Abstract
In a global environment of rising costs and limited funds, robotic and computer-assisted orthopaedic technologies could provide the means to drive a necessary revolution in arthroplasty productivity. Robots have been used to operate on humans for 20 years, but the adoption of the technology has lagged behind that of the manufacturing industry. The use of robots in surgery should enable cost savings by reducing instrumentation and inventories, and improving accuracy. Despite these benefits, the orthopaedic community has been resistant to change. If the ergonomics and economics are right, robotic technology just might transform the provision of joint replacement.
A revolution is needed in joint replacement productivity. The orthopaedic profession and industry of arthroplasty may shortly face an inflection point as the cost of delivering the service continues to rise in a climate of global austerity. The purchasers of health care, both state- and insurer-funded, must cut their spending on musculoskeletal conditions and in every other sector of health care, as the wealth of many nations shrinks in real terms. Hence the need for reform, as has already been delivered in most other industries. The revolution? More, and better, joint replacements for less cost. Robotic and computer-assisted orthopaedic technologies could be the vehicle for this change.
It is 20 years since robots were first used to operate on humans, on both the prostate and in joint replacement.1,2 In the same time frame, their use in manufacturing industries has become almost universal. Robotic technologies were one of the keys to unlocking the productivity conundrum. Robots can now perform all the tasks akin to bone preparation and implant insertion with a level of reliability and speed that cannot be matched by humans. By doing so they have reduced costs and improved productivity, making skilled artisans less effective, and replacing many of them altogether. Can this robotic platform of technology transform both the profession and industry of joint replacement? Certainly, a seven-year follow-up of a robotic surgery prospective randomised controlled trial shows continued benefit from accurate surgery giving some grounds for optimism.3 Alternatively, the sun may again go down on this embodiment of technology. Several forces are at work, changing the way operations are performed; robots are not the only threat to the surgeon artisan’s primacy, so the short-term future is unclear.
While free choice is not always available within state-funded systems, patients as consumers are attracted to technologies whose marketing strategy includes claims about the impact of accuracy on clinical outcome. However, this success, while measurable in commercial earnings, is not yet detectable by any of the normal metrics of cost-effectiveness in surgery.
Robots in medicine
Today, robotic technology in medicine is still limited. Master-slave robotic assistance in radical prostatectomy is a key part of thousands of procedures each year, delivering levels of accuracy that cannot be matched by conventional instrumentation. However, the clinical cost-effectiveness argument for radical prostatectomy has not yet been won.4 Nevertheless, the financial success of the Da Vinci robotic system (Intuitive Surgical Inc., Sunnyvale, California, USA) in the prostate world, despite its enormous cost both in capital outlay and running expense, is evidence that such systems can establish a position in the market as the business is highly profitable.5 The technology either deskills the complex procedure, or upskills the surgeon, depending on the point of view. It has created its demand by marketing its perceived benefits of more precise surgery.
Meanwhile in arthroplasty, robotic technology is not yet profitable, but offers three distinct advantages: detailed planning, reliable bone preparation and the ability to sculpt bone in ways that cannot be achieved using conventional tools. Conventional arthroplasty surgery has not remained static - the range of devices has expanded while the interval between each size has decreased, increasing inventories several times over. Instrumentation sets have become more complex, allowing the surgeon greater flexibility but at the cost of more trays of instruments. Meeting sterilisation standards requires a longer turnaround, often off-site, which increases the cost for the hospital and the manufacturers, who are now considering any alternative to providing the huge range of implants and number of instruments required for each case, especially as the number of procedures proliferates.
Image-based planning
All modern arthroplasty systems have now adopted, or are soon to offer, a three-dimensional (3D) planning-based approach, which can be implemented using conventional instruments, with robotic assistance, or by using a patient-specific instrumentation (PSI) model.6 This 3D planned approach has always been essential for robotic systems, but improvements in computing power have made the process fast and commercially possible in the PSI model. Axial scans from either CT or MRI are reformatted to create a 3D model of the patient’s osteoarticular anatomy. This model informs the choice of implant size, shape and position, which are optimised in a planning phase that requires the surgeon’s approval. The surgeon can also select the prosthesis that will best fit the patient’s shape from the range of devices offered by the planning software, although currently most PSI offerings are device-specific. In all cases, whether PSI or robotic, the presence of a detailed plan makes substantial savings by reducing the inventory and instrumentation needed for the case. Going one step further and making the prosthesis specifically for the patient, fitting the component to the patient’s own anatomy, and manufacturing it in a ‘just in time’ process seems like the ultimate solution: this is actually practised by certain companies using both PSI and robotics. This process eliminates the cost of holding a large stock of prostheses, so could bring economic benefits. Currently, however, these are positioned as high-cost items out of reach of any routine tariff.
Intra-operative systems
Robotic systems in clinical use today are in two major forms. The original ‘Robodoc’ (Currexo, Freemont, California, USA) is an active robot that is in use in South Korea. This requires the patient to be attached solidly to the robot, which then machines the bones precisely. This approach, first implemented in humans in the early 1990s, renders the surgeon entirely superfluous for the bone preparation, and is clinically successful in the fields of hip7 and knee8 replacement. It has not been widely adopted, perhaps because of the perceived lack of autonomy felt by the surgeon, who has to stand by and watch the procedure.
There are two semi-active robots in clinical use, with similar implementations. Both the Sculptor Robotic Guidance Arm (RGA, Stanmore Implants Worldwide, Elstree, UK) (Fig. 1) and the Robotic Arm Interactive Orthopaedic System (RIO, MAKO Surgical Corp., Fort Lauderdale, Florida, USA) are based upon an active constraint approach.9 Intra-operatively, the bone being sculpted is fixed to a navigation aid, either optical or mechanical. The process of registration then takes place (Fig. 2); multiple points on the bone are touched by the surgeon who uses a two-millimetre ball-tip inserted into the end of the robotic guidance arm. The points are then contour-mapped to the 3D model and checks made to ensure an acceptable match before proceeding. The constraint-boundaries are activated and the surgeon begins the process of bone sculpting, under ‘active constraint’10 or ‘haptic’ feedback.11 The 3D image of the process is viewed on a colour monitor attached to the robot. By a process of ‘painting’ the screen, the surgeon knows when the required amount of bone is resected. As the boundary is approached, the resistance increases, preventing surgical error, and if it is crossed, the robot will automatically stop functioning until the arm is moved back within the desired zone. This approach is more accurate than conventional surgery and has favourable short-term clinical results.12,13
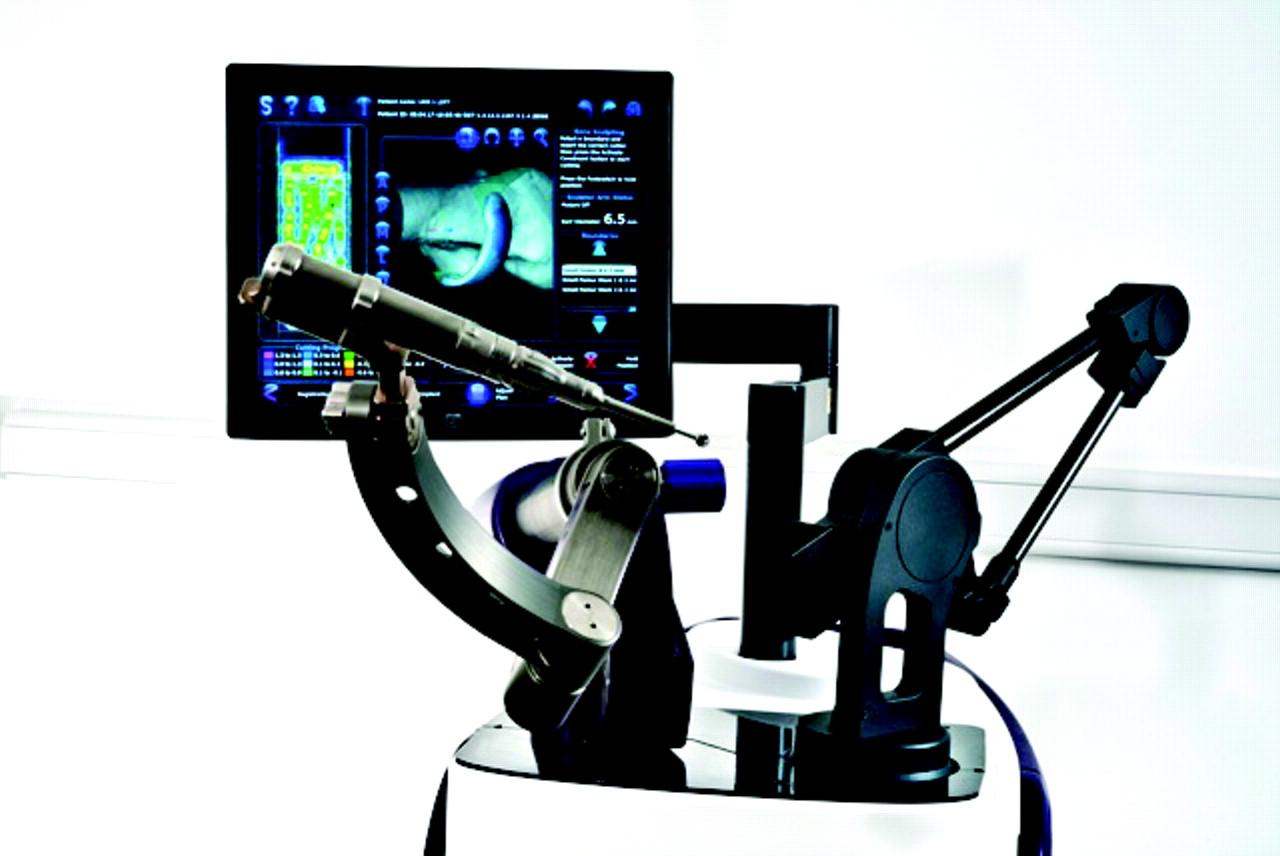
Fig. 1
The Stanmore Sculptor Robotic Guidance Arm, which assists with bone preparation for the implantation of patient-specific implants.
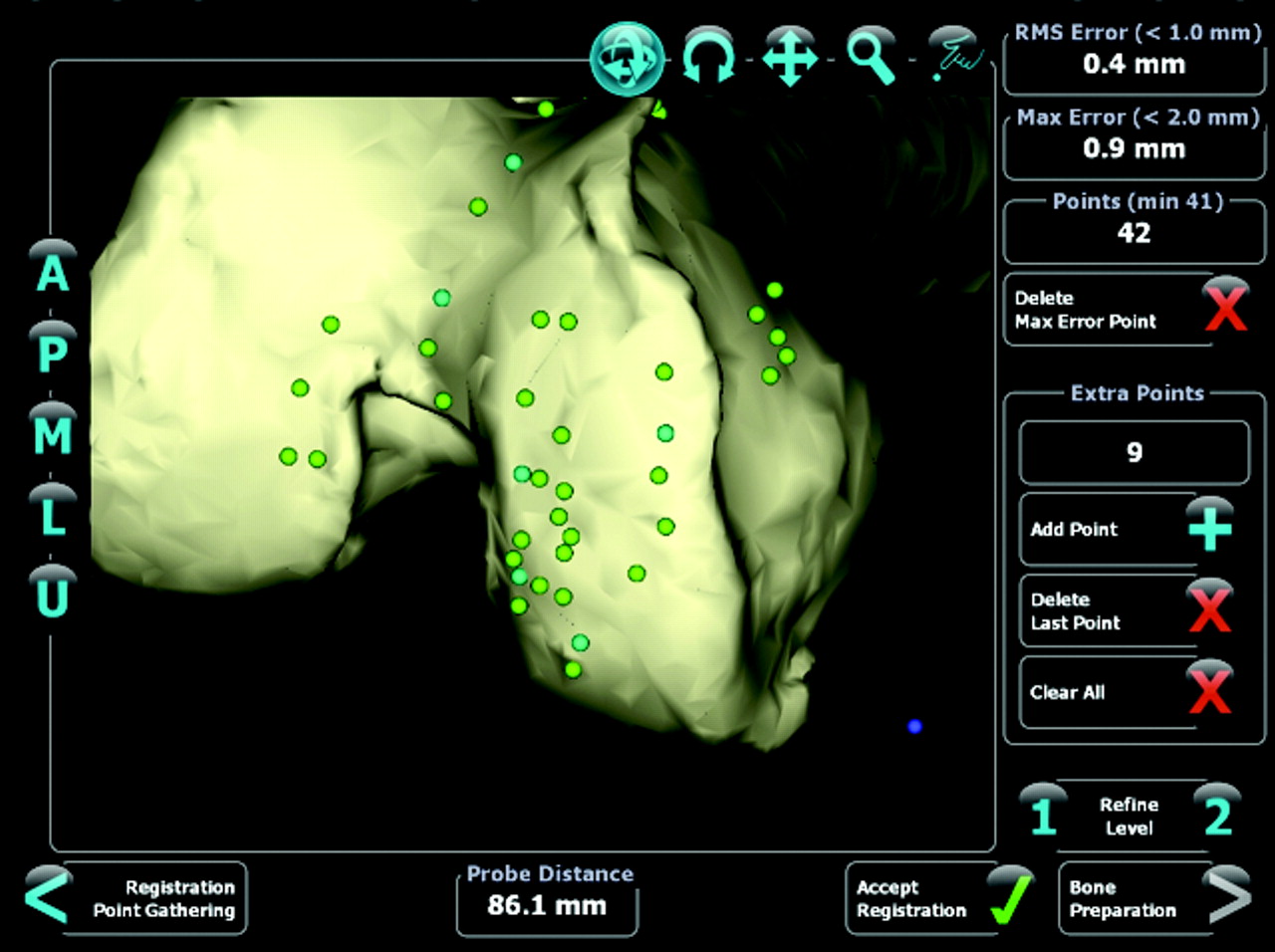
Fig. 2
Example of registration during total knee replacement surgery.
This image-based robotic approach is not limited to unicompartmental knee replacement. The RIO system offers medial, lateral and patellofemoral options, and now also offers robotic control of acetabular cup placement.14 The technology is obviously suitable for any joint replacement task and is limited only by the development of applications.
Industry evolution
The pressing global need is one of improved productivity – a better clinical outcome for a lower cost. In some higher cost environments the robot is perceived as a way of reducing risk and improving function. This may be more important than any consideration of cost, so the patient or their insurers may pay more for the procedure. This is a plausible explanation for the financial success of the Da Vinci system in radical prostatectomy and may be one reason for the adoption of the RIO system across the USA. In the UK and Europe, the forces driving adoption of technologies may be different. The surgical skills needed for unicompartmental knee replacement have been well developed for decades, making the clinical case for expensive robots less pressing. Aggressive marketing strategies by private care providers, with budgets that can accommodate the capital expenditure of £1m needed to purchase the RIO robot, drive publicity campaigns to increase market share. The Sculptor RGA approach is different, and may be disruptive. Stanmore Implants Worldwide are positioning the Sculptor RGA as a low-cost or even no-cost robot that is free to use when associated with an implant sale, at a price that is competitive with conventional products that have to carry the cost-burden of inventory and instrumentation sets. This approach might be successful, but only if the ease, speed and reliability of use are adequate and pass the test of usability by the profession.
Professional resistance to change
Surgeons train for years, acquiring demanding surgical skills that they can then exploit to the benefit of their patients. Technology can help that process, enabling expert skills to be acquired rapidly and reliably even in technically demanding operations such as hip resurfacing.15 The joint replacement profession has been slow to accept such assistance, reflecting a conservative approach to acquiring the new skills required for the safe adoption of new procedures. This cautious approach to acquiring new skills has played a major role in the unfolding metal-on-metal hip debacle, where the shortcomings of a single device have been used to shield surgical inaccuracy from scrutiny. The profession has been even slower to adopt standards of accuracy and precision that are the norm in manufacturing. This resistance to change has been played out in different industries over centuries: some early resistors to mechanisation in England were deported to Australia16 where their descendants appeared more willing to embrace change. An eminent Australian surgeon, Andrew Shimmin, has been co-chairing a London-based interactive meeting, ‘The Great Debate’17 over the last four years. Surgical attendees have been polled regarding their resistance to change and perception of risks. Although self-selected, as delegates who have paid to attend the meeting, the emerging data are challenging. Surgeons who perform high volumes of operations appear more risk averse than those performing fewer cases, perhaps as they are more aware of their real complication rates; as expert surgeons they may have more to lose. This risk aversion is a natural brake on the adoption of the robotic and other novel technologies. The choice of which embodiment of technology is right for the surgeon, the hospital and the patient group is an exciting one, and one that needs debating. Which technology can provide the solution that is appropriate for your practice today?
Government, industry and profession
In the UK, and realistically in the rest of the developed world, every purchaser of health care is trying hard to improve productivity, but trying even harder to reduce costs. The revolution in industry that has driven productivity up and costs down has done so by hugely increasing the volume produced by a single skilled artisan. In cataract surgery, this revolution has taken place with a ten-fold change in productivity, a reduction in fee per case, but an increase in gross income for the surgeons. Everybody wins. That same revolution is coming to joint replacement. More operations each day, at higher standards, for less cost per procedure: if robotics cannot deliver this, it will not be part of the revolution and PSI technology might win instead. It is possible that the associated reduction in inventory and stock costs may drive adoption of this technology by healthcare providers across the world.
This may be a period of change in our working lives, of increasing standards and harder work for less pay, but the end product will be better for the consumer. If we get it right as a profession, by embracing rising standards and increasing productivity, we may also protect or even increase our individual incomes. The embodiment of that change may be robotic.
1 Davies BL , HibberdRD, NgWS, TimoneyAG, WickhamJE. The development of a surgeon robot for prostatectomies. Proc Inst Mech Eng H1991;205:35–38.CrossrefPubMed Google Scholar
2 Paul HA , BargarWL, MittlestadtB, et al.Development of a surgical robot for cementless total hip arthroplasty. Clin Orthop Relat Res1992;285:57–66.PubMed Google Scholar
3 Henckel J, Alsop H, Cobb J. 7 year follow up of a PRCT of robot assisted unicompartmental knee arthroplasty. CAOS International; Seoul 13-16 June 2012. Google Scholar
4 Malcolm JB , FabrizioMD, BaroneBB, et al.Quality of life after open or robotic prostatectomy, cryoablation or brachytherapy for localized prostate cancer. J Urol2010;183:1822–1828. Google Scholar
5 Stynes T. Intuitive surgical 3Q profit rises 41% on stronger sales. Wall Street Journal, October 18, 2011. Google Scholar
6 Radermacher K , PortheineF, AntonM, et al.Computer assisted orthopaedic surgery with image based individual templates. Clin Orthop Relat Res1998;354:28–38.CrossrefPubMed Google Scholar
7 Bargar WL , BauerA, BörnerM. Primary and revision total hip replacement using the Robodoc system. Clin Orthop Relat Res1998;354:82–91.CrossrefPubMed Google Scholar
8 Siebert W , MaiS, KoberR, HeecktPF. Technique and first clinical results of robot-assisted total knee replacement. Knee2002;9:173–180.CrossrefPubMed Google Scholar
9 Davies BL , HarrisSJ, LinWJ, et al.Active compliance in robotic surgery; the use of force control as a dynamic constraint. :. Proc Inst Mech Eng H1997;211:285–292. Google Scholar
10 Jakopec M , HarrisSJ, Rodriguez y BaenaF, et al.The first clinical application of a "hands-on" robotic knee surgery system. Comput Aided Surg2001;6:329–339.CrossrefPubMed Google Scholar
11 Roche M , O'LoughlinPF, KendoffD, MusahlV, PearleAD. Robotic arm-assisted unicompartmental knee arthroplasty: preoperative planning and surgical technique. Am J Orthop (Belle Mead NJ)2009;38(2 Suppl):10–15.PubMed Google Scholar
12 Cobb J , HenckelJ, GomesP, et al.Hands-on robotic unicompartmental knee replacement: a prospective, randomised controlled study of the acrobot system. J Bone Joint Surg [Br]2006;88-B:188–197.CrossrefPubMed Google Scholar
13 Roche MD, Augustin D, Conditt MA. One year outcomes of robotically guided UKA. J Bone Joint Surg [Br] 2010;92-B(Supp I):156-157. Google Scholar
14 Tarwala R , DorrLD. Robotic assisted total hip arthroplasty using the MAKO platform. Curr Rev Musculoskelet Med2011;4:151–156.CrossrefPubMed Google Scholar
15 Cobb JP , KannanV, BrustK, ThevendranG. Navigation reduces the learning curve in resurfacing total hip arthroplasty. Clin Orthop Relat Res2007;463:90–97.CrossrefPubMed Google Scholar
16 Hobsbawm E. Labouring Men. Studies in the History of Labour. London: Weidenfeld and Nicolson; 1964. Google Scholar
17 Cobb JP, Shimmin A. The Great Debate, 2011. http://www.imperial.ac.uk/surgeryandcancer/divisionofsurgery/clinical_themes/musculo/tgd2012/ (date last accessed 28 March 2012). Google Scholar