Abstract
Objectives
In order to elucidate the influence of sympathetic nerves on lumbar radiculopathy, we investigated whether sympathectomy attenuated pain behaviour and altered the electrical properties of the dorsal root ganglion (DRG) neurons in a rat model of lumbar root constriction.
Methods
Sprague-Dawley rats were divided into three experimental groups. In the root constriction group, the left L5 spinal nerve root was ligated proximal to the DRG as a lumbar radiculopathy model. In the root constriction + sympathectomy group, sympathectomy was performed after the root constriction procedure. In the control group, no procedures were performed. In order to evaluate the pain relief effect of sympathectomy, behavioural analysis using mechanical and thermal stimulation was performed. In order to evaluate the excitability of the DRG neurons, we recorded action potentials of the isolated single DRG neuron by the whole-cell patch-clamp method.
Results
In behavioural analysis, sympathectomy attenuated the mechanical allodynia and thermal hyperalgesia caused by lumbar root constriction. In electrophysiological analysis, single isolated DRG neurons with root constriction exhibited lower threshold current, more depolarised resting membrane potential, prolonged action potential duration, and more depolarisation frequency. These hyperexcitable alterations caused by root constriction were significantly attenuated in rats treated with surgical sympathectomy.
Conclusion
The present results suggest that sympathectomy attenuates lumbar radicular pain resulting from root constriction by altering the electrical property of the DRG neuron itself. Thus, the sympathetic nervous system was closely associated with lumbar radicular pain, and suppressing the activity of the sympathetic nervous system may therefore lead to pain relief.
Article focus
To clarify whether sympathectomy could reduce pain behaviour and excitability of dorsal root ganglion (DRG) neuron in a rat model of lumbar radiculopathy by behavioural and electrophysiological analyses
Key messages
The sympathetic nervous system generates pain after nerve damage, although it does not influence pain generation under normal conditions. It was reported that suppressing sympathetic nerve activity reduced neuropathic pain and several mechanisms have been suggested. However, alteration of the electrical property of the DRG neurons by sympathectomy has not been well investigated
We used single isolated DRG neurons in the electrophysiological analysis, which are not affected by surrounding factors such as blood flow and effects of neurotransmitters. Therefore, the present result suggests that sympathectomy attenuates pain behaviour caused by root constriction by altering the electrical property of the DRG neuron itself
Strengths and limitations
To our knowledge, this is the first study to investigate the alterations of the electrical property of the single isolated DRG neuron by sympathectomy in an animal model of lumbar radiculopathy
The limitations of this study are that membrane currents were not investigated. Further studies are needed to clarify the mechanism underlying the action potential changes caused by sympathectomy
Introduction
Lumbar radicular pain caused by lumbar disc herniation or lumbar spinal canal stenosis is one of the most common symptoms of neuropathic pain treated by orthopaedic surgeons or pain clinicians.1-6 Patients with lumbar radicular pain are usually treated with oral medication, epidural block or surgery; however, some patients are difficult to treat using these methods and are afflicted with chronic pain. Previous studies have suggested that the sympathetic nervous system plays an important role in generating chronic neuropathic pain.1-3,7-11 However, it does not generate pain under normal circumstances. In neuropathic pain, an abnormal sympathetic-somatosensory interaction is formed that leads to sympathetically maintained pain.11,12 To treat such patients, in particular those suffering from chronic pain such as complex regional pain syndrome, sympathetic nerve blocks and surgical sympathectomy are used, which are often effective.9,10
It has been reported that sympathetic nerve fibres sprout in the dorsal root ganglion (DRG) after nerve injury,1,7,11,13-16 and that sympathectomy can relieve mechanical allodynia and thermal hyperalgesia in peripheral nerve injury models.2,8,13,14,17 In contrast, some animal studies have reported that sympathectomy does not reduce allodynia and hyperalgesia.18-21 In addition, a clinical report demonstrated that the placebo effect of phentolamine, a sympathetic blocker for painful polyneuropathies, was responsible for pain relief.22 In summary, the pain relief effect of sympathectomy for neuropathic pain, especially for lumbar radicular pain, has not been well investigated.
In our previous study, we investigated the influence of the sympathetic nervous system on lumbar radicular pain using a lumbar root constriction model.1,3 Immunohistochemical analysis showed that sympathetic nerve fibres were more abundant on the constriction side of the DRG than on the contralateral side.3 Norepinephrine (NE), a neurotransmitter of the sympathetic nervous system, enhances the excitability of the DRG neurons,1 suggesting that the sympathetic nervous system is closely related to lumbar radicular pain.
We used a model of lumbar radiculopathy and behavioural and electrophysiological analyses to investigate whether sympathectomy could attenuate pain-related behaviour and alter the electrical properties of the DRG neurons.
Materials and Methods
Experimental animals
The Sapporo Medical University Animal Care and Use Committee approved all the experimental protocols used in this study. We used a total of 60 adult male Sprague-Dawley rats weighing between 150 g and 200 g and divided them into three equal experimental groups: root constriction (RC) group, RC+ sympathectomy (Syx) group, and control group (n = 20 in each group; each comprising ten for the behavioural study and ten for the electrophysiological study).
Surgical procedures
Root constriction and sympathectomy were performed by a method previously described3,4 under a microscope on rats anaesthetised intraperitoneally with sodium pentobarbital (50 mg/kg) under sterile conditions. Each rat was placed in the prone position, and the L5-6 intervertebral space was identified. Through a midline dorsal incision at L4-S1, the paraspinal muscles were retracted to expose the left L5-6 facet joint. A left L5 hemilaminectomy and L5-6 partial facetectomy were performed. The incision and muscle exposures were minimised in order to avoid invasion of the DRG neurons as much as possible. The left L5 spinal nerve root and DRG were carefully exposed, and the L5 spinal nerve root was tightly ligated extradurally with 8-0 nylon suture proximal to the DRG in the RC group. In the RC+Syx group, sympathectomy was immediately performed after the root constriction procedure. The animals were placed in the supine position, and later, using a transperitoneal approach, the L2 to L5 sympathetic ganglions and chains on both sides were visualised by gently retracting the psoas major and resected. In the control group, no such procedures were performed.
Behavioural analysis
This was undertaken in order to evaluate mechanical allodynia and thermal hyperalgesia. In order to assess the mechanical withdrawal response, the rats were placed in a Plexiglas chamber (IITC Life Science Inc., Woodland Hills, California) measuring 18 cm × 25 cm × 18 cm above a wire mesh floor, which allowed full access to the hind paw, and behavioural accommodation was allowed for at least 20 minutes before the test. The mechanical withdrawal response was measured as the frequency of withdrawals of the hind paw elicited by a defined mechanical stimulus of 3.4 g using a calibrated nylon filament (Semmes-Weinstein Monofilaments; North Coast Medical Inc., San Jose, California). The mechanical stimulus was applied to the middle area between the foot pads on the plantar surface of the left (constriction side) and right (contralateral side) hind paw. Each hind paw was probed consecutively by ten tactile stimulations alternating between the left and right. Each test was repeated three times at intervals of at least 10 minutes, which resulted in each foot receiving 30 mechanical stimulations. The mechanical sensitivity was assessed by counting the total number of withdrawal responses elicited out of a total possible score of 30. The mechanical withdrawal frequency for each rat was expressed as the number of responses from the contralateral side (not constricted) subtracted from the number of responses from the ipsilateral side (constricted). When the mechanical sensitivity of the hind paws did not differ, the mechanical withdrawal frequency was calculated as zero. The procedure was performed two days before and three, seven, ten, 14, 21, and 28 days after surgery.
In order to assess the thermal withdrawal response, the rats were placed in a Plexiglas chamber on a glass platform and allowed to acclimatise for at least 20 minutes before the test. The thermal withdrawal response was measured as the latency of hind paw withdrawals elicited by a radiant heat source (Tail Flick Analgesia Meter; IITC Inc.), which was moved beneath an area of the hind paw that was flush against the glass. The intensity of the heat stimulus was constant throughout all experiments and adjusted to elicit a quick withdrawal reflex at a latency of approximately 6 seconds to 8 seconds in the control rats. A cut-off time of 10 seconds was set to prevent tissue damage. Each hind paw was tested five times at intervals of at least 5 minutes, alternating between the left and right. The mean withdrawal latency was calculated from the last four measurements. Consequently, the thermal withdrawal latency of each rat was defined as the latency of the contralateral response (not constricted) minus the ipsilateral response (constricted). Positive and negative scores indicated increased and decreased sensitivity of the ipsilateral hind paw, respectively. This test was performed after evaluating mechanical sensitivity. Investigators blinded to the surgical protocol of each rat performed these behavioural tests.1,3,4,23
Electrophysiological analysis
All experiments were performed at room temperature (22°C to 24°C). At 10 to 14 days after surgery, the rats were killed. Under a stereomicroscope, the ipsilateral L5 DRG was quickly excised and placed in oxygenated normal Tyrode’s solution (mmol/L) composed of NaCl 143; KCl 5.4; CaCl2 1.8; MgCl2 0.5; NaH2PO4 0.33; glucose 5.5; and HEPES 5.0 (pH 7.4 by NaOH). The excised DRG was immersed in Ca2+-free Tyrode’s solution at room temperature (> 20 minutes) to remove extracellular Ca2+. The tissue was digested with collagenase (8 mg/mL, Type II, 034–10533; Wako Pure Chemical Industries, Tokyo, Japan) for 80 minutes in a shaking incubator (37°C, 1.5 Hz) and rinsed with Ca2+-free Tyrode’s solution. The DRG neurons were dissociated in Ca2+-free Tyrode’s solution by stirring with a hand-made pipette.1,4
In order to evaluate the excitability of the DRG neurons, we recorded action potentials (APs) in response to depolarising current by the whole-cell patch-clamp method (Axopatch 200B; Molecular Devices, Sunnyvale, California). Because the small DRG neurons may be involved in the transmission of nociceptive information, we selected small cells < 40 µm in diameter for patch-clamp recordings.24,25 We used normal Tyrode’s solution as the external solution. The composition of the internal pipette solution used in current-clamp recordings was (mmol/L) as follows: K-aspartate 110; KCl 20; MgCl2 1.0; ATP-K2 5.0; phosphocreatine-K2 5.0; EGTA 5; and HEPES 5.0 (pH 7.4 by KOH).
We used two protocols to perform current-clamp recordings; a short stimulation protocol (depolarising currents of 0.2 nA to 4.0 nA, 0.5 ms) and a long stimulation protocol (depolarising currents of 0.01 nA to 0.39 nA (increments of 0.02 nA), 1000 ms). In order to evaluate the excitability of the DRG neurons in the three experimental groups, we examined the electrical properties such as threshold current, threshold voltage, RMP, amplitude, AHP, APD50, and dV/dt max in the short stimulation protocol. The threshold current was defined as the minimum current required in order to evoke an AP. The threshold voltage was defined as the beginning of the upstroke of AP. The RMP was recorded 3 minutes after a stable recording was first obtained. The amplitude was measured from the RMP baseline to the peak. AHP was measured from the RMP to the valley nadir. APD50 was measured as the interval from the onset of AP to the point of 50% repolarisation. The dV/dt max was the maximum rate of depolarisation of AP. In the long stimulation protocol we examined the maximum number of AP spikes for each current, which was defined as the maximum spike count.
Statistical analysis
All data are expressed as mean and standard error of the mean (sem). Both behavioural and electrophysiological data were analysed by one-way factorial measures of analysis of variance (ANOVA) and the Tukey-Kramer test among the three experimental groups. Differences were considered to be statistically significant at a p-value < 0.05.
Results
Behavioural analysis
The duration of withdrawal frequencies in the three experimental groups (n = 10 each) in response to mechanical stimulation using a 3.4 g von Frey filament are shown in Figure 1a, and in response to thermal stimulation in Figure 1b.
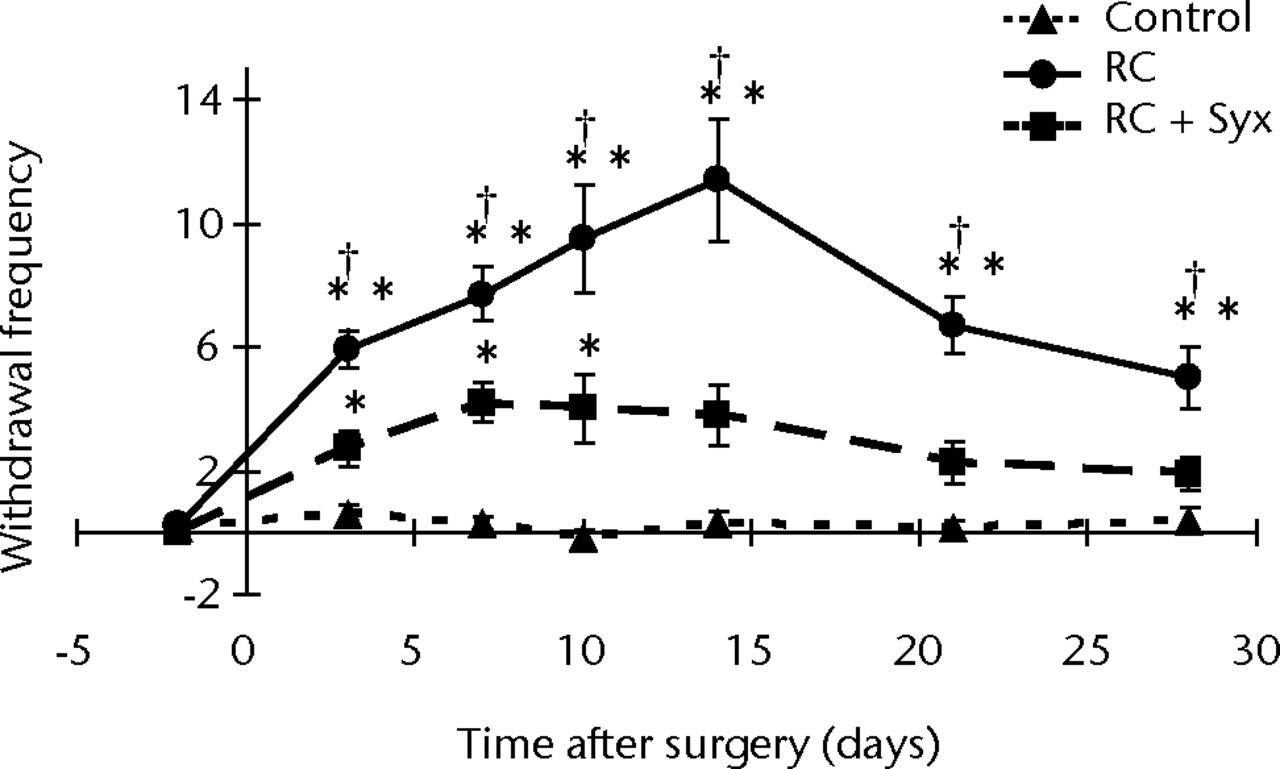
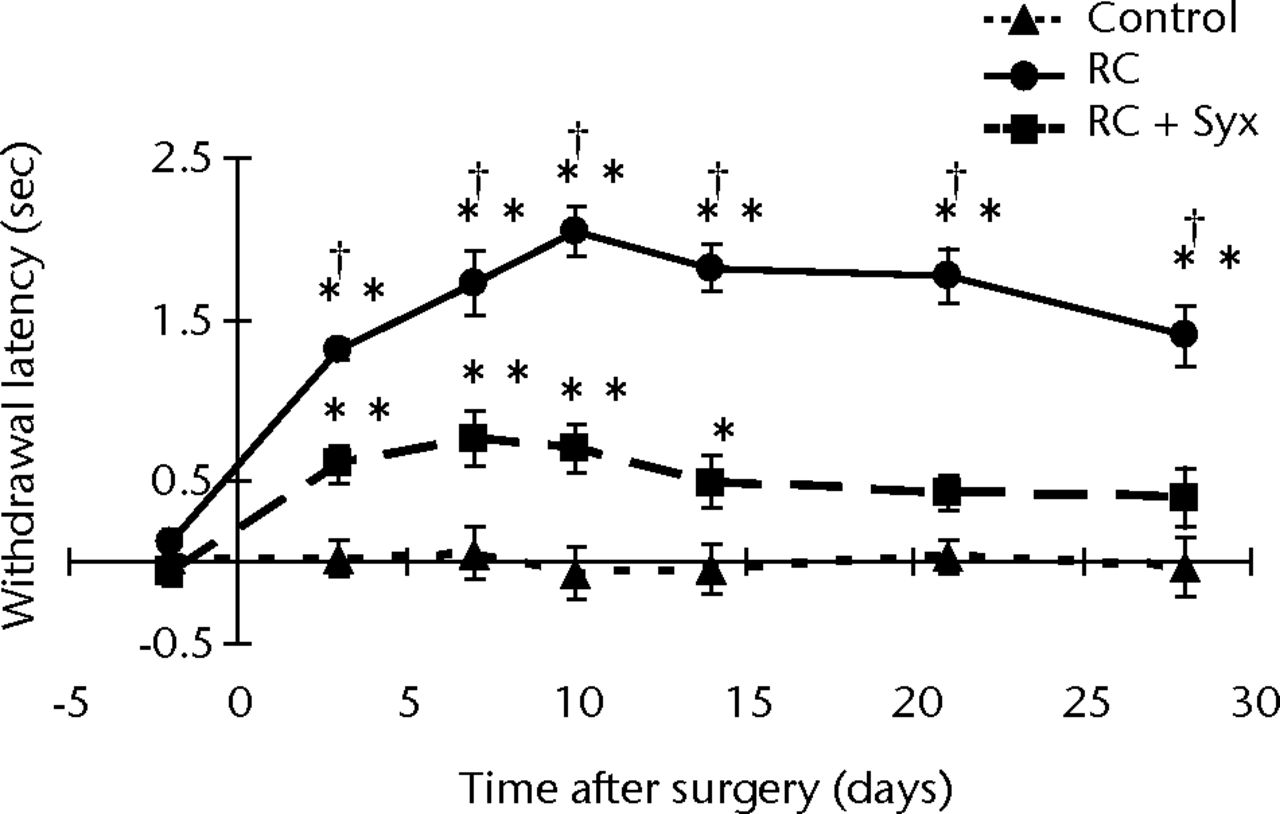
Figs. 1a - 1b
Graphs showing the mean responses to a) mechanical stimulation, with the root constriction (RC) group showing mechanical hypersensitivity from three to 28 days post-operatively surgery, but significant reduction of hypersensitivity in the RC + sympathectomy (RC+Syx) group, and b) thermal stimulation, with the RC group showing thermal hypersensitivity from three to 28 days post-operatively, again with the hypersensitivity significantly reduced in the RC+Syx group. Mechanical hypersensitivity from three to ten days post-operatively and thermal hypersensitivity from three to 14 days post-operatively was not completely reduced compared with the control group. Error bars denote the standard error of the mean (* p < 0.05 versus control; ** p < 0.01 versus control; † p < 0.05 versus RC+Syx).
The mechanical withdrawal frequencies in the RC group increased from three to 28 days after surgery, compared with the control group, but significantly reduced in the RC+Syx group over the same time period. The mechanical withdrawal frequencies were not completely reduced in the RC+Syxgroup compared with the control group at three, seven, or ten days after surgery, but there was no significant difference between the RC+Syx and control groups at 14, 21, and 28 days after surgery (Fig. 1a).
Thermal hypersensitivity also increased in the RC group compared with the control group from three to 28 days after surgery and was significantly reduced in the RC+Syx group. The withdrawal latencies were not completely reduced in the RC+Syx group compared with the control group at three, seven, ten, or 14 days after surgery. No significant difference between the RC+Syx and control groups at 21 and 28 days (Fig. 1b) was observed.
Electrophysiological analysis
The action potentials (APs) of the DRG neurons in the three experimental groups (n = 10 each) recorded by the whole-cell patch-clamp method are shown in Figure 2. In the RC group, the DRG neurons exhibited a significantly lower threshold current, more depolarised resting membrane potential (RMP), prolonged action potential duration at 50% repolarisation (APD50), and greater maximum (max) spike count compared with the control group. These hyperexcitable alterations were attenuated in the RC+Syx group and there were no significant differences between the RC+Syx and control groups (Fig. 3). None of the other parameters tested (threshold voltage, amplitude, after hyperpolarisation (AHP), and dV/dt max) exhibited significant differences in the three groups. Changes in the discharge pattern and the excitability of the DRG neurons in the long stimulation protocol are shown in Figure 4. In the RC+Syx group, the mean value of the max spike count was 5.0 (sem 1.5), which was significantly smaller than the mean of 13.7 (sem 1.7) in the RC group.
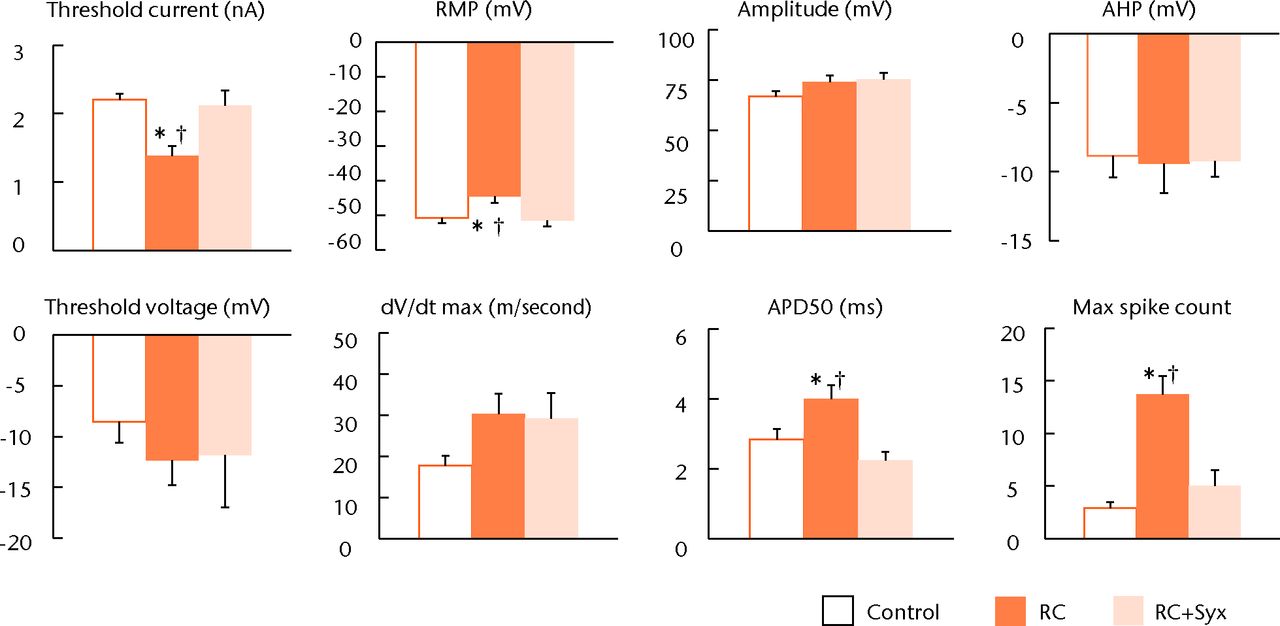
Fig. 2
Bar charts showing the electrophysiological properties of the dorsal root ganglion (DRG) in the three experimental groups. The root constriction (RC) neurons exhibited lower threshold current, increased depolarised resting membrane potential (RMP), prolonged action potential duration at 50% repolarisation (APD50), and increasing depolarisation frequency (max spike count) compared with the control neurons. These hyperexcitable alterations caused by root constriction were significantly attenuated by surgical sympathectomy. There were no significant changes in any of the other parameters tested such as threshold voltage, amplitude and after hyperpolarisation (AHP) (* p < 0.05 versus control; † p < 0.05 versus RC+Syx).
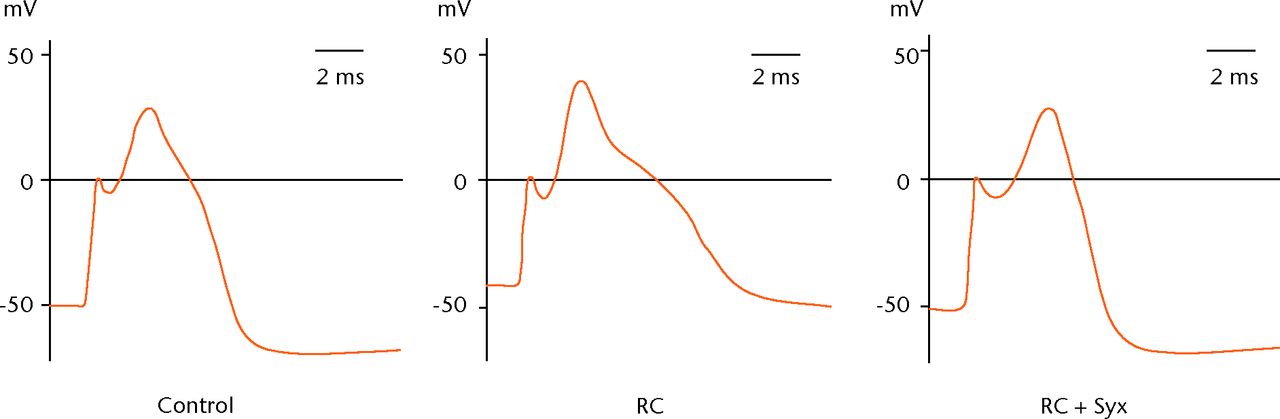
Fig. 3
Example graphs showing action potentials in the short stimulation protocol. The root constriction (RC) neurons exhibited more depolarised resting membrane potential (RMP) and prolonged action potential duration at 50% repolarisation (APD50) compared with the control neurons. These changes were not observed in the RC+sympathectomy (Syx) neurons that exhibited properties similar to controls.
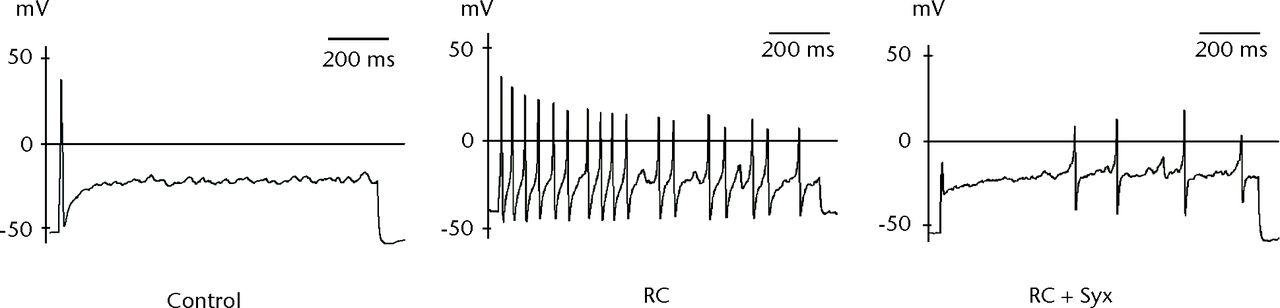
Fig. 4
Responses of the dorsal root ganglion (DRG) neurons in the long stimulation protocol (maximum spike count). The root constriction (RC) neuron exhibited an increased number of action potential (AP) spikes and more depolarised resting membrane potential (RMP) compared with the control neurons. These hyperexcitable alterations were reduced in the RC+ sympathectomy (Syx) neurons.
Discussion
The sympathetic nervous system is considered to be one of the most important factors in the generation of neuropathic pain.1,3,12 McLachlan et al11 reported that in the sciatic nerve ligation model, sympathetic nerve fibres increased in the corresponding DRGs and sprouted to the DRG somata, forming basket-like structures. Other studies also reported that sympathetic sprouting occurred in various neuropathic pain models.7,13-16,26,27 In addition, Shinder et al26 demonstrated that sympathetic basket-skeins were observed in the DRGs excised from human neuropathic pain patients. We have shown that sympathetic nerve fibres sprouted to the DRG myelin sheaths in the DRG neurons in our root constriction model.3 This implies that the sympathetic nervous system has a direct and/or indirect influence on the DRG, causing not only peripheral nerve injury but also lumbar root damage. Thus, the sympathetic nervous system is closely associated with neuropathic pain, and suppressing the activity of the sympathetic nervous system might therefore lead to pain relief.
In the present behavioural study, surgical sympathectomy significantly attenuated mechanical allodynia and thermal hyperalgesia caused by root constriction. However, in the RC+Syx group, mechanical and thermal hypersensitivities were not reduced significantly compared with the control group, but they were significantly attenuated compared to the RC group. Sympathectomy may be insufficient to alleviate radicular pain, because not only the sympathetic nervous system but also other factors such as the inflammatory response and effects of neurotransmitters are related to radicular pain. Meanwhile, several studies have also reported that sympathectomy reduces mechanical allodynia and thermal hyperalgesia in various animal pain models.2,8,17 Clinical reports have shown that a sympathetic nerve block reduces lumbar radicular pain28,29 and sympathectomy increases intraradicular,30 cauda equina,31 and sciatic nerve blood flow.32 It has also been suggested that the recovery of reduced DRG blood flow might prevent allodynia in a radicular pain model.8 Sekiguchi et al2 reported that sympathectomy reduced mechanical allodynia, tumor necrosis factor-alpha (TNF-α) expression, and DRG neuron apoptosis following nerve root crush injury.2 Chen et al17 showed that surgical and chemical sympathectomy significantly attenuated bee venom-induced mechanical hyperalgesia and inflammatory response. Therefore, sympathectomy may exert its analgesic effect through several mechanisms.
In the present electrophysiological study, the root constriction DRG neurons exhibited a lower threshold current, more depolarised RMP, prolonged AP duration, and max spike count. Kirita et al4 reported that the Na+ current was significantly increased in the root constriction neurons and lumbar radicular pain may be associated with increased excitability of the involved DRG neurons. Another report showed that the fast Na+ current in the DRGs increased in a peripheral nerve injury model.33 The present electrophysiological analysis showed that surgical sympathectomy significantly attenuated the hyperexcitability of the root constriction DRG neurons. In particular, more hyperpolarised RMP and reduced AP duration in the RC+Syx group may suggest that outward currents were increased by K+ channel activity, because RMP and AP duration are both significantly dependent on K+ channel activity. Previous reports demonstrated that an ATP-sensitive K+ channel activator reversed the hyperexcitability induced by bradykinin in the DRG neurons34 and expression of inwardly rectifying K+ channels in chronically compressed DRG neurons suppressed neuronal excitability and hyperalgesia.35 These reports suggest that alteration in a few types of K+ channels is one of the important factors causing neuropathic pain. In addition, Xie et al7 reported that K+ channel blockers increase the spontaneous activity of the DRG neuron and sympathetic sprouting, and Honma et al36 reported that NE inhibits Ca2+-activated K+ channel activity and outward K+ currents leading to cell membrane depolarisation. The results of this study along with previous reports imply that the sympathetic nerves contribute to pain by altering the K+ channel.
One of the main advantages of this study was the use of isolated DRG neurons in the electrophysiological analysis. Isolated DRG neurons are not influenced by their surrounding factors such as blood flow and presence of neurotransmitters. Therefore, the results of the present study show that root constriction altered the DRG neuron itself, and this alteration was inhibited by sympathectomy. This result may be evidence demonstrating that the sympathetic nervous system is one of the key factors in pain generation caused by nerve root damage as a result of alterations in the DRG neuron itself. In fact, the excitability of isolated DRG neurons from normal rats did not alter with NE application, but significantly increased in the DRG neurons with root constriction.1 These findings indicate that the DRG neuron itself is changed by root constriction. In addition, DRG neuron hypersensitivity has been improved by sympathectomy immediately after root constriction. This result suggests that the sympathetic nervous system plays an important role in altering the DRG neuron as a result of root constriction, and that suppressing the activity of the sympathetic nerve at an early stage after root constriction may be important in suppressing the development of a pain cycle because of root damage.
Membrane currents were not investigated in the present study. AP changes such as hyperpolarised RMP and reduced AP duration observed in the RC+Syx group might be caused not only by alterations in the K+ channel but also by another ion channel, such as the Cl– channel. Further studies are needed to clarify the mechanism underlying the AP changes caused by sympathectomy.
Conclusions
In behavioural analysis, sympathectomy significantly attenuated mechanical allodynia and thermal hyperalgesia caused by root constriction, but the pain relief effect was not complete. In electrophysiological analysis, hyperexcitable alterations of the DRG neurons caused by root constriction such as lower threshold current, more depolarised RMP, prolonged APD50, and max spike count were significantly attenuated by surgical sympathectomy. We used isolated DRG neurons, which are not affected by surrounding factors such as blood flow and presence of neurotransmitters. Therefore, the present results suggest that sympathectomy attenuates lumbar radicular pain caused by root constriction by altering the electrical property of the DRG neuron itself. Sympathectomy hyperpolarised the RMP of the RC neurons and shortened the AP duration. The present results suggest that sympathectomy attenuates excitability of the DRG neurons by altering the K+ channels and may contribute to pain relief in a lumbar radiculopathy model.
1 Tanimoto K , TakebayashiT, KobayashiT, TohseN, YamashitaT. Does norepinephrine influence pain behavior mediated by dorsal root ganglia?: a pilot study. Clin Orthop Relat Res2011;469:2568–2576.CrossrefPubMed Google Scholar
2 Sekiguchi M , KobayashiH, SekiguchiY, KonnoS, KikuchiS. Sympathectomy reduces mechanical allodynia, tumor necrosis factor-alpha expression, and dorsal root ganglion apoptosis following nerve root crush injury. Spine (Phila Pa 1976)2008;33:1163–1169.CrossrefPubMed Google Scholar
3 Mizuno S , TakebayashiT, KiritaT, et al.The effects of the sympathetic nerves on lumbar radicular pain: a behavioural and immunohistochemical study. J Bone Joint Surg [Br]2007;89-B:1666–1672.CrossrefPubMed Google Scholar
4 Kirita T , TakebayashiT, MizunoS, et al.Electrophysiologic changes in dorsal root ganglion neurons and fibreal changes in a lumbar radiculopathy model. Spine (Phila Pa 1976)2007;32:E65–E72. Google Scholar
5 Hasue M . Pain and the nerve root: an interdisciplinary approach. Spine (Phila Pa 1976)1993;18:2053–2058. Google Scholar
6 Takeuchi H , KawaguchiS, MizunoS, et al.Gene expression profile of dorsal root ganglion in a lumbar radiculopathy model. Spine (Phila Pa 1976)2008;33:2483–2488.CrossrefPubMed Google Scholar
7 Xie W , StrongJA, LiH, ZhangJM. Sympathetic sprouting near sensory neurons after nerve injury occurs preferentially on spontaneously active cells and is reduced by early nerve block. J Neurophysiol2007;97:492–502.CrossrefPubMed Google Scholar
8 Murata Y , OlmarkerK, TakahashiI, TakahashiK, RydevikB. Effects of lumbar sympathectomy on pain behavioral changes caused by nucleus pulposus-induced spinal nerve damage in rats. Eur Spine J2006;15:634–640.CrossrefPubMed Google Scholar
9 Dunn DG . Chronic regional pain syndrome, type 1: Part II. AORN J2000;72:643–651.CrossrefPubMed Google Scholar
10 Dunn D . Chronic regional pain syndrome, type 1: Part I. AORN J2000;72:422–432.CrossrefPubMed Google Scholar
11 McLachlan EM , JänigW, DevorM, MichaelisM. Peripheral nerve injury triggers noradrenergic sprouting within dorsal root ganglia. Nature1993;363:543–546.CrossrefPubMed Google Scholar
12 Roberts WJ . A hypothesis on the physiological basis for causalgia and related pains. Pain1986;24:297–311.CrossrefPubMed Google Scholar
13 Pertin M , AllchorneAJ, BeggahAT, WoolfCJ, DecosterdI. Delayed sympathetic dependence in the spared nerve injury (SNI) model of neuropathic pain. Mol Pain2007;3:21.CrossrefPubMed Google Scholar
14 Xie W , StrongJA, ZhangJM. Increased excitability and spontaneous activity of rat sensory neurons following in vitro stimulation of sympathetic fiber sprouts in the isolated dorsal root ganglion. Pain2010;151:447–459.CrossrefPubMed Google Scholar
15 Kim HJ , NaHS, BackSK, HongSK. Sympathetic sprouting in sensory ganglia depends on the number of injured neurons. Neuroreport2001;12:3529–3532.CrossrefPubMed Google Scholar
16 Chien SQ , LiC, LiH, XieW, PabloCS, ZhangJM. Sympathetic fiber sprouting in chronically compressed dorsal root ganglia without peripheral axotomy. J Neuropathic Pain Symptom Palliation2005;1:19–23.CrossrefPubMed Google Scholar
17 Chen HS , QuF, HeX, WangY, WenWW. Chemical or surgical sympathectomy prevents mechanical hyperalgesia induced by intraplantar injection of bee venom in rats. Brain Res2010;1353:86–93. Google Scholar
18 Zhao C , ChenL, TaoYX, et al.Lumbar sympathectomy attenuates cold allodynia but not mechanical allodynia and hyperalgesia in rats with spared nerve injury. J Pain2007;8:931–937. Google Scholar
19 Shir Y , SeltzerZ. Effects of sympathectomy in a model of causalgiform pain produced by partial sciatic nerve injury in rats. Pain1991;45:309–320.CrossrefPubMed Google Scholar
20 Lavand’homme P , PanHL, EisenachJC. Intrathecal neostigmine, but not sympathectomy, relieves mechanical allodynia in a rat model of neuropathic pain. Anesthesiology1998;89:493–499. Google Scholar
21 Ringkamp M , EschenfelderS, GrethelEJ, et al.Lumbar sympathectomy failed to reverse mechanical allodynia- and hyperalgesia-like behavior in rats with L5 spinal nerve injury. Pain1999;79:143–153.CrossrefPubMed Google Scholar
22 Verdugo RJ , CamperoM, OchoaJL. Phentolamine sympathetic block in painful polyneuropathies. II. Further questioning of the concept of ‘sympathetically maintained pain’. Neurology1994;44:1010–1014. Google Scholar
23 Terashima Y , KawamataM, TakebayashiT, et al.Changes in synaptic transmission of substantia gelatinosa neurons in a rat model of lumbar radicular pain revealed by in vivo patch-clamp recording. Pain2011;152:1024–1032.CrossrefPubMed Google Scholar
24 Scroggs RS , FoxAP. Calcium current variation between acutely isolated adult rat dorsal root ganglion neurons of different size. J Physiol1992;445:639–658.CrossrefPubMed Google Scholar
25 Stucky CL , LewinGR. Isolectin B(4)-positive and -negative nociceptors are functionally distinct. J Neurosci1999;19:6497–6505.CrossrefPubMed Google Scholar
26 Shinder V , Govrin-LippmannR, CohenS, et al.Structural basis of sympathetic-sensory coupling in rat and human dorsal root ganglia following peripheral nerve injury. J Neurocytol1999;28:743–761.CrossrefPubMed Google Scholar
27 Zhang JM , StrongJA. Recent evidence for activity-dependent initiation of sympathetic sprouting and neuropathic pain. Sheng Li Xue Bao2008;60:617–627.PubMed Google Scholar
28 Boas RA . Sympathetic nerve blocks: in search of a role. Reg Anesth Pain Med1998;23:292–305.CrossrefPubMed Google Scholar
29 Stone LS , BrobergerC, VulchanovaL, et al.Differential distribution of alpha2A and alpha2C adrenergic receptor immunoreactivity in the rat spinal cord. J Neurosci1998;18:5928–5937.CrossrefPubMed Google Scholar
30 Yabuki S , KikuchiS. Nerve root infiltration and sympathetic block: an experimental study of intraradicular blood flow. Spine (Phila Pa 1976)1995;20:901–906. Google Scholar
31 Onda A , YabukiS, IwabuchiM, et al.Lumbar sympathectomy increases blood flow in a dog model of chronic cauda equina compression. J Spinal Disord Tech2004;17:522–525.CrossrefPubMed Google Scholar
32 Kinoshita Y , MonafoWW. Guanethidine chemical sympathectomy: spinal cord and sciatic nerve blood flow. Am J Physiol1993;265:H1155–H1159.CrossrefPubMed Google Scholar
33 Abdulla FA , SmithPA. Changes in Na(+) channel currents of rat dorsal root ganglion neurons following axotomy and axotomy-induced autotomy. J Neurophysiol2002;88:2518–2529.CrossrefPubMed Google Scholar
34 Du X , WangC, ZhangH. Activation of ATP-sensitive potassium channels antagonize nociceptive behavior and hyperexcitability of bDRG neurons from rats. Mol Pain2011;7:35. Google Scholar
35 Ma C , RosenzweigJ, ZhangP, JohnsDC, LaMotteRH. Expression of inwardly rectifying potassium channels by an inducible adenoviral vector reduced the neuronal hyperexcitability and hyperalgesia produced by chronic compression of the spinal ganglion. Mol Pain2010;6:65.CrossrefPubMed Google Scholar
36 Honma Y , YamakageM, NinomiyaT. Effects of adrenergic stimulus on the activities of Ca2+ and K+ channels of dorsal root ganglion neurons in a neuropathic pain model. Brain Res1999;832:195–206.CrossrefPubMed Google Scholar
Funding statement:
None declared
Author contributions:
T. Iwase: Animal surgery, Behavioural studies, Electrophysiological studies, Data analysis, Manuscript preparation
T. Takebayashi: Study design, Manuscript preparation
K. Tanimoto: Electrophysiological studies, Data analysis
Y. Terashima: Animal surgery, Behavioural studies
T. Miyakawa: Animal surgery, Behavioural studies
T. Yamashita: Study design, Manuscript preparation
T. Kobayashi: Electrophysiological advice
N. Tohse: Electrophysiological advice, Manuscript preparation
ICMJE Conflict of Interest:
None declared
©2012 British Editorial Society of Bone and Joint Surgery. This is an open-access article distributed under the terms of the Creative Commons Attributions licence, which permits unrestricted use, distribution, and reproduction in any medium, but not for commercial gain, provided the original author and source are credited.