractures, the mechanical properties and radiological density of the uninjured contralateral tibia was assessed.
Methods
Skeletally mature aged rats were used. A total of 14 rats received 1µg/kg ibandronate (iban) daily and 17 rats received 1 ml 0.9% sodium chloride (control) daily. Stress at failure and toughness of the tibial diaphysis were calculated following four-point bending tests.
Results
Uninjured cortical bone in the iban group had a significantly greater mean (standard deviation (sd)), p < 0.001, stress at failure of 219.2 MPa (sd 45.99) compared with the control group (169.46 MPa (sd 43.32)) following only nine weeks of therapy. Despite this, the cortical bone toughness and work to failure was similar. There was no significant difference in radiological density or physical dimensions of the cortical bone.
Conclusions
Iban therapy increases the stress at failure of uninjured cortical bone. This has relevance when normalising the strength of repair in a limb when comparing it with the unfractured limb. However, the 20% increase in stress at failure with iban therapy needs to be interpreted with caution as there was no corresponding increase in toughness or work to failure. Further research is required in this area, especially with the increasing clinical burden of low-energy diaphyseal femoral fractures following prolonged use of bisphosphonates.
Cite this article: Bone Joint Res 2015;4:99–104
Article focus
To assess the effect of bisphosphonates on the mechanical properties and radiological density of cortical bone.
Key messages
Bisphosphonate increases the stress at failure of cortical bone by up to 20% following nine weeks of therapy.
This increase in stress at failure should be interpreted with caution as there was no corresponding increase in bone toughness and work to failure.
The effect of prolonged bisphosphonate treatment on cortical bone needs further study.
Strengths and limitations
This is the first study to assess the effects of bisphosphonates on cortical bone which has used aged skeletally mature animals. This is more representative of the situation in routine clinical practice when bisphosphonates are prescribed for the prevention of fragility fractures in the elderly population.
This study showed that the use of bisphosphonates resulted in an increase in the stress at failure in the unfractured limb, however, as this was performed as a single test to failure, the effect on the fatigue properties of the bone were not assessed.
A potential source of error could arise from the derivation of the second moment of area. In this study, as the cross section was assumed to be a triangle, and measurement resolution was to ± 0.01 mm. μCT, scanning of the limb could be used to determine the exact shape and dimensions.
Introduction
The effect of bisphosphonates on bone is primarily via an anti-catabolic effect owing to inhibition of osteoclast mediated bone resorption. The effect of bisphosphonates on the mechanical properties of cortical bone remain poorly reported. Animal trials in rodents, canines and primates have consistently shown that bisphosphonates increase the compressive load at failure of cancellous bone within the vertebra1-6 and femoral neck.1,2 Prospective human studies7-9 have shown that bisphosphonates prevent loss of bone mineral density (BMD) and decrease the risk of fracture, particularly in cancellous bone at the vertebral column and femoral neck. Recent randomised double blind placebo controlled clinical studies10,11 have even suggested an actual increase in BMD at these sites with oral bisphosphonate taken once a month. However, laboratory studies that demonstrate preservation of BMD and an increase in cancellous bone compressive strength with bisphosphonate therapy have not shown consistent effects on cortical bending strength.1,12
The combination of findings in clinical trials and animal data, in particular the demonstrable increase in strength of cancellous bone and reduction in the risk of fracture, has led to the widespread use of bisphosphonates in the prevention of fractures.
The effect of bisphosphonate on the mechanical properties of cortical bone is of particular importance as cortical bone remodels less quickly than cancellous bone. The bisphosphonate inhibition of remodelling,13 with a resultant accumulation of ‘micro-damage’,14 may manifest clinically as cortical insufficiency fractures.13,15,16
The aim of this study was to identify the effect of bisphosphonate administration on the mechanical properties and radiological density of cortical bone. Ibandronate (iban) was administered at a therapeutic dose known to prevent post-ovariectomy loss in BMD.17 Stress at failure, work to failure, toughness of bone, physical measurements of bone and radiological density of the tibial diaphysis were assessed.
Materials and Methods
All animal procedures were approved by the Home Office of the United Kingdom, and adhered to the Animals (Scientific Procedures) Act 1986. In total, 31 skeletally mature male ex-breeder Sprague-Dawley rats (Harlan, United Kingdom) that were all aged > one year were included in this randomised study, with the sample size based on a power calculation for a fracture healing study. The rats were fed standard rat chow and provided with water ad libitum. They were housed in individual cages at a constant temperature (21˚C; ± 1˚C) and humidity (45% to 55%) using a 12-hour light/dark cycle. Animals received three weeks of daily subcutaneous injections prior to the creation of a standardised right tibial osteotomy and operative stabilisation with a plate and two bicortical screws on either side as part of a fracture healing study.18 All animals were noted to mobilise freely with no restrictions within 24 hours post-surgery. In total, 14 animals received 1 μg/kg iban daily and the remaining 17 received daily subcutaneous injections of 0.9% saline, which acted as the control. The mean weight of iban and control rats was 491.93 g (sd 55.4) and 508.12 g (sd 41.9), respectively. Six weeks post-osteotomy, animals were sacrificed and the left uninjured tibiae were carefully dissected free of soft tissue for use in this study.
Plain radiographs were obtained using a portable radiograph unit (Acu-Ray JR, Stern Manufacturing, Toronto, Canada) with an output of 60 kV, 2 mAs and exposure time of 0.1 ms. Images were captured on digital radiograph plates (Fuji CR Cassette, Fuji Photo Film Co Ltd, Japan). Lateral contact radiographs were obtained to exclude the effect of fibula overlay in radiological analysis of bone density. An aluminium step wedge consisting of ten 1 mm steps was placed near the area of interest in each radiograph. Subsequent image analysis was performed in MATLAB (The MathWorks, Inc., Massachusetts). For each rat, the point where the fibula joins the tibia distally was marked. A 100 × 50 pixel box was marked from this point to include the mid shaft of the bone. To assess bone density, an average grey level of the diaphyseal tibia within this box was measured. This measured grey level was then converted to an aluminium equivalent (Al.Eq) density using a previously described method.19
Following plain radiographs, the samples were placed in 0.9% saline within individual tubes and frozen at -20˚C prior to mechanical testing which took place within two weeks.20 Mechanical testing to failure with a four-point bend was performed using a rig (Fig. 1) designed for rat tibias (The University of Edinburgh Physics Workshop, Edinburgh, United Kingdom) in conjunction with a Zwick/Roell Z005 (Zwick GmbH & Co, Ulm, Germany) materials testing machine. The upper loading rig was mounted on a pivot to ensure symmetrical loading of the irregularly shaped rat tibias at all four loading points. The upper loading span was spaced 10 mm apart and the lower static span was spaced 20 mm apart. Contact points were rounded to minimise notching of the bone after load application. Specimens were warmed in a water bath set at 37˚C for a minimum of 30 minutes prior to mechanical testing. Immediately following removal from the water bath, the tibias were mounted on the rig resting on the lateral surface and a four-point bend applied while the bone was well hydrated. The bones were observed during loading and no rotation of the specimen was observed. A pre-load of 1 N was applied to the bone before loading to failure at a rate of 10 Ns-1 following the protocol set out in another study,18 and a 5 kN load cell was used. A load-deflection curve was obtained for each specimen during mechanical testing. The area under this curve, up to the point of failure, was derived to quantify work to failure.
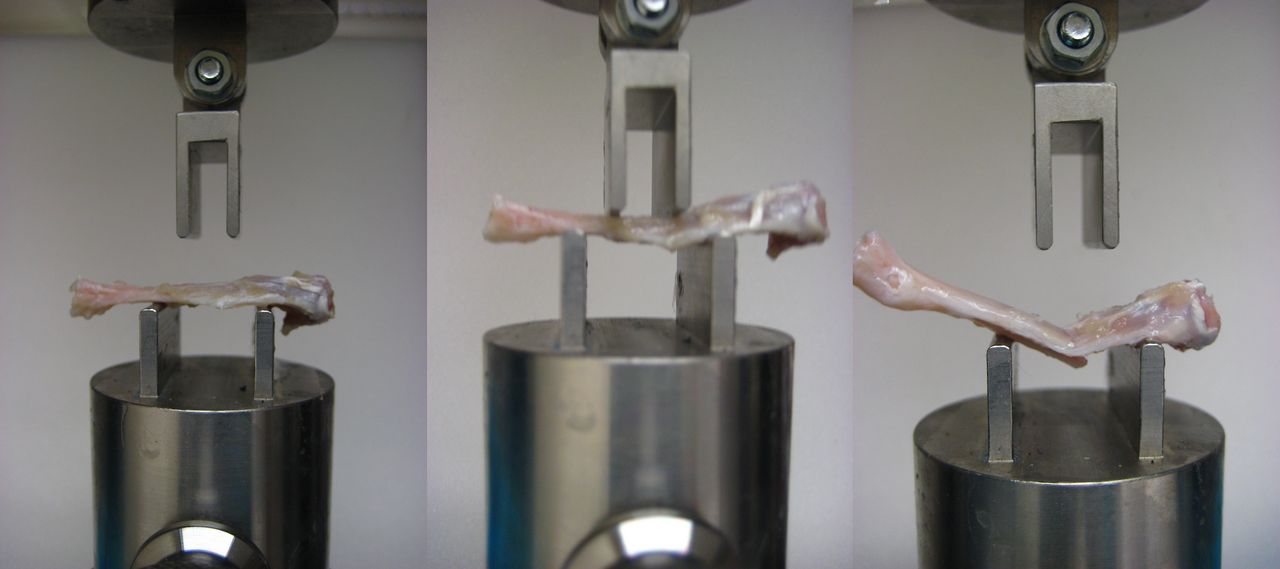
Fig. 1
Photographs of four-point bending of the rat tibia.
Following failure, the tibial midshaft cross section most closely resembled a triangle (Fig. 2). The sagittal and coronal widths which corresponded to the vertical height (H) and base length (B) of the triangular cross section were obtained using sliding callipers. A digital image (Canon Digital IXUS 75 camera) of the distal segment cross section for each sample was obtained. These images were printed and the vertical height and base length of the triangular cross section for the whole bone and the intramedullary canal were measured manually. The scale for the digital image was obtained by comparing the external widths previously measured with the callipers to the readings obtained from the image. The internal vertical height (h) and base length (b) of the triangular intramedullary cross section were then calculated. The cross-sectional moment of inertia (INA) was then derived using equation 1 (Fig. 3) was then derived using equation 1 (Fig. 3), in order to facilitate the calculation of the stress because of bending (σB), according to elastic beam bending theory (equation 2, Fig. 4).
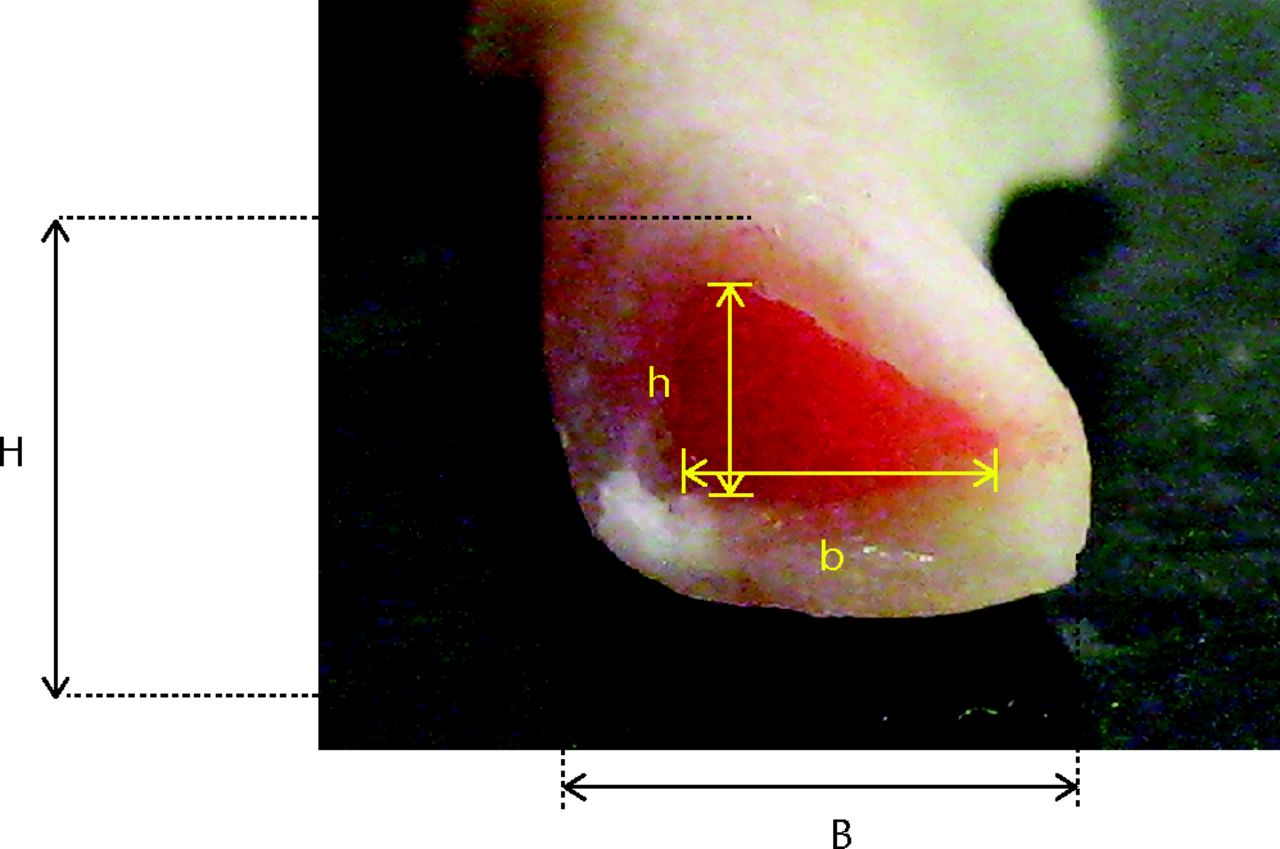
Fig. 2
Photograph of triangular cross section of the tibia. (H, vertical height; B, base length; h, internal vertical height; b, internal base length.)
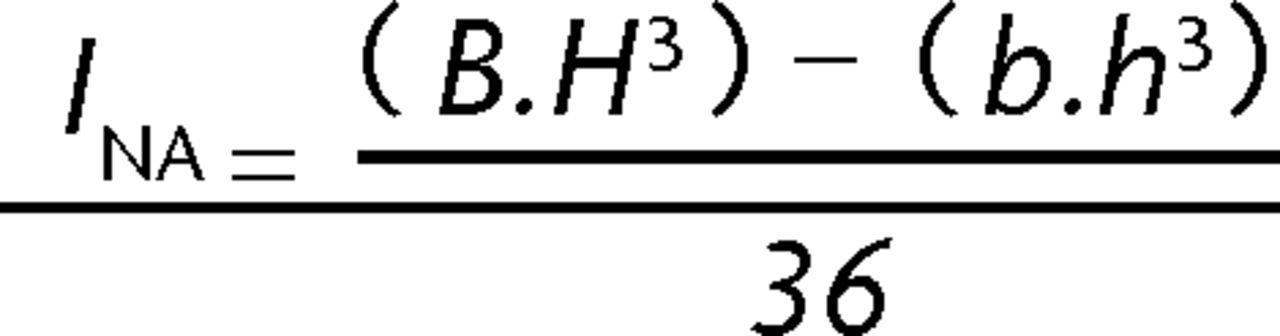
Fig. 3
Equation 1
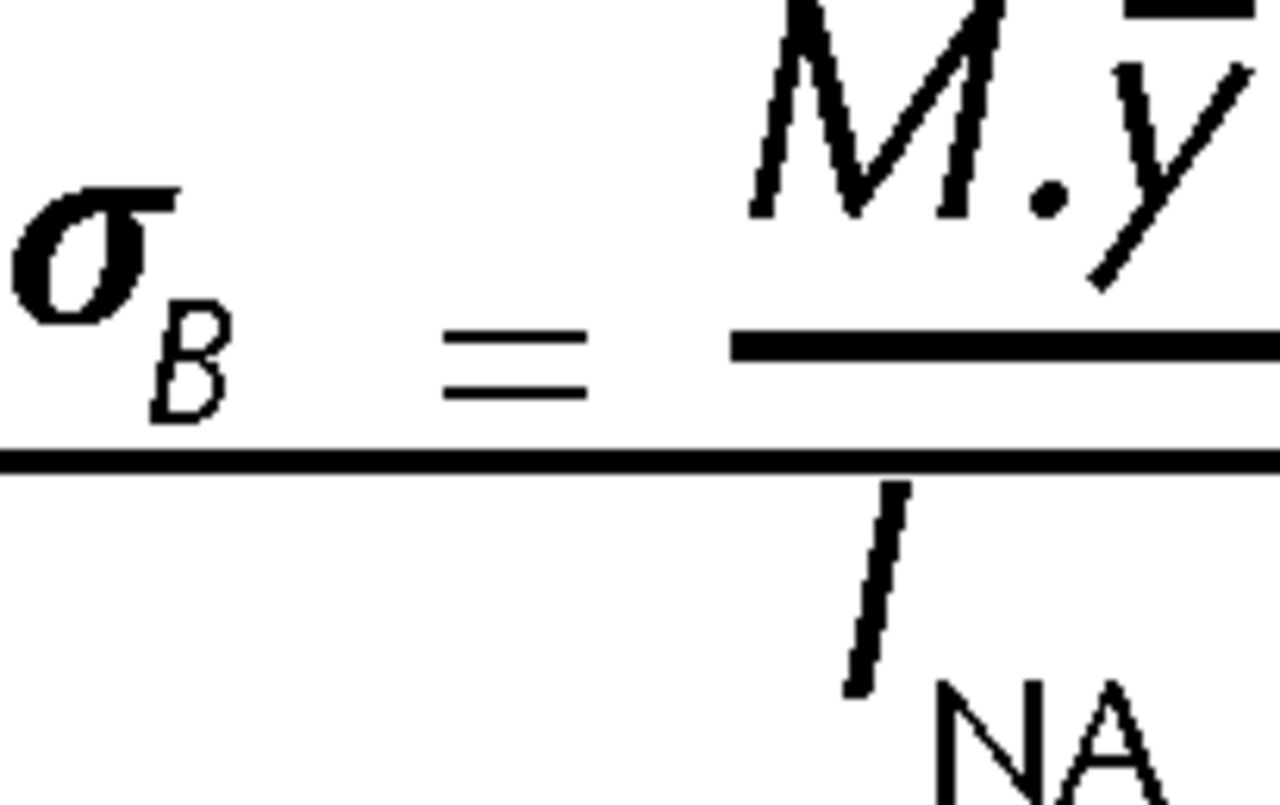
Fig. 4
Equation 2
Theoretical strain was calculated with measurements gathered during the four-point bending test as previously described.21 A stress–strain curve was plotted and the area under this curve to the point of fracture was measured to assess toughness as previously described.22 Two animals in the IBAN group were excluded from analysis as failure occurred in a mode not consistent with bending stress.
Statistical analysis
This was performed using Minitab 16 (Minitab Inc, State College, Pennsylvania). The variables were tested for normality using a Ryan–Joiner test. Peak stress, toughness, physical measurements of cortical bone and Al.Eq density were found to be normally distributed and were analysed using a one-way analysis of variance. Both work to failure and deflection were found to be not normally distributed and were analysed using a Kruskal–Wallis test for non-parametric data.
Results
Following nine weeks of treatment, prior to sacrifice, the mean (sd) weight of iban and control rats was 504 g (sd 69.15) and 505 g (sd 37.72), respectively (p = 0.53). Stress at failure, toughness, physical measurements of coronal and sagittal width and Al.Eq density measurements for each animal are presented in Table I.
Table I
Mechanical properties, physical dimensions and aluminium equivalent (Al.Eq) measurements of density for each animal
Property | Mean iban (sd) | Mean control (sd) | p-value |
---|---|---|---|
Stress (MPa) | 219.2 (46.0) | 169.5 (43.3) | 0.006 |
Work (mJ) | 77.2 (50.5) | 52.9 (23.9) | 0.094 |
Toughness (J/m3) | 2.51 (1.74) | 1.66 (0.86) | 0.093 |
Deflection (mm) | 0.804 (0.480) | 0.549 (0.274) | 0.081 |
Coronal width (mm) | 3.70 (0.82) | 3.83 (0.61) | 0.627 |
Sagittal width (mm) | 2.87 (0.23) | 3.03 (0.22) | 0.066 |
Al.Eq density (mm) | 1.53 (0.27) | 1.75 (0.36) | 0.074 |
-
Iban, ibandronate;sd, standard deviation
The iban group had a statistically significant (p = 0.006) higher stress at failure (sd) 219.2 MPa (sd 45.99) compared with the control group (169.5 MPa, sd 43.32) (Fig. 5) (Fig. 5). There was a trend towards a greater toughness in the iban group but this did not reach statistical significance (p = 0.093) (iban vs control = 2.51 MJ/mm3, sd 1.74 vs 1.67 MJ/mm3, sd 0.86). Similarly, there was a greater, but not statistically significant (p = 0.094), mean work to failure in the iban group compared with controls. The deformation to the point of fracture was greater in the iban group, but not significantly different (p = 0.081) (iban vs control = 0.68 mm, sd 0.23; vs 0.55 mm, sd 0.27).
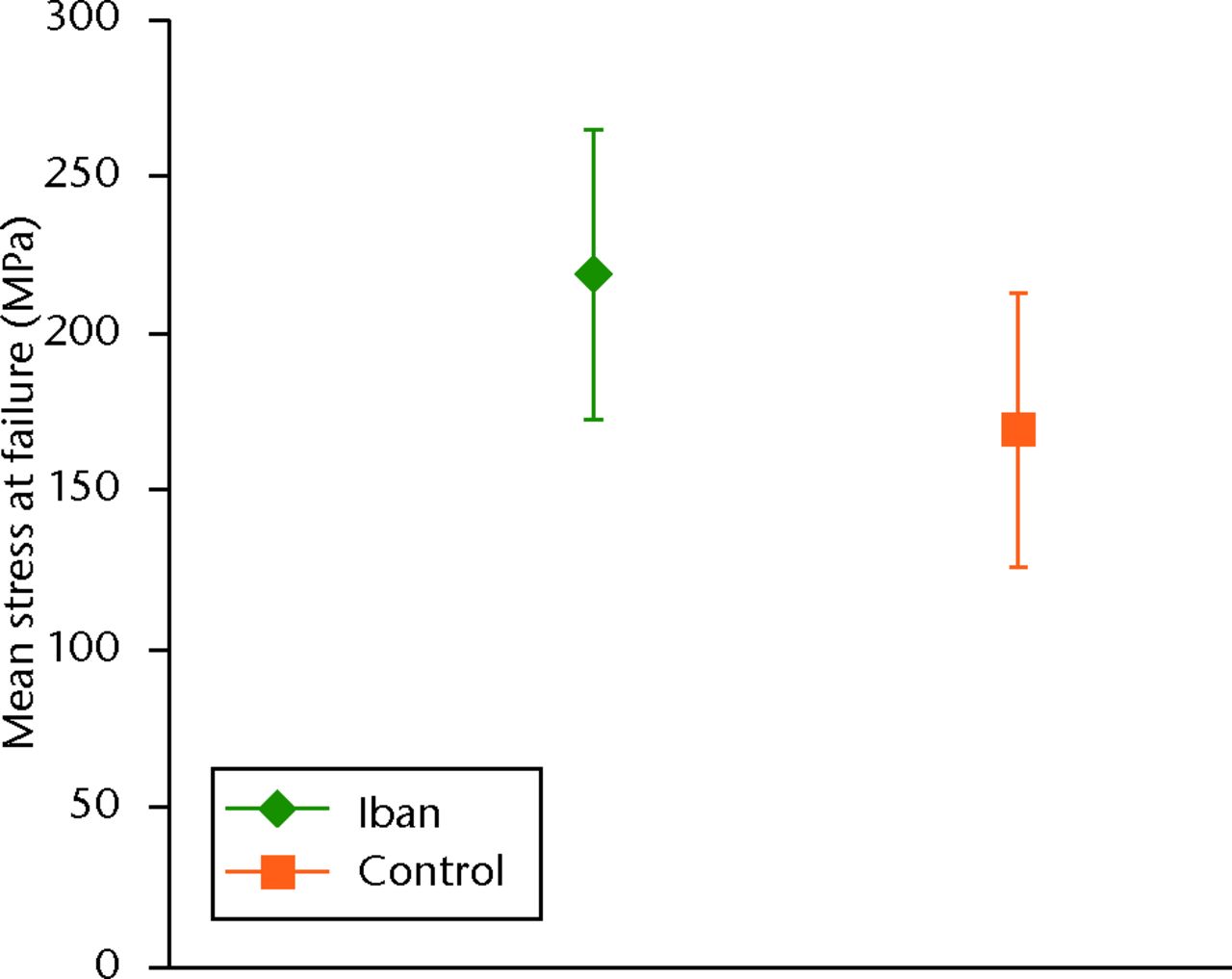
Fig. 5
Graph showing the stress at failure of rats treated with ibandronate (iban) versus control.
There was no statistically significant difference in the physical measurements of bone width. The mean (sd) coronal and sagittal cortical bone widths for the iban versus control groups were 3.7 mm (sd 0.83) versus 3.8 mm (sd 0.62) and 2.7 mm (sd 0.24) versus 3.0 mm (sd 0.21), respectively. There was no difference in the cross sectional moment of inertia between the groups (iban vs control = 0.97 mm4, sd 0.07 vs 1.01 mm4, sd 0.08).
There was no statistically significant difference in the mean (sd) Al.Eq density at the tibial diaphysis (iban vs control = 1.53, sd 0.24 vs 1.76, sd 0.33). A scatter plot of the relationship between Al.Eq density and stress at failure is presented in Figure 6.
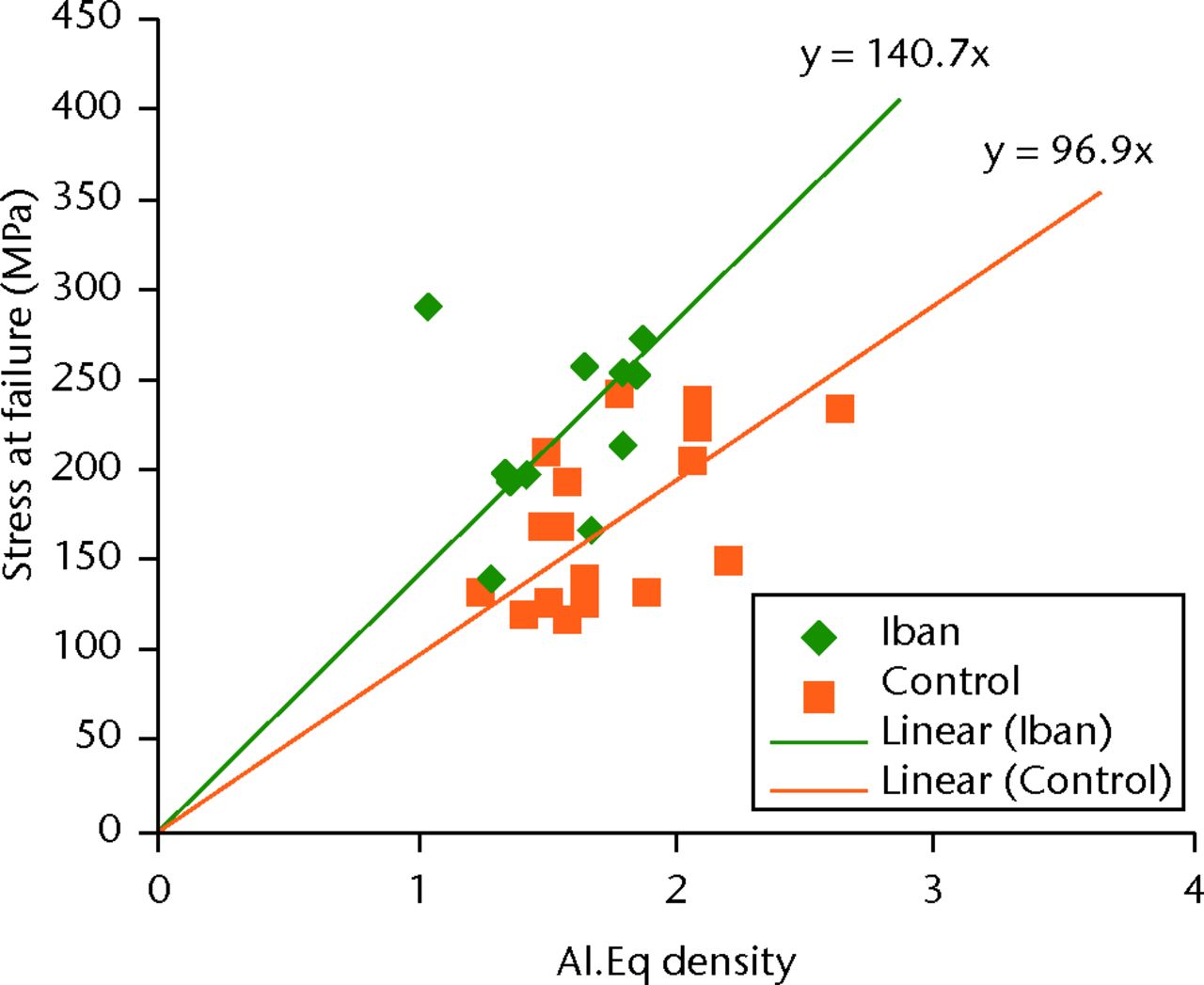
Fig. 6
Graph showing scatter plot of stress at failure versus density of the aluminium equivalent (Al.Eq; Iban, ibandronate).
Discussion
There is conflicting evidence regarding the effect of bisphosphonates on the mechanical properties of cortical bone. In growing rats, both a short bisphosphonate period of treatment of three weeks with pamidronate23 and a longer duration of up to two years with alendronate3 have been shown to increase femoral cortical strength in three-point bending stress tests compared with healthy controls. In ovariectomised rats, three months of clodronate24 and one year of zoledronic acid25 increased femoral24,25 and tibial24 cortical bending strength in comparison with sham-ovariectomised controls. However, six months of alendronate therapy in ovariectomised rats did not affect femoral diaphyseal strength in bending tests, despite the increased mechanical strength noted in cancellous bone tested in compression.2 Similarly, six months of alendronate26 in castrated male rats did not increase bending strength of the femur diaphysis, despite the improved strength noted previously in ovariectomised rats that received a similar dosing regimen.3
The differing results may be because previous studies in rodents have used ovariectomised2,24,25 skeletally immature models3,23,26 or castrated skeletally immature animals26 whereby a sufficient period of time27,28 between castration and the experimental period was not observed to permit development of osteopaenia post castration. The effect of bisphosphonate on growing bone is unlikely to resemble the clinical scenario of bisphosphonate treatment for fragility fracture prevention in the elderly. In the rodent, ovariectomy has been shown to increase bone formation in tibial cortical bone29 and results in elevated tibial bending strength up to six months post-ovariectomy.24 Therefore, an ovariectomised model was not used as it may have confounded our results.
To our knowledge, this is the first study to use aged ex-breeder animals which is more representative of the situation in routine clinical practice when bisphosphonates are prescribed for the prevention of fragility fractures in the elderly population. Skeletally mature animals were also used to eliminate possible differing effects of bisphosphonate on growing bone. In all animals used within this study, both the proximal and distal tibial epiphyses had fused.
Contrary to the often stated opinion that bone remodelling does not occur in the rat,30,31 Bentolila et al32 observed bone remodelling following fatigue loading of rodent ulnas. After ten days of axial loading, there was a strong association between linear ‘microcracks’ and osteoclastic-led tunnelling units. In addition, 40% fewer ‘microcracks’ were observed compared with the similarly loaded contralateral ulna immediately before the rodents were killed. This suggested that the ‘microcracks’ were being repaired by a process of bone remodelling as seen with the Haversian technique.
It is known that following a fracture, osteopaenia is detectable as a decrease in bone mineral density (BMD) in the contralateral uninjured limb.33 Our results indicate that iban at a therapeutic dose17 may increase stress of cortical bone at failure despite the expected fracture-induced osteopaenia. Our finding differs to that previously reported in a beagle dog model of long bone fracture, where alendronate therapy at a high non-therapeutic dose did not affect the rate of stress at failure either of the healing fractured radius or the contralateral radial diaphysis.34
Our results suggest that within the total of nine weeks of iban therapy, the mean stress at failure of cortical bone is increased by greater than 20%. There was also no difference in the cortical bone physical dimensions. It may be that the regions of remodelling bone that are undergoing resorption by osteoclasts do not contribute to the mechanical strength of the bone. Therefore, the rapid increase in bone strength with bisphosphonate treatment could be accounted for by these regions that are now able to contribute to the mechanical strength of the bone as they are no longer resorbed by osteoclasts.
A previous study using cortical femoral specimens from human donors reported a linear relationship between the decrease in bone strength and toughness with ageing.35 Therefore, the authors concluded that with respect to age-related change in bone material properties, the relationship between bone strength and toughness is linear, and thus similar to that seen in synthetic fibre composites.36 We found that despite an increase in bone strength of more than 20% following nine weeks of bisphosphonate therapy, the toughness of bone and work to failure did not change significantly, suggesting that the energy absorbed per unit of bone prior to failure changed less than the absolute strength. This increase in strength, but not in toughness, may indicate that for patients on bisphosphonates, hip protection devices should focus on reducing the energy transmitted to the bone in order to provide the maximum synergistic benefit in fracture reduction.
Despite the significant positive effect of iban therapy on the stress at failure of rat tibia cortical bone in bending tests, no statistically significant effect was noted in the radiological assessment of bone density. The mechanical properties may be altered prior to detectable radiological change or the method used in this study was not able to detect subtle changes in radiological density, especially in that of small bones. Perhaps the use of micro-computed tomography analysis may have detected a less obvious difference. Whatever the cause for the increase in strength, the observed effect of a bisphosphonate on the mechanical properties of the uninjured limb needs to be considered when reporting proportional strength of fracture repair compared with the unfractured limb, as it is often used in fracture healing studies.
This study demonstrates that iban therapy increases the stress at failure of cortical bone in the uninjured limb in the early phase following a fracture. It is increasingly evident that a large proportion of osteoporotic fractures occur at non-vertebral sites of predominant cortical bone and is due to the significant cortical bone loss that occurs in osteoporosis.37 The observed increase in stress at failure of cortical bone with iban treatment is encouraging. However, the lack of a similar beneficial effect on toughness of bone and work to failure should be borne in mind. The effect of prolonged bisphosphonate treatment on cortical bone, especially in the longer term, needs to be studied further, especially in view of reports of low-energy subtrochanteric femoral fractures following continuous long-term use of bisphosphonates.13,15,16
1 Smith SY , ReckerRR, HannanM, MüllerR, BaussF. Intermittent intravenous administration of the bisphosphonate ibandronate prevents bone loss and maintains bone strength and quality in ovariectomized cynomolgus monkeys. Bone2003;32:45–55.CrossrefPubMed Google Scholar
2 Toolan BC , SheaM, MyersER, et al.Effects of 4-amino-1-hydroxybutylidene bisphosphonate on bone biomechanics in rats. J Bone Miner Res1992;7:1399–1406.CrossrefPubMed Google Scholar
3 Guy JA , SheaM, PeterCP, MorrisseyR, HayesWC. Continuous alendronate treatment throughout growth, maturation, and aging in the rat results in increases in bone mass and mechanical properties. Calcif Tissue Int1993;53:283–288.CrossrefPubMed Google Scholar
4 Lalla S , HothornLA, HaagN, BaderR, BaussF. Lifelong administration of high doses of ibandronate increases bone mass and maintains bone quality of lumbar vertebrae in rats. Osteoporos Int1998;8:97–103.CrossrefPubMed Google Scholar
5 Müller R , HannanM, SmithSY, BaussF. Intermittent ibandronate preserves bone quality and bone strength in the lumbar spine after 16 months of treatment in the ovariectomized cynomolgus monkey. J Bone Miner Res2004;19:1787–1796.CrossrefPubMed Google Scholar
6 Yoshida Y , MoriyaA, KitamuraK, et al.Responses of trabecular and cortical bone turnover and bone mass and strength to bisphosphonate YH529 in ovariohysterectomized beagles with calcium restriction. J Bone Miner Res1998;13:1011–1022.CrossrefPubMed Google Scholar
7 Black DM , CummingsSR, KarpfDB, et al.Randomised trial of effect of alendronate on risk of fracture in women with existing vertebral fractures. Fracture Intervention Trial Research Group. Lancet1996;348:1535–1541.CrossrefPubMed Google Scholar
8 Bone HG , HoskingD, DevogelaerJP, et al.Ten years’ experience with alendronate for osteoporosis in postmenopausal women. N Engl J Med2004;350:1189–1199. Google Scholar
9 Chesnut CH III , SkagA, ChristiansenC, et al.Effects of oral ibandronate administered daily or intermittently on fracture risk in postmenopausal osteoporosis. J Bone Miner Res2004;19:1241–1249.CrossrefPubMed Google Scholar
10 Orwoll ES , BinkleyNC, LewieckiEM, et al.Efficacy and safety of monthly ibandronate in men with low bone density. Bone2010;46:970–976.CrossrefPubMed Google Scholar
11 Lewiecki EM , KeavenyTM, KopperdahlDL, et al.Once-monthly oral ibandronate improves biomechanical determinants of bone strength in women with postmenopausal osteoporosis. J Clin Endocrinol Metab2009;94:171–180.CrossrefPubMed Google Scholar
12 Bauss F , LallaS, EndeleR, HothornLA. Effects of treatment with ibandronate on bone mass, architecture, biomechanical properties, and bone concentration of ibandronate in ovariectomized aged rats. J Rheumatol2002;29:2200–2208.PubMed Google Scholar
13 Odvina CV , ZerwekhJE, RaoDS, et al.Severely suppressed bone turnover: a potential complication of alendronate therapy. J Clin Endocrinol Metab2005;90:1294–1301.CrossrefPubMed Google Scholar
14 Li J , MashibaT, BurrDB. Bisphosphonate treatment suppresses not only stochastic remodeling but also the targeted repair of microdamage. Calcif Tissue Int2001;69:281–286. Google Scholar
15 Goh SK , YangKY, KohJS, et al.Subtrochanteric insufficiency fractures in patients on alendronate therapy: a caution. J Bone Joint Surg [Br]2007; 89-B:349–353.CrossrefPubMed Google Scholar
16 Neviaser AS , LaneJM, LenartBA, Edobor-OsulaF, LorichDG. Low-energy femoral shaft fractures associated with alendronate use. J Orthop Trauma2008;22:346–350.CrossrefPubMed Google Scholar
17 Bauss F , WagnerM, HothornLH. Total administered dose of ibandronate determines its effects on bone mass and architecture in ovariectomized aged rats. J Rheumatol2002;29:990–998.PubMed Google Scholar
18 Savaridas T , WallaceRJ, SalterDM, SimpsonAHRW. Do bisphosphonates inhibit direct fracture healing?: a laboratory investigation using an animal model. Bone Joint J2013;95-B:1263–1268.CrossrefPubMed Google Scholar
19 Dawson SP , RossE, MacGillivrayTJ, MuirAY, SimpsonAHRW. The use of digital x-ray to monitor decalcification in sheep femora. IFMBE Proceedings2009;2:322–324. Google Scholar
20 Sedlin ED , HirschC. Factors affecting the determination of the physical properties of femoral cortical bone. Acta Orthop Scand1966;37:29–48.CrossrefPubMed Google Scholar
21 Turner CH , BurrDB. Basic biomechanical measurements of bone: a tutorial. Bone1993;14:595–608.CrossrefPubMed Google Scholar
22 Mashiba T , HiranoT, TurnerCH, et al.Suppressed bone turnover by bisphosphonates increases microdamage accumulation and reduces some biomechanical properties in dog rib. J Bone Miner Res2000;15:613–620.CrossrefPubMed Google Scholar
23 Ferretti JL , CointryG, CapozzaR, et al.Biomechanical effects of the full range of useful doses of (3-amino-1-hydroxypropylidene)-1,1-bisphosphonate (APD) on femur diaphyses and cortical bone tissue in rats. Bone Miner1990;11:111–122.CrossrefPubMed Google Scholar
24 Kippo K , HannuniemiR, LaurénL, et al.Effect of clodronate treatment on established bone loss in ovariectomized rats. Bone1998;23:333–342.CrossrefPubMed Google Scholar
25 Hornby SB , EvansGP, HornbySL, et al.Long-term zoledronic acid treatment increases bone structure and mechanical strength of long bones of ovariectomized adult rats. Calcif Tissue Int2003;72:519–527.CrossrefPubMed Google Scholar
26 Broulik PD , RosenkrancováJ, RůzickaP, SedlácekR. Effect of alendronate administration on bone mineral density and bone strength in castrated rats. Horm Metab Res2005;37:414–418.CrossrefPubMed Google Scholar
27 Reim NS , BreigB, StahrK, et al.Cortical bone loss in androgen-deficient aged male rats is mainly caused by increased endocortical bone remodeling. J Bone Miner Res2008;23:694–704.CrossrefPubMed Google Scholar
28 Danielsen CC , MosekildeL, AndreassenTT. Long-term effect of orchidectomy on cortical bone from rat femur: bone mass and mechanical properties. Calcif Tissue Int1992;50:169–174.CrossrefPubMed Google Scholar
29 Turner RT , VandersteenhovenJJ, BellNH. The effects of ovariectomy and 17 beta-estradiol on cortical bone histomorphometry in growing rats. J Bone Miner Res1987;2:115–122.CrossrefPubMed Google Scholar
30 Nunamaker DM . Experimental models of fracture repair. Clin Orthop Relat Res1998;355 (Suppl):S56–S65.CrossrefPubMed Google Scholar
31 O’Loughlin PF , MorrS, BogunovicL, et al.Selection and development of preclinical models in fracture-healing research. J Bone Joint Surg [Am]2008; 90-A(Suppl 1):79–84.CrossrefPubMed Google Scholar
32 Bentolila V , BoyceTM, FyhrieDP, et al.Intracortical remodeling in adult rat long bones after fatigue loading. Bone1998;23:275–281.CrossrefPubMed Google Scholar
33 Karlsson M , NilssonJA, SernboI, et al.Changes of bone mineral mass and soft tissue composition after hip fracture. Bone1996;18:19–22.CrossrefPubMed Google Scholar
34 Peter CP , CookWO, NunamakerDM, et al.Effect of alendronate on fracture healing and bone remodeling in dogs. J Orthop Res1996;14:74–79.CrossrefPubMed Google Scholar
35 Zioupos P , CurreyJD. Changes in the stiffness, strength, and toughness of human cortical bone with age. Bone1998;22:57–66.CrossrefPubMed Google Scholar
36 Harris B , DoreySE, CookeRG. Strength and toughness of fibre composites. Compos Sci Technol1988;31:121–141. Google Scholar
37 Zebaze RM , Ghasem-ZadehA, BohteA, et al.Intracortical remodelling and porosity in the distal radius and post-mortem femurs of women: a cross-sectional study. Lancet2010;375:1729–1736.CrossrefPubMed Google Scholar
Funding statement:
This research was funded by The Osteosynthesis & Trauma Care Foundation (OTC) in collaboration with Association Internationale Pour L´Ostéosynthèse Dynamique (AIOD) and The Royal College of Surgeons of Edinburgh (RCSEd).
Author contributions:
T. Savaridas: Study design, Data collection and analysis, Manuscript preparation
R. J. Wallace: Study design, Data collection and analysis, Manuscript preparation
S. Dawson: Development of image analysis program, Generating image analysis results, proofreading, Final edits of paper
A. H. R. W. Simpson: Study design and manuscript preparation
ICMJE Conflict of Interest:
None declared
© Wallace et al. This is an open-access article distributed under the terms of the Creative Commons Attributions licence, which permits unrestricted use, distribution, and reproduction in any medium, but not for commercial gain, provided the original author and source are credited.