Abstract
Objectives
To evaluate the neck strength of school-aged rugby players, and to define the relationship with proxy physical measures with a view to predicting neck strength.
Methods
Cross-sectional cohort study involving 382 rugby playing schoolchildren at three Scottish schools (all male, aged between 12 and 18 years). Outcome measures included maximal isometric neck extension, weight, height, grip strength, cervical range of movement and neck circumference.
Results
Mean neck extension strength increased with age (p = 0.001), although a wide inter-age range variation was evident, with the result that some of the oldest children presented with the same neck strength as the mean of the youngest group. Grip strength explained the most variation in neck strength (R2 = 0.53), while cervical range of movement and neck girth demonstrated no relationship. Multivariable analysis demonstrated the independent effects of age, weight and grip strength, and the resultant model explained 62.1% of the variance in neck strength. This model predicted actual neck strength well for the majority of players, although there was a tendency towards overestimation at the lowest range and underestimation at the highest.
Conclusion
A wide variation was evident in neck strength across the range of the schoolchild-playing population, with a surprisingly large number of senior players demonstrating the same mean strength as the 12-year-old mean value. This may suggest that current training regimes address limb strength but not neck strength, which may be significant for future neck injury prevention strategies. Age, weight and grip strength can predict around two thirds of the variation in neck strength, however specific assessment is required if precise data is sought.
Article focus
Evaluation of the neck strength of school-aged rugby players
The determination of a proxy measure that could be used practically by coaches and medical staff in order to determine individual neck strength
Key messages
It is assumed that adequate neck strength is protective against neck injury in rugby players. We found the neck strength of school aged rugby players to generally increase with age, but to vary widely, which may be important for future injury prevention strategies and in developing training interventions
Specific neck strength can be predicted via the model presented with measures of age, weight and grip strength
Strengths and limitations
This is the first study to directly assess the neck strength of school-aged rugby players, with the relatively large size of the sample and the range of assessment parameters being particular strengths of the study
A limitation is the assumed but unsubstantiated causal association between neck strength and severity of injury to the neck in the sport of rugby. This is recommended as a focus for future research
Introduction
Professional rugby union carries a higher incidence of injury compared with other sports,1 most of which results from the contact phases of the game.1-6 Increases in strength and speed, and therefore also power and momentum, are considered to contribute to the rise in the injury rate of professional players.2,3 A similar trend is suspected in rugby played in schools. Due to the lack of appropriate data collection systems this has not been substantiated,7 although two recent studies do support the assertion of an increasing injury problem within youth rugby.2,8
Catastrophic injury is rare in rugby, with an injury risk reported as 0.8/1000 playing hours in the professional game.2 Serious neck and spinal injuries are also thought to be rare in the youth game.9 However, every neck injury is of significance due to the profound morbidity that may result. A recent survey of admissions to spinal injury units in the United Kingdom demonstrated that serious neck injuries continue to occur in school rugby players, albeit with a low frequency.10 Data from the spinal injuries unit in Glasgow suggests an alarming spike in the rates of catastrophic spinal injury in the Scottish schoolchild rugby playing population.11 Less severe neck injuries are more common in the game and may account for up to 30% of all reported injuries.8
The cervical spine is repeatedly exposed to potentially injurious forces, which are usually attenuated by controlled spinal motion through the cervical musculature, ligaments and inter-vertebral discs.12 Pinsault, Anxionnaz and Vuillerme13 speculated that repeated violent impacts on the cervical spine impair muscle function and proprioception, which is important for the initiation of spinal reflexes that act to stabilise and protect the cervical spine. Strengthening of the neck is considered to be a preventative measure against neck injury in physically demanding contact sports such as rugby, where increased neck strength may be useful.14,15 Development of the cervical musculature is advocated as beneficial in school grade players to protect against injury. However, the neck strength of youth rugby players has not been documented, nor the extent to which neck strength changes with physical maturity.
The primary aim of this study was to evaluate the neck strength of school-aged rugby players. Secondary aims were to investigate and define the relationship of neck strength with proxy physical measures with a view to predicting neck strength.
Materials and Methods
Study population and design
An observational cohort study was performed to investigate the specific neck strength of rugby playing schoolchildren. All rugby-playing schoolboys between the ages of 12 and 18 at three Scottish schools were invited to take part. Participation was entirely voluntary. Signed consent was obtained from the pupil after documented consultation with their parent or guardian. Regional Ethics Committee approval was received for this study. A total of 382 boys were assessed.
Neck strength assessment
Maximal voluntary isometric cervical muscle strength was assessed with a bespoke device based on a 300 kg load cell (Tedea-Huntleigh, Cardiff, United Kingdom) and TR150 Portable Strain Display Load Cell/Force Transducer (Honeywell, Marlton, New Jersey) employing a testing protocol previously reported.14 Any maximal muscle assessment contains the potential for a degree of muscle soreness following the test. This was minimised by limiting rotational movements and testing the muscle contraction along anatomical lines. Additionally the test was ended at the moment muscle force was measured to peak and thus sustained muscle contractions were limited. An average of three maximal peak force measurements was recorded in each test. The test was performed subjecting the neck to manual controlled linear incremental loading in the absence of pain or neurological symptom (that stopped the test) the head was held in the neutral anatomic position at all times throughout the test. The peak isometric force was recorded at the point of head movement.
Isometric cervical muscle testing is well validated in adult populations.16-18 We incorporated additional validation within this cohort; the intra-observer variability and repeatability formed excellent correlation coefficients (r = 0.9, p < 0.001) that has been reported separately.19
Additional measures
Height (Leicester Height Measure; SECA, Birmingham, United Kingdom), weight (medical grade mechanical flat scales; SECA) and grip strength (JAMAR hydraulic hand dynamometer; Sammoms Preston, Bolingbrook, Illinois) were assessed in the entire population. Cervical range of movement (ROM) (Cervical Range of Motion Instrument; Performance Attainment Associates, Minneapolis, Minnesota) and neck circumference (standard tape measure) was assessed in a subgroup of 166 boys aged 15 to 18 years. For efficiency of testing in large numbers at single sittings we decided not to assess these additional factors in the youngest boys (aged 12 to 14 years).
Statistical analysis
Data were analysed using SPSS v14 (SPSS Inc., Chicago, Illinois) and GraphPad v5 (GraphPad Software, La Jolla, California). Data were checked for normality, and following this parametric tests used. One way analysis of variance (ANOVA) was used to assess variance in continuous measurements across groups (neck strength, ROM, height, weight, body mass index across age groups). A Bonferroni correction was applied to reduce the chance of a type I error associated with multiple testing. The data was reported as means with standard deviations (sd) as a measure of dispersion.
Pearson correlation coefficients were reported for the bivariate correlation of continuous variables (neck strengths with age, height and weight). Multivariable modelling was achieved using multiple linear regression. Potential predictive variables were selected if their bivariate significance was p < 0.10 to account for the possibility of variables achieving statistical significance once the confounding influence of another variable was controlled. Once this model was performed, a second definitive model was constructed with the variables that had reached significance on the first model. This was to achieve the most predictive model, with the fewest predictive variables. The overall significance level was selected as p < 0.05 and two-tailed p-values are reported throughout. The performance of the model was assessed using a Bland and Altman plot.20
Results
Data were collected for 382 schoolchildren. Mean height, weight and grip strength are reported in Table I. Isometric neck extension strength increased with age (p < 0.001, ANOVA), although wide variations within age groups were apparent (Fig. 1).
Table I
Mean height, weight and grip strength by age grade
Age group (yrs) | Number | Mean (sd) height (cm) | Mean (sd) weight (kg) | Mean (sd) grip strength (kg) | Mean (sd) neck extension strength (kg) |
---|---|---|---|---|---|
12 | 54 | 155 (7.6) | 48 (9.2) | 23 (3.9) | 18 (3.1) |
13 | 68 | 163 (7.8) | 54 (10.8) | 27 (5.0) | 21 (3.9) |
14 | 64 | 170 (7.5) | 61 (9.8) | 33 (8.1) | 25 (5.7) |
15 | 66 | 175 (7.0) | 68 (11.4) | 38 (6.9) | 28 (6.0) |
16 | 54 | 179 (7.5) | 72 (10.2) | 42 (8.1) | 30 (6.0) |
17 | 59 | 180 (6.2) | 76 (12.8) | 43 (8.1) | 32 (6.3) |
18 | 17 | 182 (8.1) | 84 (14.9) | 46 (6.9) | 34 (8.1) |
Total | 382 |
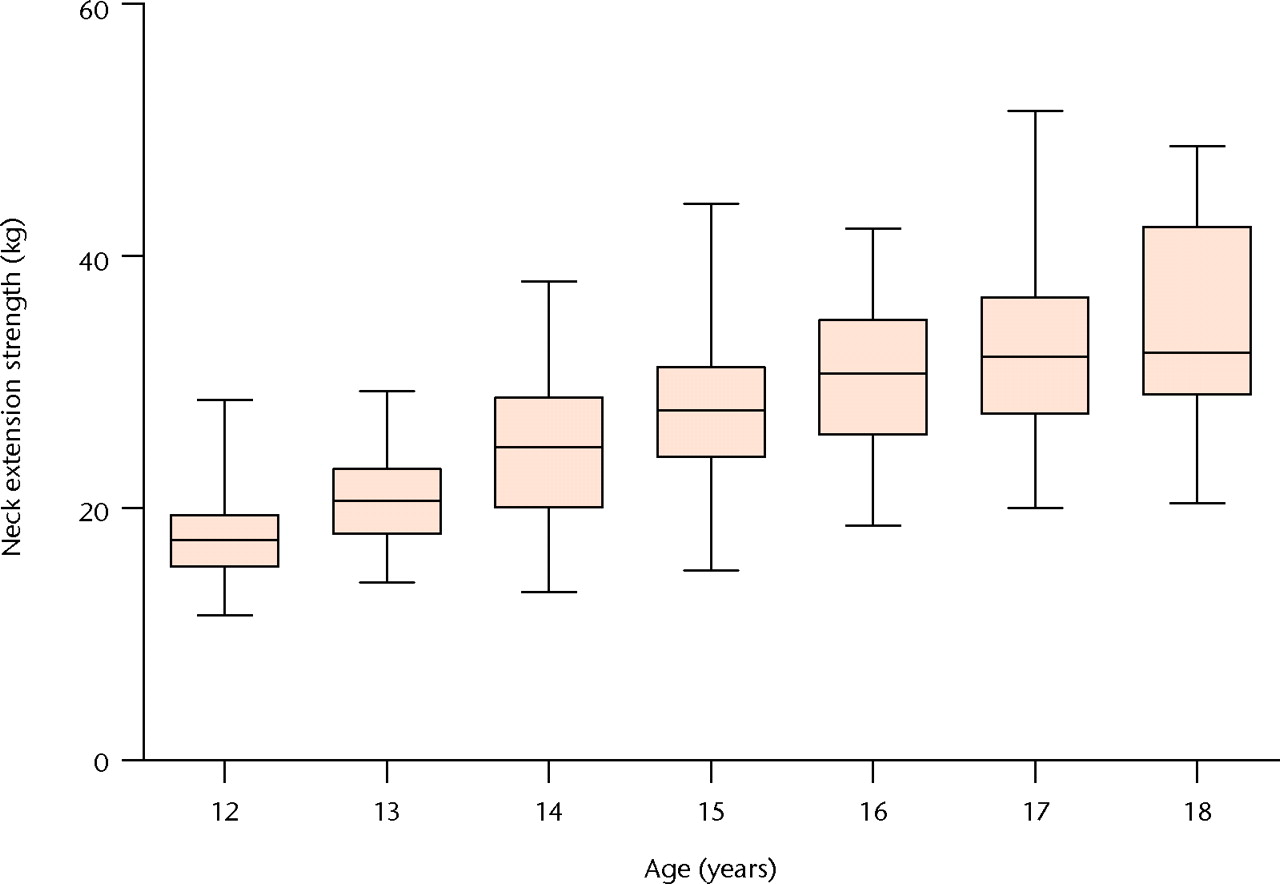
Fig. 1
Box plot showing neck extension strength by age group. The boxes represent the median value and interquartile range, and the whiskers represent the range of data.
The mean neck extension strength increased with age, from 18 kg (sd 3.1) for the 12-year-old group to 34 kg (sd 8.1) for the 18-year-old group. However at each age group there was a large range of between 15 kg and 30 kg. As illustrated in Figure 1, there were some 18-year-old participants who were weaker than the mean value for the 12-year-old group. This demonstrates that neck strength does not increase uniformly with growth. Further parameters were assessed in the subgroup of 166 boys aged between 15 and 18 years (Table II). Circumference of the neck was similar in the different age groups. Cervical ROM for flexion-extension and side flexion increased with age (p = 0.007 and p = 0.025, respectively), but no statistically significant change was seen in rotational ROM (p = 0.902).
Table II
Mean cervical range of movement (ROM) and neck circumference of participants aged between 15 and 18 years
Mean (sd) ROM (°) | |||||
---|---|---|---|---|---|
Age group (yrs) | Number (n = 166) | Mean (sd) neck circumference (cm) | Left/right rotation | Side flexion | Flexion/extension |
15 | 36 | 35.2 (3.3) | 70.3 (6.6) | 41.0 (6.5) | 73.8 (12.4) |
16 | 54 | 33.1 (3.0) | 70.4 (9.0) | 43.0 (6.6) | 78.2 (9.7) |
17 | 59 | 33.9 (3.2) | 71.3 (6.9) | 43.3 (5.9) | 76.1 (11.4) |
18 | 17 | 32.4 (3.0) | 70.9 (7.3) | 47.6 (7.6) | 93.2 (9.4) |
p-value | 0.006 | 0.902 | 0.007 | 0.025 |
Isometric neck extension strength correlated with age, weight and grip strength and modestly with height. Further measures of cervical range of motion and neck circumference did not correlate with cervical extension (Table III, Fig. 2).
Table III
Bivariate correlation of neck extension strength with anthropometric measures and range of movement (ROM) characteristics
Characteristic | Pearson correlation (r) | p-value |
---|---|---|
Age | 0.668 | < 0.001 |
Height | 0.452 | < 0.001 |
Weight | 0.717 | < 0.001 |
Grip strength | 0.731 | < 0.001 |
ROM* flexion/extension | 0.140 | 0.074 |
ROM side flexion | 0.012 | 0.115 |
ROM rotation | -0.003 | 0.971 |
Neck circumference | -0.048 | 0.544 |
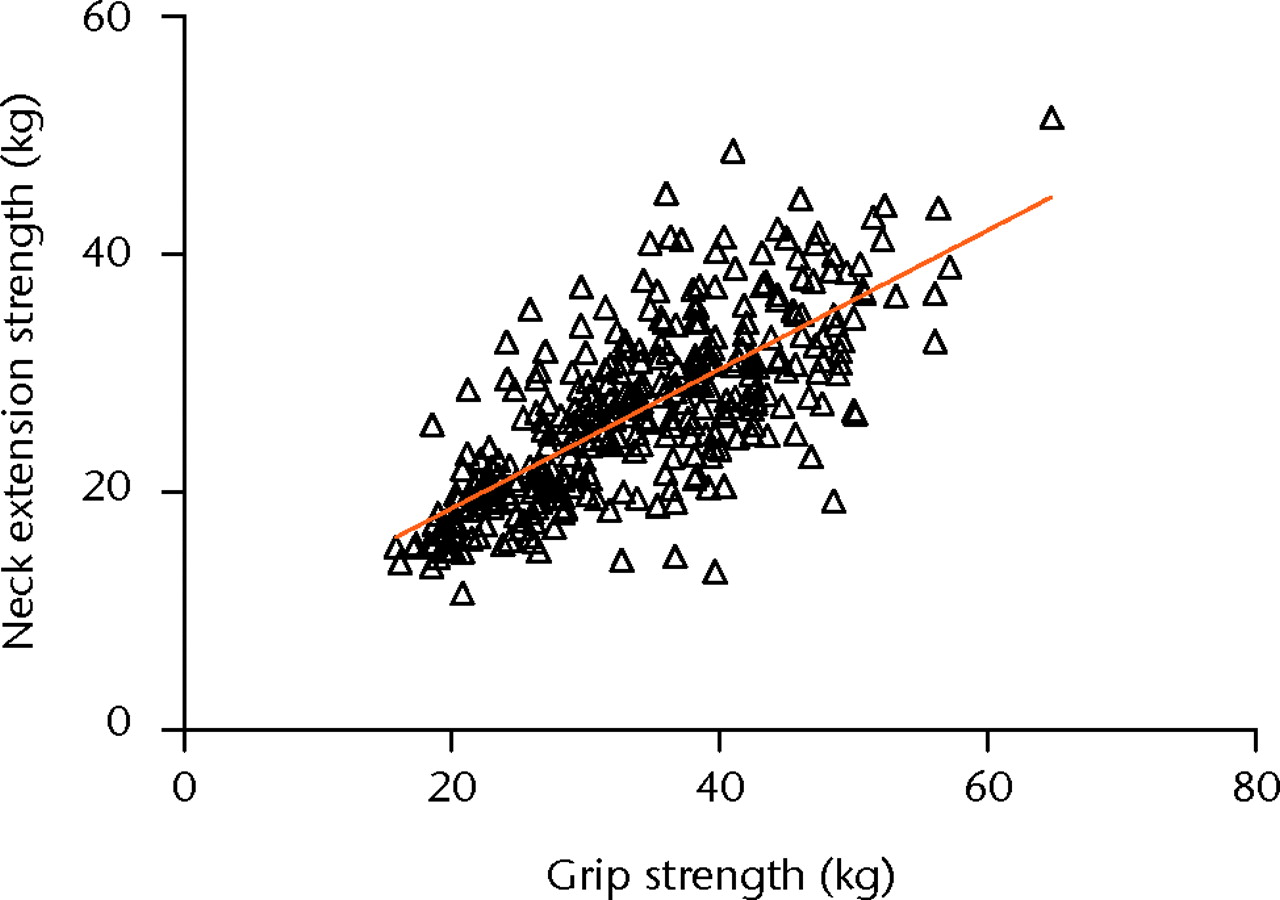
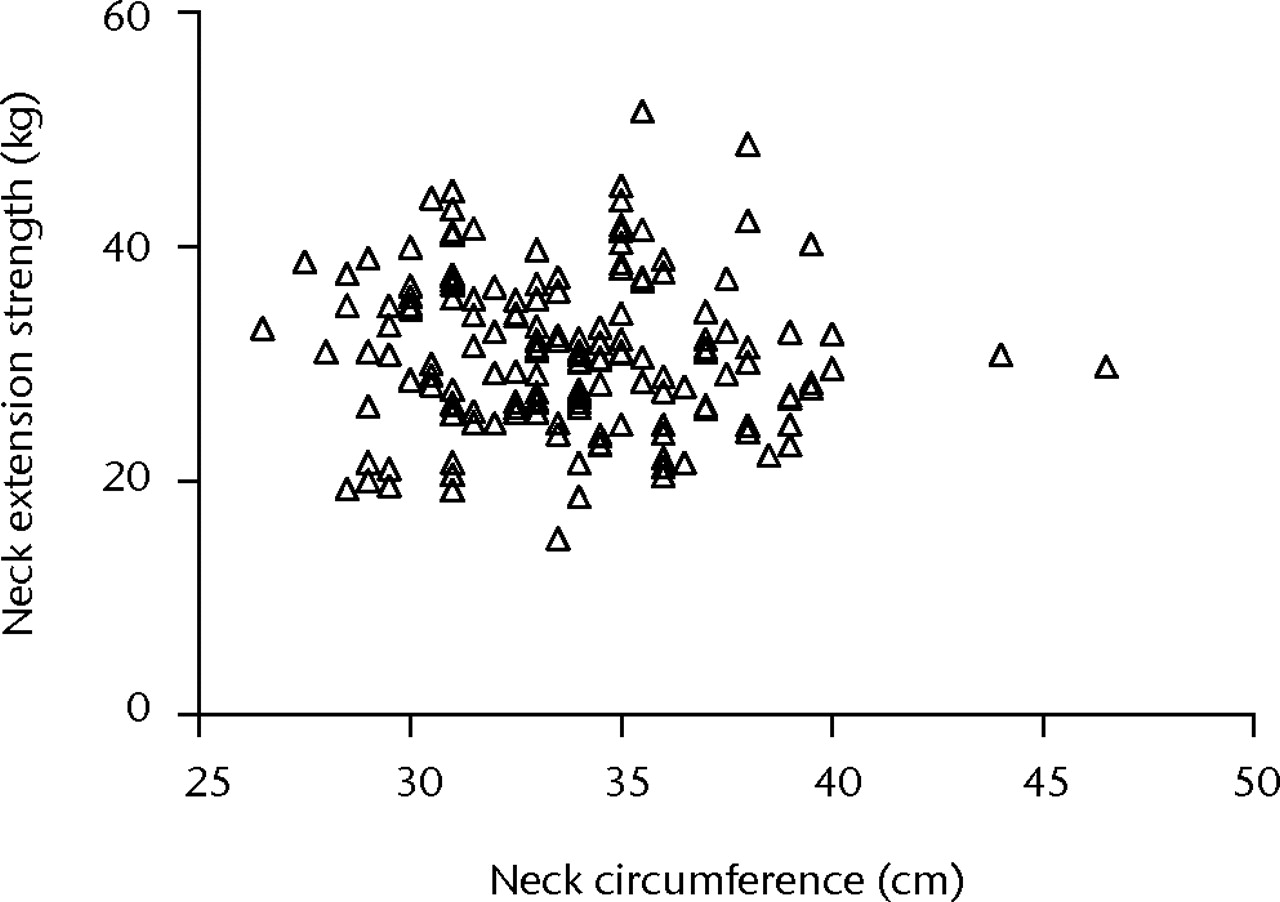
Figs. 2a - 2b
Plots showing bivariate correlations of neck extension strength versus a) grip strength and b) neck circumference.
Of the neck strength proxy measures, grip strength explained around half the variation in neck extension strength (R2 = 0.53) whereas neck circumference demonstrated no relationship (Fig. 2). A multivariable analysis demonstrated the independent effects of age, height, weight and grip strength on neck extension strength. Height was not a significant predictor of neck strength (p = 0.672) and was therefore removed (Table IV).
Table IV
Multivariable linear regression of predictors of neck strength (R2 = 0.621) (CI, confidence interval)
Variable | B (95% CI) | Beta | p-value |
---|---|---|---|
Constant | -6.42 | ||
Age | 0.89 (0.46 to 1.31) | 0.21 | < 0.001 |
Weight | 0.16 (0.11 to 0.22) | 0.32 | < 0.001 |
Grip strength | 0.28 (0.19 to 0.37) | 0.35 | < 0.001 |
The resultant model explained 62.1% of the overall variance in neck strength (Fig. 3). The model performed well for the majority of players however; there were outliers in the predictive model at extremes of neck extension strength (Fig. 4). It tended to over predict extension strength at the lowest of the range and under predict at the highest extremes.
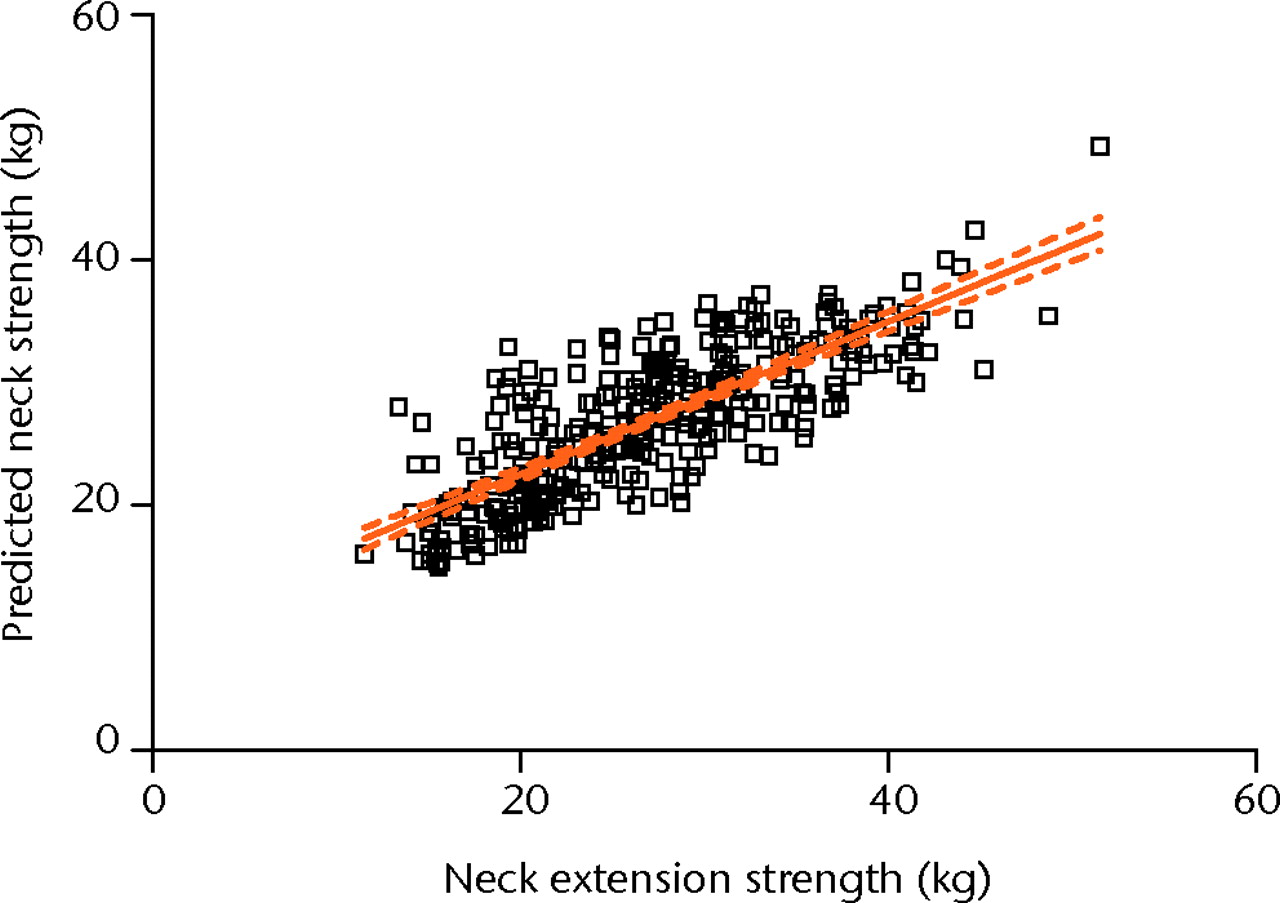
Fig. 3
Bivariate correlation of predicted versus actual neck extension strength based on multiple linear regression model R2 = 0.621.
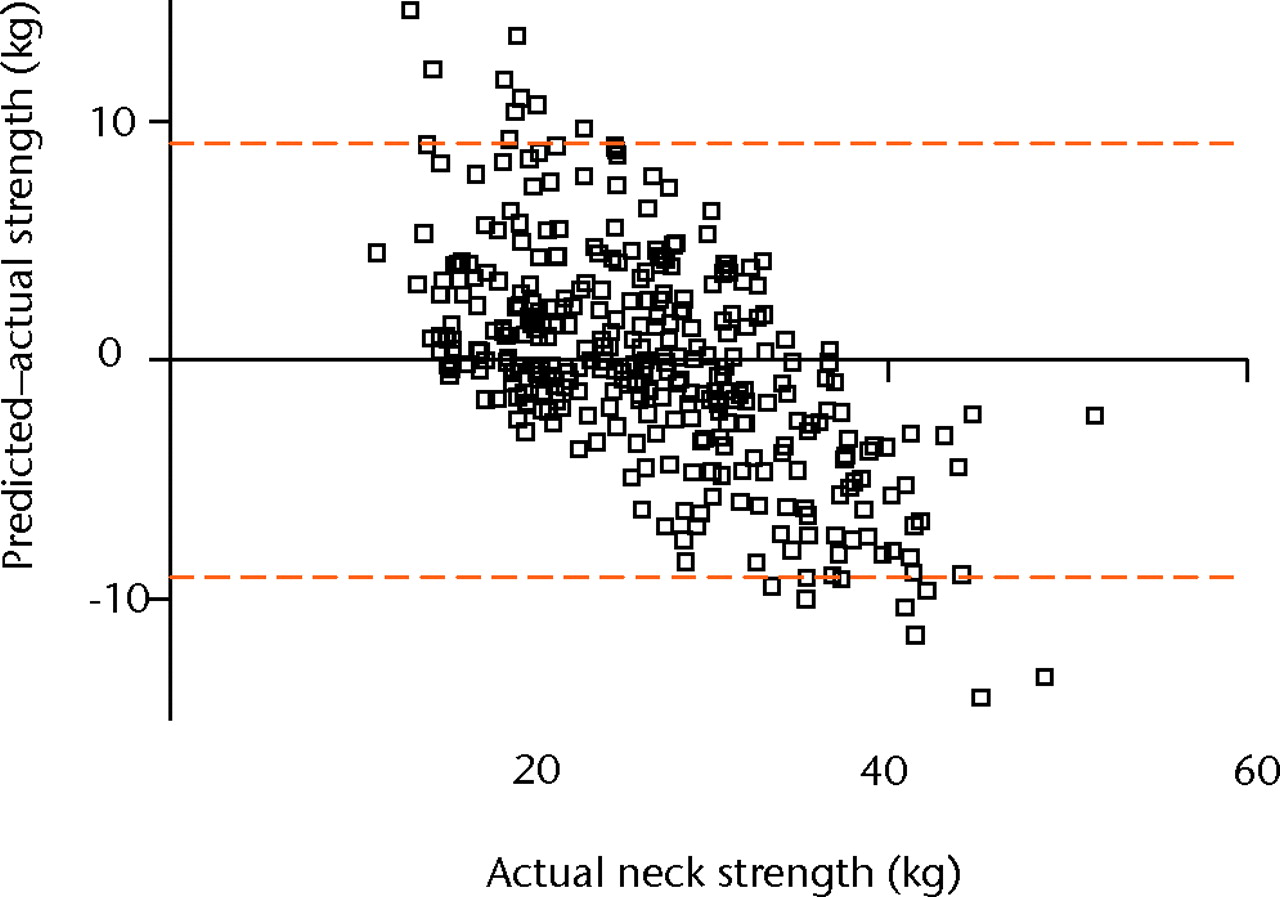
Fig. 4
Bland-Altman plot showing agreement between predictive model and actual neck extension strength (error bars represent the 95% confidence intervals (1.96×sd)).
Discussion
The results of this study demonstrate the progressive changes in isometric neck extension strength with age, and the relationship with bodyweight and grip strength in school-aged rugby players. A large variation of neck strength was evident at each age group, and the neck strength of a number of older participants was equivalent to that of the mean value for the 12-year-old group. This may suggest that current training regimes concentrate more on building limb strength than neck strength.
Neck strength is thought to be of key importance in the prevention of neck injuries through the modulation of excessive translational and shear forces (tackle) or excessive impact and shear force when the athlete’s head is forced into the playing surface.21 It is thought that when these forces are not dissipated effectively injury may occur.14 Despite this assumption, little is known as to the specific strength of the cervical musculature in schoolboy rugby players.
Two recent papers explore the specific assessment of injury in schoolboy rugby players. Haseler, Carmont and England2 described an injury surveillance programme over a single season at a community rugby club representing some 210 male players with an age range from under-9 to under-17 teams. Relatively few injuries were reported (24 per 1000 playing hours), although a significantly higher number of moderate and severe injuries occurred in the under-16 and -17 teams compared with the more junior sides. Head injuries along with shoulder and knee injuries were the most frequently reported site of injury, but the authors acknowledged that the study was underpowered and the numbers too small to investigate the differences in these rates. McIntosh et al8 reported the incidence and risk factors of head and neck injury in Australian youth rugby players, suggesting that age and player position (forwards, especially the front row) were related to head and neck injury risk.
The testing of an individual’s neck strength requires specialist equipment and is relatively labour intensive. As such, proxy measures of global strength are advocated as pragmatic guides to estimate this. Suggested measures include grip strength and muscle cross-sectional area. Interestingly, in this study, grip strength accounted for only around 50% of the variation of specific neck strength across the cohort (Fig. 2a), while circumference of the neck, as an estimate of cross sectional area, was not associated with maximal isometric strength (r = 0.05, p = 0.55) (Table III, Fig. 2b). The range of neck flexion/extension and side flexion increased with age, but rotation remained constant.
Maclean and Hutchison,10 in a review of adolescent rugby-related admissions to United Kingdom spinal injuries units recently reported that the contact phases (specifically the tackle and scrum) of the game accounted for the majority of serious neck injuries, highlighting the importance perhaps of adequate musculature to protect this potentially vulnerable region.14 The regression model provides a useful approximation of a player’s neck strength without the need for specialist assessment, derived from measures of age, grip strength and body weight (Table IV). Measures of height, neck circumference, ROM and neck side flexion strength were not significant predictors of neck extension strength. This model demonstrated reasonable accuracy, explaining around two thirds of the variation in neck strength by associated morphological parameters. The tendency to over- or underpredict at the extreme ranges suggests that specific assessment of the neck should perhaps be employed in situations where precise measurement is particularly important, such as in players in positions more susceptible to injury (e.g. front row forwards).
This study is the first to provide data for global neck strength in adolescent rugby players. A large variation was found within each age group and a substantial number were found to have neck extensor strength comparable to that of the 12-year-old mean, suggesting that current training regimes concentrate on limb musculature and place insufficient emphasis on the neck musculature. Age, weight and grip strength predicted 62% of the variation in neck strength by logistic regression modelling that supports the use of these parameters for matching player groups, however specific testing of individual neck strength is advocated if precise information is required.
The authors would like to thank the pupils and staff at the three schools tested and to Dr J. Robson and Mr N. Rennie of the Scottish Rugby Union for their ongoing support and encouragement with this project.
1 Brooks JH , KempSP. Injury-prevention priorities according to playing position in professional rugby union players. Br J Sports Med2011;45:765–775.CrossrefPubMed Google Scholar
2 Haseler CM , CarmontMR, EnglandM. The epidemiology of injuries in English youth community rugby union. Br J Sports Med2010;44:1093–1099.CrossrefPubMed Google Scholar
3 Fuller CW , AshtonT, BrooksJH, et al.Injury risks associated with tackling in rugby union. Br J Sports Med2010;44:159–167.CrossrefPubMed Google Scholar
4 Garraway WM , LeeAJ, MacleodDA, et al.Factors influencing tackle injuries in rugby union football. Br J Sports Med1999;33:37–41.CrossrefPubMed Google Scholar
5 Fuller CW , SmithGL, JungeA, DvorakJ. The influence of tackle parameters on the propensity for injury in international football. Am J Sports Med2004;32(Suppl):43S–53S.CrossrefPubMed Google Scholar
6 Hawkins RD , FullerCW. An examination of the frequency and severity of injuries and incidents at three levels of professional football. Br J Sports Med1998;32:326–332.CrossrefPubMed Google Scholar
7 Nicol A , PollockA, KirkwoodG, ParekhN, RobsonJ. Rugby union injuries in Scottish schools. J Public Health (Oxf)2011;33:256–261.CrossrefPubMed Google Scholar
8 McIntosh AS , McCroryP, FinchCF, WolfeR. Head, face and neck injury in youth rugby: incidence and risk factors. Br J Sports Med2010;44:188–193.CrossrefPubMed Google Scholar
9 Emery CA . Injury prevention in paediatric sport-related injuries: a scientific approach. Br J Sports Med2010;44:64–69.CrossrefPubMed Google Scholar
10 Maclean JG, Hutchison JD. Serious neck injuries in U19 rugby union players: an audit of admissions to spinal injury units in Great Britain and Ireland. Br J Sports Med 2011:Epub. Google Scholar
11 Allan DB, Brydone AS. Spinal injuries in Scottish youth rugby. Procs COMOC, Glasgow, 2010. Google Scholar
12 Torg JS , Ramsey-EmrheinJA. Suggested management guidelines for participation in collision activities with congenital, developmental, or postinjury lesions involving the cervical spine. Med Sci Sports Exerc1997;29:256–272.CrossrefPubMed Google Scholar
13 Pinsault N , AnxionnazM, VuillermeN. Cervical joint position sense in rugby players versus non-rugby players. Phys Ther Sport2010;11:66–70.CrossrefPubMed Google Scholar
14 Peek K , GathererD. The rehabilitation of a professional Rugby Union player following a C7/T1 posterior microdiscectomy. Phys Ther Sport2005;6:195–200. Google Scholar
15 Lark SD , McCarthyPW. The effects of a single game of rugby on active cervical range of motion. J Sports Sci2009;27:491–497.CrossrefPubMed Google Scholar
16 Garcés GL , MedinaD, MilutinovicL, GaravoteP, GueradoE. Normative database of isometric cervical strength in a healthy population. Med Sci Sports Exerc2002;33:464–470.CrossrefPubMed Google Scholar
17 Jordan A , MehlsenJ, BülowPM, OstergaardK, Danneskiold-SamsøeB. Maximal isometric strength of the cervical musculature in 100 healthy volunteers. Spine (Phila Pa 1976)1999;24:1343–1348.CrossrefPubMed Google Scholar
18 Chiu TT , SingKL. Evaluation of cervical range of motion and isometric neck muscle strength: reliability and validity. Clin Rehabil2002;16:851–858.CrossrefPubMed Google Scholar
19 Hamilton DF, Simpson HR, Gatherer D. Repeatability and inter-tester reliability of a new tool to assess isometric neck strength in adolescents. Procs Physiotherapy Research Society, Middlesbrough 2010. Google Scholar
20 Bland JM , AltmanDG. Statistical methods for assessing agreement between two methods of clinical measurement. Lancet1986;1:307–310.PubMed Google Scholar
21 Boden BP , JarvisCG. Spinal injuries in sports. Neurol Clin2008;26:63–78.CrossrefPubMed Google Scholar
Funding statement:
This research received no specific grant from any funding agency in the public, commercial or not-for-profit sectors.
Author contributions:
D. F. Hamilton: Study design, Data collection, Data analysis and interpretation, Writing the paper, Approval of final text
A. H. R. W. Simpson: Study design, Data collection and interpretation, Review of the draft for intellectual content, Approval of final text
D. Gatherer: Data collection and interpretation, Review of the draft for intellectual content, Approval of final text
P. J. Jenkins: Data analysis and interpretation, Review of the draft for intellectual content, Approval of final text
R. W. Nutton: Study design, Data collection and interpretation, Review of the draft for intellectual content, Approval of final text
J. G. B. Maclean: Study design, Data collection and interpretation, Review of the draft for intellectual content, Approval of final text
J. D. Hutchison: Study design, Data collection and interpretation, Review of the draft for intellectual content, Approval of final text
ICMJE Conflict of Interest:
None declared
©2012 British Editorial Society of Bone and Joint Surgery. This is an open-access article distributed under the terms of the Creative Commons Attributions licence, which permits unrestricted use, distribution, and reproduction in any medium, but not for commercial gain, provided the original author and source are credited.