Abstract
Aims
The use of 3D printing has become increasingly popular and has been widely used in orthopaedic surgery. There has been a trend towards an increasing number of publications in this field, but existing literature incorporates limited high-quality studies, and there is a lack of reports on outcomes. The aim of this study was to perform a scoping review with Level I evidence on the application and effectiveness of 3D printing.
Methods
A literature search was performed in PubMed, Embase, and Web of Science databases. The keywords used for the search criteria were ((3d print*) OR (rapid prototyp*) OR (additive manufactur*)) AND (orthopaedic). The inclusion criteria were: 1) use of 3D printing in orthopaedics, 2) randomized controlled trials, and 3) studies with participants/patients. Risk of bias was assessed with Cochrane Collaboration Tool and PEDro Score. Pooled analysis was performed.
Results
Overall, 21 studies were included in our study with a pooled total of 932 participants. Pooled analysis showed that operating time (p < 0.001), blood loss (p < 0.001), fluoroscopy times (p < 0.001), bone union time (p < 0.001), pain (p = 0.040), accuracy (p < 0.001), and functional scores (p < 0.001) were significantly improved with 3D printing compared to the control group. There were no significant differences in complications.
Conclusion
3D printing is a rapidly developing field in orthopaedics. Our findings show that 3D printing is advantageous in terms of operating time, blood loss, fluoroscopy times, bone union time, pain, accuracy, and function. The use of 3D printing did not increase the risk of complications.
Cite this article: Bone Joint Res 2021;10(12):807–819.
Article focus
-
The use of 3D printing has become increasingly popular in orthopaedic surgery, but existing literature incorporates limited high-quality studies with case reports/series, and there is a lack of analysis on outcomes with level I evidence.
-
This scoping review evaluated the effectiveness of 3D printing, including functional outcomes and accuracy. Subgroup analyses were performed on preoperative and intraoperative use, as well as region of surgery.
Key messages
-
3D printing is advantageous in terms of operating time, blood loss, fluoroscopy times, bone union time, pain, accuracy, and function. There is no increase in complications.
-
The use of 3D printing is still at its early stages and development in orthopaedics. Further randomized controlled trials (RCTs) will delineate its full potential.
-
Surgeons should be cautious when using 3D printing for simulation and personalized cutting jigs, as CT image reconstruction may not fully reflect the thickness of the periosteum and soft-tissue on bone, which may result in improper placement of the cutting jig. This could have a potential effect on accuracy of various surgeries including fracture fixation, deformity corrections, tumour excision, arthroplasties, etc. Furthermore, when using personalized jigs, there may be more soft-tissue dissection to ensure accurate placements.
Strengths and limitations
-
This study incorporated only RCTs, which give Level I evidence.
-
This is the first analysis to assess the follow-up functional outcomes, accuracy, and complications with 3D printing compared with conventional group.
-
The included studies had some level of heterogeneity and different surgeries were performed in the selected RCTs. Also, there were few studies for pooled analysis for bone union time and pain score.
Introduction
In the modern era, the use of 3D printing has become increasingly popular and has been widely used for planning, simulating surgeries, and intraoperative procedures. The technology has allowed a wide range of possibilities, and has become an integral part of clinical workflow in producing patient-specific models that enhance visuospatial dexterity for the clinician and maximize perception of anatomy.1,2 Current medical usage of 3D printing has been applied in orthopaedic surgery,3 urology,4 cardiology,5 gastroenterology,6 and numerous other medical specialties. Education for the surgeon, trainee, and patient has also become incorporated into clinical practice with 2D images projected into a 3D-customized model.
The potential and diversity of 3D printing has been growing since its development. 3D models are created by fusing or depositing materials including plastics, ceramics, powders, liquids, or even living cells. This process is also known as additive manufacturing, rapid prototyping, or solid free-form technology.7 Nowadays, with wider availability, prices have become much more affordable compared to the past. In fact, new models can now be purchased at approximately USD $300.3 Commercially available 3D printers also have readily available software that converts CT and MRI data into 3D models that can be printed with convenience. This has allowed more widespread use as anatomical abnormalities can be better appreciated by the surgeon for operations.
In orthopaedic surgery, the use of 3D printing has allowed advances in medical treatment. Applications include preoperative planning, education, surgical cutting guides, rehabilitation devices, surgical simulation, and prosthesis development.8 Currently, there has been a trend towards an increasing number of publications in this field, but current review papers have shown that existing literature has limited high-quality studies, with most being case reports and case series.8,9 Previous analysis also had limited Level I evidence, showing only the early results of 3D printing,10 and whether follow-up outcomes and accuracy are improved or complication rates are increased is still largely unknown.9 In recent years, there has been an increased emergence of randomized controlled trials (RCTs), and therefore analysis is warranted. The aim of this study was to perform a scoping review and provide evidence with high-quality RCTs to highlight the effectiveness of 3D printing in orthopaedic surgery.
Methods
Search strategy
The PubMed, Embase, and Web of Science databases (date last accessed 26 April 2021) were searched. The keywords used for the search criteria were ((3d print*) OR (rapid prototyp*) OR (additive manufactur*)) AND (orthopaedic).
Search criteria
The inclusion criteria were: 1) use of 3D printing in orthopaedics, 2) RCTs, and 3) studies with participants/patients. The exclusion criteria were: 1) non-RCTs, 2) basic science studies, 3) conference abstracts, 4) articles not in English, 5) protocol studies, 6) no control group, 7) non-orthopaedic-related/no orthopaedic pathology, 8) missing data, and 9) nonoperative study.
Selection of studies
Two independent reviewers (RMYW, SWL) selected the articles. Each reviewer screened the titles and abstracts of each published study. Articles were selected based on the inclusion and exclusion criteria. Each article was reviewed and any disagreement was resolved by consensus and discussion.
Data extraction
For eligible studies, the two reviewers extracted information on: 1) country of study, 2) orthopaedic condition, 3) interventional groups and 3D printing details, 4) sample size, 5) age, 6) clinical outcomes, 7) follow-up outcomes, 8) complications, and 9) key findings of study.
Quality assessment of studies
The Cochrane Collaboration tool was used to assess the risk of bias in the domains of random sequence generation, allocation concealment, blinding of participants and personnel, blinding of outcome assessments, incomplete outcome data, and selective reporting.11 Risk of bias in each domain was classified as low-risk, high-risk, or unclear. Quality of the studies was further assessed with the PEDro scale.12 The scale consists of 11 items to assess the quality of internal validity and statistical information. Studies scoring ≥ 6 are considered “good” quality, 4 to 5 are “fair” quality, and < 4 are of “poor” quality. Poor-quality trials would be excluded from analysis.
Statistical analysis
Assessment outcomes of operating time (minutes), blood loss (millilitres), fluoroscopy (number of times), bone union (months), pain (visual analogue scale), accuracy (fracture reduction, stem placement, and pedicle screw placement), function (functional score), and complications (event) were performed. Preoperative and intraoperative subgroup analysis was performed where data were available to assess effectiveness of 3D printing. Upper limb, spine, and lower limb subgroup analysis was performed where data were available. The effects of 3D printing on outcomes were analyzed by Review Manager (RevMan 5.4, The Cochrane Centre, The Cochrane Collaboration). For results with continuous variables, the standardized mean difference (SMD) and 95% confidence interval (CI) of the outcomes were calculated. All models used z-test to determine whether there is statistically significant difference between two compared groups. For results with dichotomous variables, the odds ratios (ORs) with 95% CI of the outcomes were calculated. A p-value < 0.05 was considered statistically significant. Trial sequential analysis was performed to assess the statistical reliability of functional score. Heterogeneity was evaluated by I2 index or p-value test, where the I2 index > 50% or p-value < 0.1 represented significant heterogeneity between studies. The random effect models were used in the forest plots of operating time, blood loss, use of fluoroscopy times, and functional score, where there was heterogeneity. While fixed-effect models were used in the forest plots of bone union time, pain score, accuracy, and complications, where results were not heterogenous and also categorical (accuracy and complications).
Results
Literature search
A total of 2,318, 1,106, and 311 studies were identified from PubMed, Embase, and Web of Science, respectively. All duplicate entries were removed, leaving 2,987 studies. Each title and abstract were then reviewed, and 2,956 studies were removed based on inclusion and exclusion criteria. Overall, 31 full-text articles were reviewed and a further ten were excluded: two had no pathology, one had no control group, two were protocol papers, one had missing data, and four were nonoperative studies. Our results show a total of 21 studies for our scoping review (Figure 1).
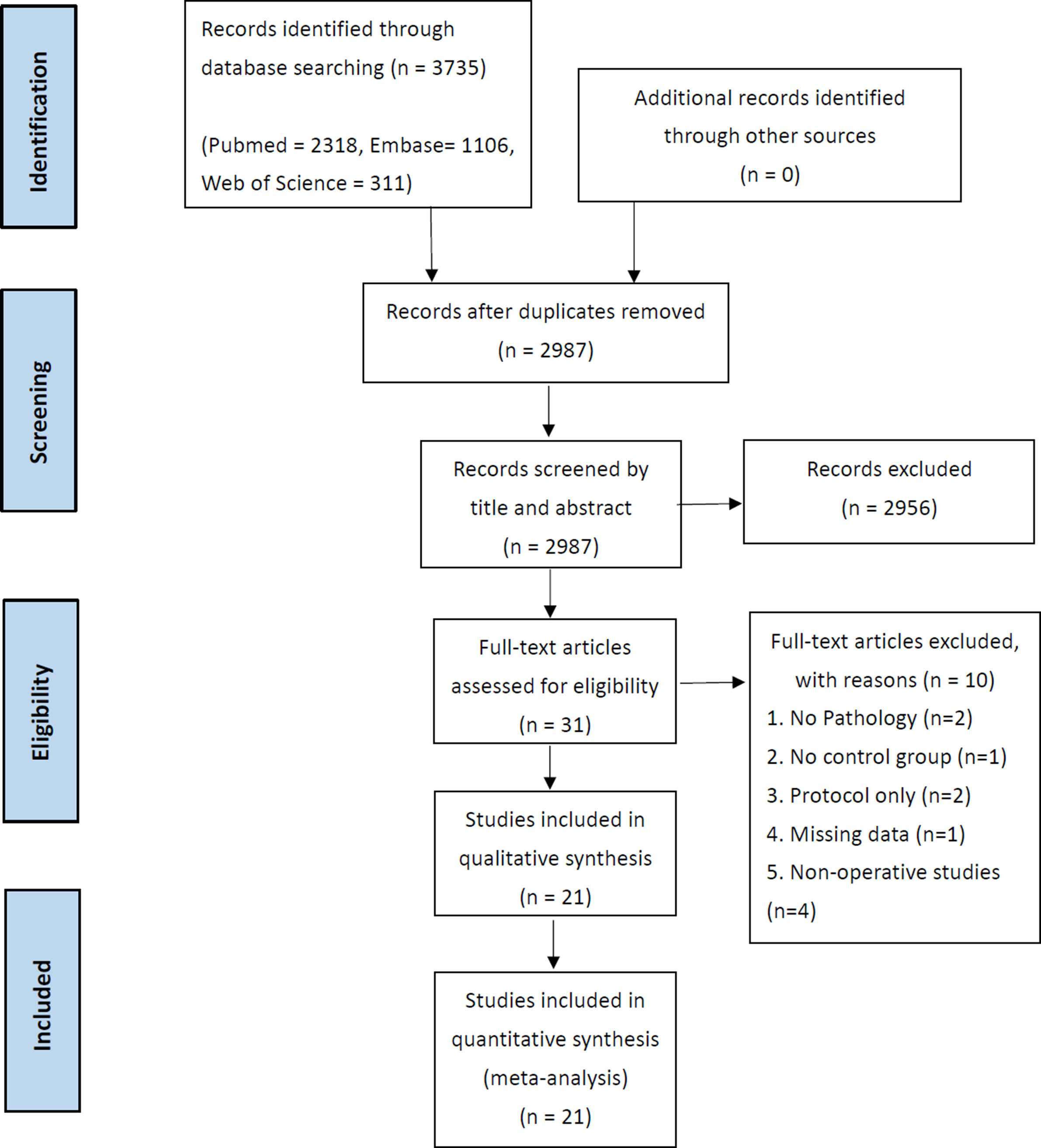
Fig. 1
Preferred Reporting Items for Systematic Reviews and Meta-Analyses (PRISMA) flow diagram.
Characteristics of studies
The 21 studies were published from 2013 to 2020, and were conducted in four countries including China, Sweden, India, and Slovenia. All were RCTs with the presence of a control group. There was a pooled total of 932 participants; the sample size ranged from 12 to 93. All papers were surgery-related, with 12 articles related to orthopaedic fractures, three related to the elective spine, one related to upper limb reconstruction, four related to adult joint reconstruction, and one related to foot and ankle speciality. 3D printing was used in terms of surgical planning, instrumentation, and surgical guide template. Out of the 21 studies, 17 had follow-up outcomes. The follow-up period ranged from seven days to two years (Table I).
Table I.
PEDro score of included studies.
Study | Eligibility criteria | Randomized allocation | Concealed allocation | Baseline comparability | Blinded participants | Blinded therapists | Blinded assessors | Adequate follow-up | Intention-to-treat analysis | Between-group comparisons | Point estimates and variability | Total score |
---|---|---|---|---|---|---|---|---|---|---|---|---|
Chen et al13 | 1 | 1 | 0 | 0 | 1 | 0 | 0 | 0 | 0 | 1 | 1 | 5 |
Zheng et al14 | 1 | 1 | 1 | 1 | 0 | 0 | 0 | 1 | 0 | 1 | 1 | 7 |
Huang et al15 | 1 | 1 | 0 | 1 | 0 | 0 | 0 | 1 | 1 | 1 | 1 | 7 |
You et al16 | 0 | 1 | 0 | 1 | 0 | 0 | 0 | 1 | 1 | 1 | 1 | 6 |
Kong et al17 | 1 | 1 | 1 | 1 | 1 | 0 | 0 | 1 | 1 | 1 | 1 | 9 |
Shuang et al18 | 0 | 1 | 0 | 1 | 0 | 0 | 0 | 1 | 1 | 1 | 1 | 6 |
Zhang et al19 | 0 | 1 | 0 | 1 | 0 | 0 | 0 | 1 | 1 | 1 | 1 | 6 |
Maini et al20 | 1 | 1 | 0 | 0 | 0 | 0 | 0 | 1 | 1 | 1 | 0 | 5 |
Zheng et al21 | 1 | 1 | 0 | 1 | 0 | 0 | 0 | 1 | 1 | 1 | 1 | 7 |
Yang et al22 | 0 | 1 | 0 | 0 | 0 | 0 | 0 | 1 | 1 | 1 | 1 | 5 |
Zheng et al23 | 1 | 1 | 0 | 1 | 0 | 0 | 0 | 1 | 1 | 1 | 1 | 7 |
Hu et al24 | 1 | 1 | 0 | 0 | 0 | 0 | 0 | 1 | 1 | 1 | 1 | 6 |
Feng et al25 | 1 | 1 | 1 | 1 | 0 | 0 | 1 | 1 | 1 | 1 | 1 | 9 |
Merc et al26 | 0 | 0 | 0 | 0 | 0 | 0 | 0 | 1 | 1 | 1 | 1 | 4 |
Wu et al27 | 1 | 1 | 0 | 1 | 0 | 0 | 0 | 1 | 1 | 1 | 1 | 7 |
Hasan et al28 | 1 | 1 | 1 | 1 | 1 | 0 | 0 | 1 | 1 | 1 | 1 | 9 |
Zhang et al29 | 1 | 1 | 0 | 0 | 1 | 0 | 0 | 1 | 1 | 1 | 1 | 7 |
Sun et al30 | 1 | 1 | 0 | 1 | 1 | 0 | 0 | 1 | 1 | 1 | 1 | 8 |
Du et al31 | 0 | 0 | 0 | 0 | 0 | 0 | 0 | 1 | 1 | 1 | 1 | 4 |
Wei et al32 | 1 | 1 | 0 | 1 | 0 | 1 | 0 | 1 | 1 | 1 | 1 | 8 |
Yin et al33 | 0 | 1 | 0 | 1 | 0 | 0 | 0 | 1 | 1 | 1 | 1 | 6 |
Main measurement outcomes
In comparing 3D printing and conventional practice for the 21 papers, 19 studies assessed operating time, 13 assessed blood loss, eight assessed fluoroscopy time, five assessed bone union time, five assessed pain score, seven assessed accuracy, 13 assessed functional outcomes with scores, and 13 assessed complications (Supplementary Table i).
Risk of bias
The risk of bias of all studies was assessed and shown in Figure 2. A total of 11 studies performed random sequence generation with low risk of bias, eight were unclear, and two were at high risk of bias. For allocation concealment, four studies were considered at low risk of bias, 14 were unclear, and three were at high risk of bias. For the method of blinding, five studies had low risk of bias, five were unclear, and 11 were at high risk of bias. For the blinding of outcome assessors, four studies were at low risk of bias, 11 were unclear, and six were at high risk of bias. For incomplete data, 19 studies had low risk of bias, one was unclear, and one had high risk of bias. For selective reporting, 14 had low risk of bias and seven had high risk of bias. For other bias, 16 had low risk of bias, one was unclear, and four had high risk of bias. PEDro was also performed and is summarized in Table I. All studies had a score of 4 or more.
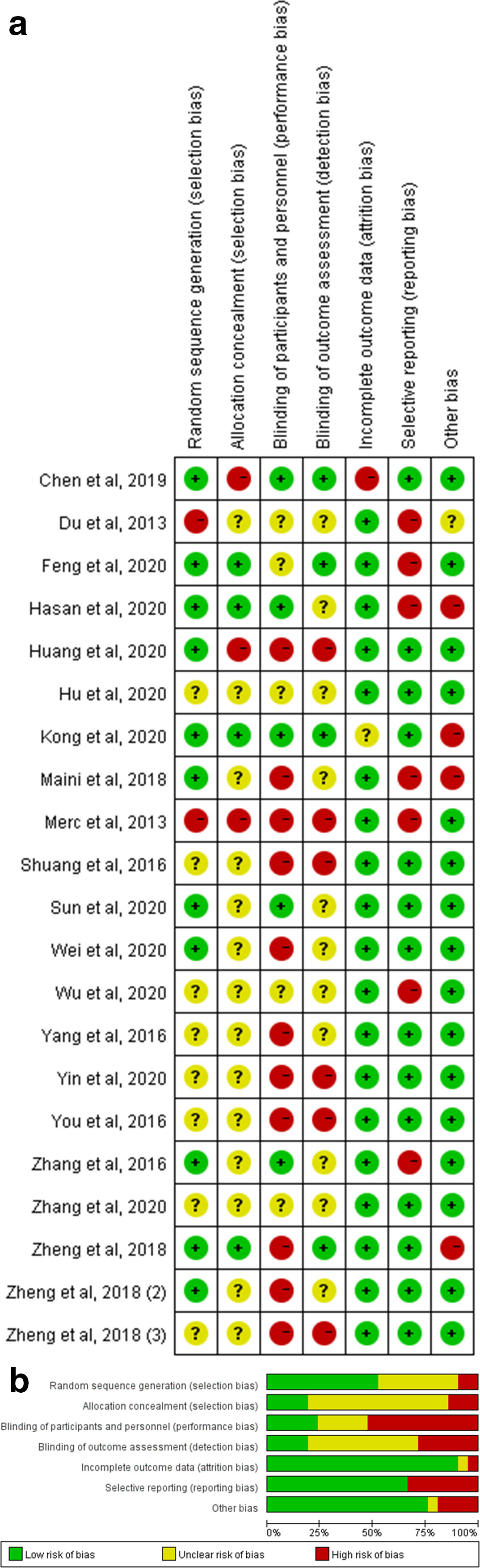
Fig. 2
a) Summary of risk of bias assessment of included randomized controlled trials (RCTs). b) Graph of the risk of bias assessment of included RCTs.
Pooled quantitative analysis
Overall, 21 RCTs proceeded for the pooled analysis to compare 3D printing with control group.
Operating time
A total of 19 studies reported operating times (minutes). Results showed that operating time was significantly less in the 3D printing group compared to the control group (standardized mean difference (SMD) = -1.28, 95% CI -1.92 to -0.65, p < 0.001, z-test). Subgroup analysis of preoperative use of 3D printing for surgical planning also decreased operating times compared to control group (SMD = -1.63, 95% CI -1.89 to -1.38, p < 0.001, z-test). Regarding intraoperative use of 3D printing, it also decreased operating times significantly compared to control group (SMD = -1.09, 95% CI -2.08 to -0.09, p = 0.030, z-test) (Figure 3).
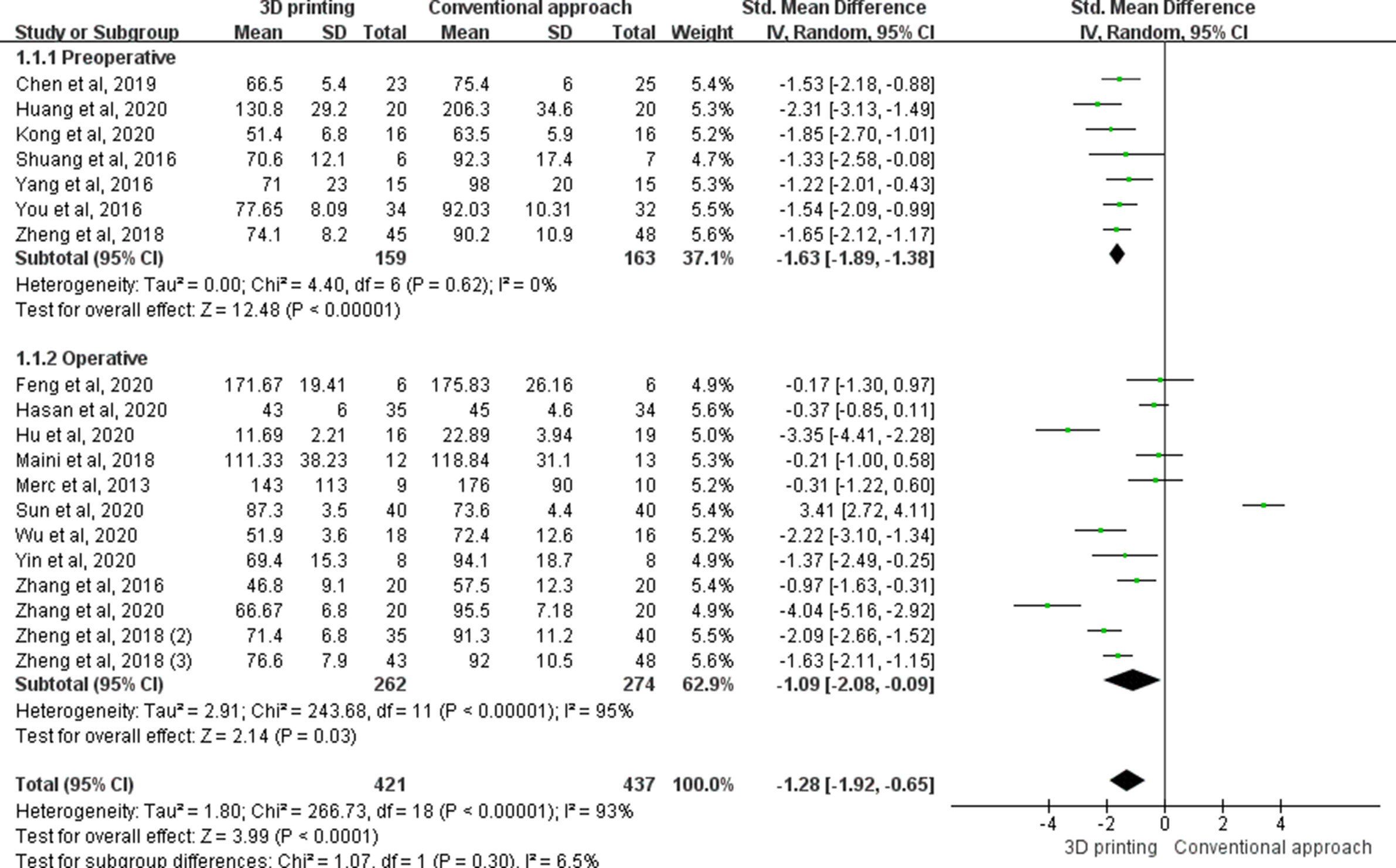
Fig. 3
The forest plot of operating time (mins). Preoperative: the 3D printing model is only used for preoperative elements, such as surgical planning, tools determination, or surgery simulation. Operative: the 3D printing model is used for factors related to operating time, such as guidance or instrumentation. CI, confidence interval; IV, inverse variance; SD, standard deviation.
Subgroup analysis for operating time for upper limb surgery with 3D printing was significantly decreased compared to the control group (SMD -1.74, 95% CI -2.13 to -1.35, p < 0.001, z-test). However, no significant differences were found for spine surgery and lower limb surgery.
Blood loss
A total of 13 studies reported on blood loss (millilitres) in operations. Results showed that blood loss was significantly less in the 3D printing group compared to control group (SMD -1.81, 95% CI -2.47 to -1.15, p < 0.001, z-test). Subgroup analysis of preoperative use of 3D printing for blood loss in operations was also decreased compared to the control group (SMD -1.4, 95% CI -1.85 to -0.94, p < 0.001, z-test). Intraoperative use of 3D printing for blood loss in operations was also significantly decreased compared to the control group (SMD -2.28, 95% CI -3.56 to -1.00, p < 0.001, z-test) (Figure 4).

Fig. 4
The forest plot of intraoperative blood loss (ml). Preoperative: the 3D printing model is only used for preoperative elements, such as surgical planning, tools determination, or surgery simulation. Operative: the 3D printing model is used in factors related to operating time, such as guidance or instrumentation. CI, confidence interval; IV, inverse variance; SD, standard deviation.
Subgroup analysis for blood loss for upper limb surgery with 3D printing was significantly decreased compared to the control group (SMD -1.67, 95% CI -2.45 to -0.90, p < 0.001, z-test). This was also significant for 3D printing in lower limb surgery compared to the control group (SMD -1.52, 95% CI -2.41 to -0.63, p < 0.001, z-test). However, no significant difference was found for spine surgery.
Use of fluoroscopy times
Eight studies reported on fluoroscopy times in operations. Results showed that fluoroscopy was significantly less in the 3D printing group compared to the control group (SMD -1.60, 95% CI -2.06 to -1.14, p < 0.001, z-test). Subgroup analysis of preoperative use of 3D printing for fluoroscopy times in operations was also decreased compared to the control group (SMD -1.41, 95% CI -1.91 to -0.91, p < 0.001, z-test). Intraoperative use of 3D printing for fluoroscopy times in operations was also significantly decreased compared to control group (SMD -2.08, 95% CI -3.18 to -0.97, p < 0.001, z-test) (Figure 5).
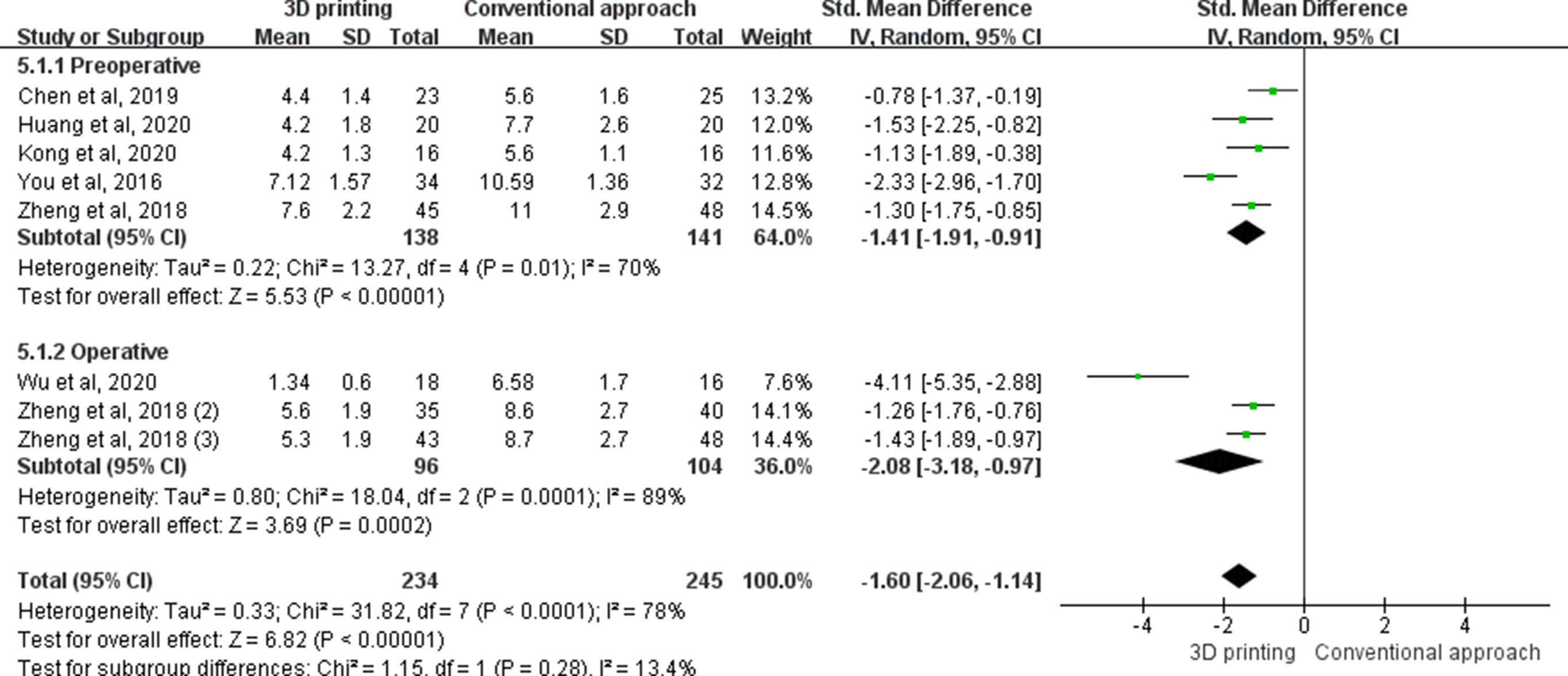
Fig. 5
The forest plot of intraoperative fluoroscopy (number of times). Preoperative: the 3D printing model is only used for preoperative elements, such as surgical planning, tools determination, or surgery simulation. Operative: the 3D printing model is used in factors related to operating time, such as guidance or instrumentation. CI, confidence interval; IV, inverse variance; SD, standard deviation.
Subgroup analysis for fluoroscopy times for upper limb surgery with 3D printing was significantly decreased compared to the control group (SMD -1.42, 95% CI -2.04 to -0.80, p < 0.001, z-test). This was also significant for 3D printing in lower limb surgery compared to control group (SMD -1.85, 95% CI -2.66 to -1.05, p < 0.001, z-test).
Bone union time
Five studies reported on bone union time in operations. Results showed that this was significantly less in the 3D printing group compared to the control group (SMD -0.36, 95% CI -0.57 to -0.15, p < 0.001, z-test) (Figure 6).
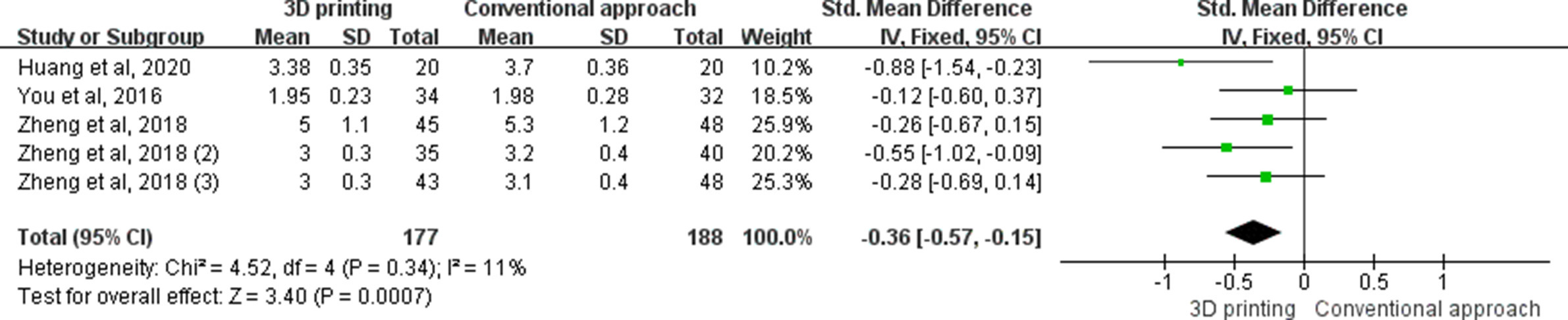
Fig. 6
The forest plot of bone union time (mths). CI, confidence interval; IV, inverse variance; SD, standard deviation.
Subgroup analysis for bone union time for lower limb surgery with 3D printing was significantly decreased compared to the control group (SMD -0.48, 95% CI -0.75 to -0.20, p < 0.001, z-test). There was no significant difference for 3D printing in upper limb surgery compared to the control group.
Pain score
Five studies reported on pain. Results showed that pain was significantly less in the 3D printing group compared to control group (SMD -0.26, 95% CI -0.51 to -0.01, p = 0.040, z-test) (Figure 7).
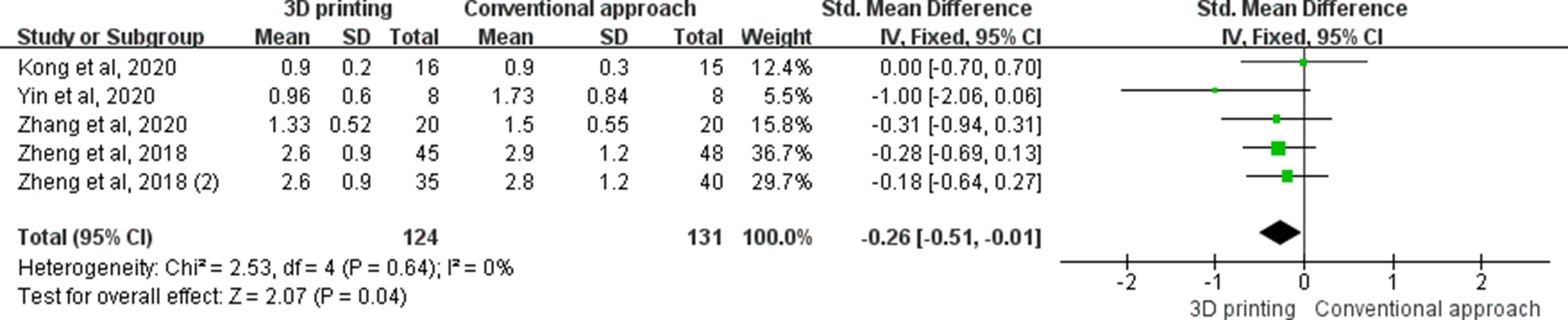
Fig. 7
The forest plot of visual analogue scale (VAS). CI, confidence interval; IV, inverse variance; SD, standard deviation.
Subgroup analysis for pain score showed that there was no significant difference for 3D printing in all three subgroups (upper limb, lower limb surgery, and spine surgery) compared to the control group.
Accuracy
Seven studies were analyzed for accuracy. Results showed that accuracy was significantly improved with 3D printing compared to the control group (SMD 0.24, 95% CI 0.14 to 0.40, p < 0.001, z-test). Subgroup analysis of lower limb/pelvis surgery with 3D printing was significantly improved with 3D printing compared to the control group (SMD 0.22, 95% CI 0.10 to 0.45, p < 0.001, z-test). Regarding spine pedicle screw placement, the use of 3D printing was also significantly more accurate compared to the control group (SMD 0.26, 95% CI 0.13 to 0.54, p < 0.001, z-test) (Figure 8).

Fig. 8
The forest plot of subgroup analysis for accuracy. CI, confidence interval; IV, inverse variance; M-H, Mantel-Haenszel; SD, standard deviation.
Functional score
A total of 13 studies report on functional scores in different locations including the elbow, ankle, knee, wrist, and spine. Results showed that there was an overall significant difference between the 3D printing group and the control group at follow-up (SMD 0.29, 95% CI 0.13 to 0.45, p < 0.001, z-test). Subgroup analysis showed significant differences in the knee (SMD 0.32, 95% CI 0.03 to 0.61, p = 0.030, z-test) and spine (SMD 0.72, 95% CI 0.15 to 1.28, p = 0.010, z-test) (Figure 9).
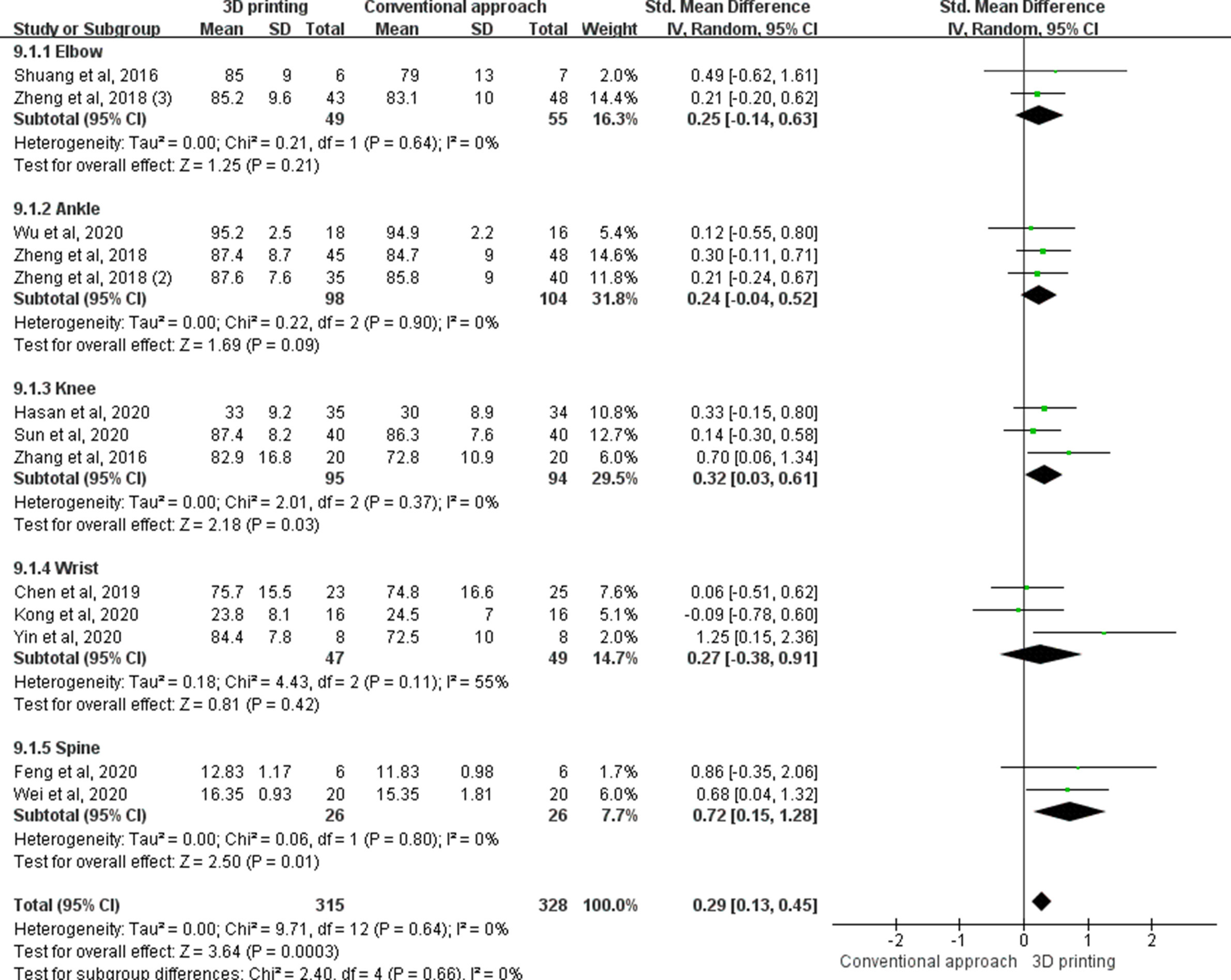
Fig. 9
The forest plot of functional score in different locations including the elbow, ankle, knee, wrist, and spine. CI, confidence interval; IV, inverse variance.
Subgroup analysis for functional score for spine surgery with 3D printing was significantly improved compared to the control group (SMD 0.72, 95% CI 0.15 to 1.28, p = 0.010, z-test). This was also significant for 3D printing in lower limb surgery compared to the control group (SMD 0.28, 95% CI 0.08 to 0.48, p = 0.006, z-test). There was no significant difference for 3D printing in upper limb surgery compared to the control group. The trial sequential analysis (TSA) showed that the result was significant and favoured 3D printing. The cumulative Z-curve had crossed the conventional boundary and monitoring boundary for benefit. The number of patients was 643, which was higher than the required information size.
Complications
A total of 13 studies reported on complications. Results showed that these were not significantly different between the 3D printing group and the control group (SMD 0.66, 95% CI 0.37 to 1.17, p = 0.160). Subgroup analysis of upper limb, spine, or lower limb/pelvis surgery did not show significant differences either (Figure 10).
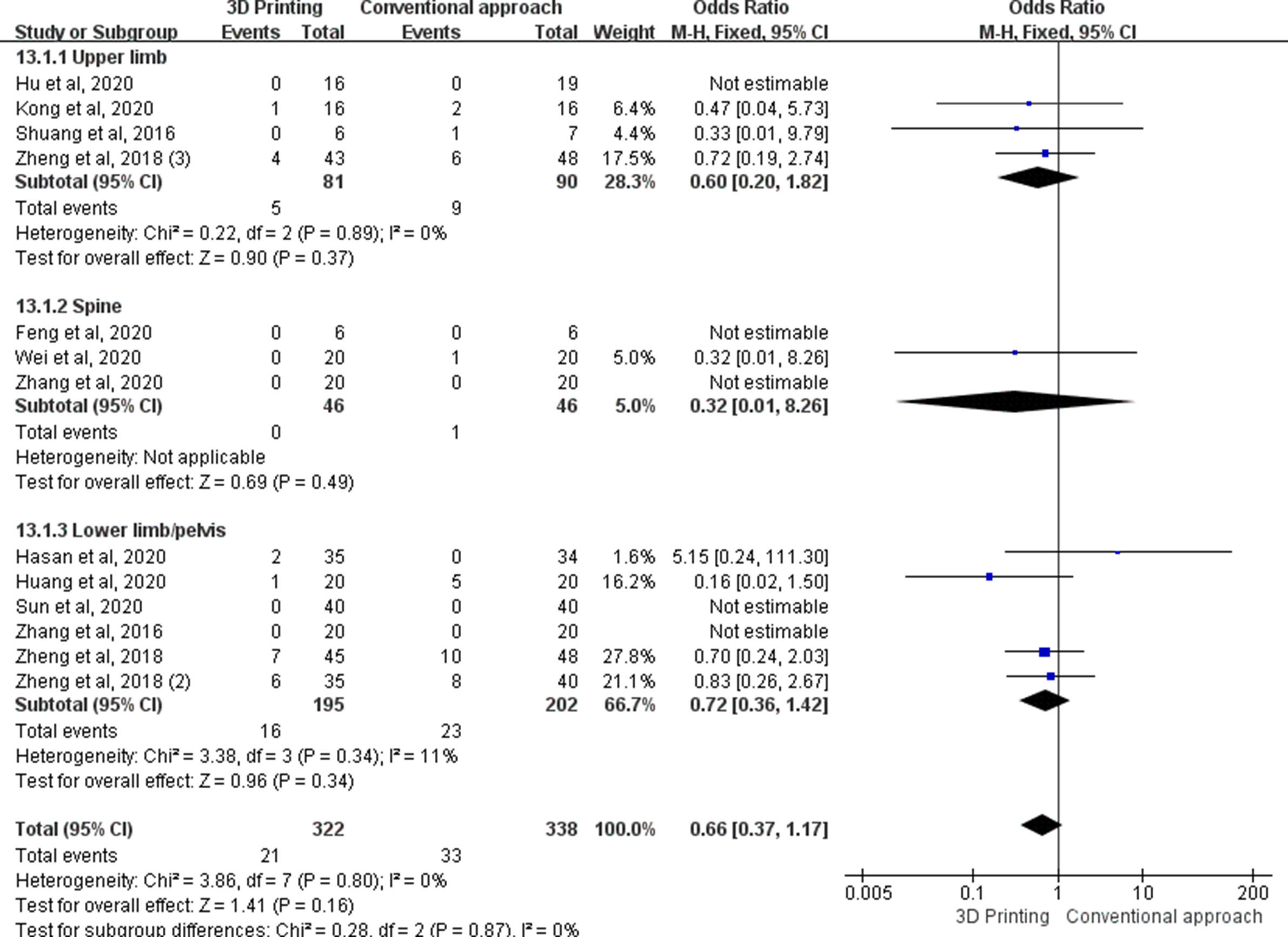
Fig. 10
The forest plot of subgroup analysis for complications. CI, confidence interval; IV, inverse variance; M-H, Mantel-Haenszel; SD, standard deviation.
Discussion
There has been an increase in 3D printing applications in orthopaedics in the past decade. Although numerous studies have been published, a substantial amount are case reports and case series. In fact, many systematic reviews have also incorporated these studies.9,34 A high level of evidence is necessary to determine the application and actual effect of 3D printing. Furthermore, there is currently a lack of pooled evidence on follow-up function, pain, and bone union,9,34,35 as well as subgroup analysis on the preoperative and intraoperative usage. With the recent increase in the number of RCTs, the aim of this study was to perform a scoping review with level I evidence in orthopaedics. A scoping review aids in providing current knowledge in the field by mapping the body of the literature on a topic.35
3D printing in templating has been performed in numerous studies and applications. It has been demonstrated that 3D printing for developmental dysplastic hips shows that the positioning was comparable with the conventional group. However, operating time and blood loss were significantly less for the 3D printing group.36 Its usefulness in optimizing inventory stock has also been shown, with 93% and 89% correctness in predicting the sizes for the acetabular and femoral components, respectively. This has led to a 61% reduction of implant inventory size.37 More importantly, in complex revision hip arthroplasties, the use of 3D printing allows for better evaluation of anatomy with accurate templating. Acetabular component, augment, buttress, and cage size can be trialled before surgery. This improves preoperative planning and would prove to be a useful clinical tool.38
The operating time with 3D printing was significantly shorter compared to the control group. In fact, this was present for both preoperative and intraoperative use. Further subgroup analysis showed that the operating time for upper limb surgeries benefited with 3D printing. With better preoperative surgical planning, it is expected that operating times will shorten. Contouring of plates onto fractures can take considerable time and can prolong surgery; therefore, 3D models for surgical planning prepared prior to surgery can save significant time.39 It is also useful in complex cases to have a rehearsal of the operation, surgical simulation, and intraoperative referencing.40 More importantly, a study from 2020 has shown that shorter surgeries can reduce operating theatre costs as procedure time is shortened,41 and there are decreased staff and operating theatre overheads. Other than orthopaedics, 3D printing has also been found to save costs in other specialities, including craniofacial reconstruction surgery.42 Particularly with the ageing population and increase in osteoporotic fractures, there is also an expected increase in the number of more complex surgeries.43,44
Blood loss and fluoroscopy times were significantly less compared to control groups in our meta-analysis. Both preoperative and intraoperative use of 3D printing was also significant (all p-values < 0.001, z-test). With shorter operating time and pre-planned surgery, the blood loss is understandably shorter, and postoperative functional recovery is also enhanced.45 The use of custom models is also patient-specific and provides an accurate approach for each patient. Furthermore, templating of implants prior to surgery would also significantly reduce fluoroscopy times as trials are reduced. Further analysis revealed that upper limb and lower limb surgeries had reduced blood loss. Although a trend emerged for spine surgery, it was not statistically significant (p = 0.270, z-test). This may be related to the more extensive dissection for spine surgeries, as well as the limited number of studies available for analysis. Future studies using 3D printing for spine surgery would reveal more evidence for the efficacy of reducing blood loss.
Radiation risks can lead to potentially serious consequences, especially to the healthcare personnel and the patient. In fact, studies have shown that orthopaedic surgeons have a significantly increased risk of cancer.46,47 During an operation, sensitive organs include the thyroid, eyes, hands, and gonads.48 Based on this, the International Commission on Radiological Protection has also set dosage limits for radiation to protect personnel.49 The use of 3D printing models would provide a benefit by reducing occupational hazards. It was shown with subgroup analysis that both upper limb and lower limb surgeries benefited with decreased fluoroscopy time compared to respective control groups.
It has been largely controversial as to whether 3D printing technologies aid in enhanced fracture union. Our results show that the pooled effect is significantly better. With quicker surgery, decreased blood loss, and increased accuracy, the biology of the fracture is preserved. This finding is important, especially in orthopaedic trauma where enhanced fracture healing can result in faster rehabilitation and return to function of the patient. Subgroup analysis also showed that lower limb and pelvis surgery resulted in quicker union. This reinforces the fact that mobilization can be enhanced. As for the pain score, although the subgroup analysis did not show significant difference in all three subgroups, it is likely due to the limited number of studies for analysis, as pooled analysis showed that 3D printing could significantly relieve pain compared with the control group.
Our study also analyzes the follow-up function of patients, and the pooled effect also correlated with improved function of these patients. Subgroup analysis also showed a significant effect on the lower limb and spine. As faster union rates, shorter surgeries, and blood loss are related to rehabilitation, it is also expected that function would improve. Besides bone fractures, diseases of articular cartilage are also prevalent. There has been an increase in biomaterials used to promote cartilage regeneration.50 3D bioprinting technology has also assisted the shaping of meniscus through stem cell-based materials,51 which has the potential to be clinically translated into bioactive implants in the future.
Our results showed that accuracy significantly improved with 3D printing compared to control group. This is clinically important as often anatomical reduction of fractures is required to prevent early degeneration and pain. Furthermore, the implications of accurate placement of pedicle screws are also important, as misplacement can lead to serious neurological injuries. More importantly, the use of 3D printing did not result in increased complications, which shows the safety of its use in various orthopaedic surgeries.
The use of 3D printing has also spread to medical teaching. Recent 3D-printed models allow for improved identification and understanding of complex anatomy, which is often difficult to appreciate with traditional textbooks. Previous studies have shown that 3D printing is suitable for teaching anatomy with the high resolution and colour reproductions now available.52,53 It also has advantages over traditional cadaver specimens with the health and safety issues of formalin fluids, costs, and access.54 Furthermore, current 3D-printed models often differ from cadavers by less than 2 mm, displaying accurate depictions of anatomy, with positive feedback from medical students.55 These 3D models can also be used to teach and inform patients regarding their condition and the surgical procedure that they will be undergoing.56 Alongside the use of 3D printing in teaching orthopaedics, the technology has also been adopted in other specialities including cardiac and vascular surgery,57 hepatic surgery,58 and ophthalmology.59
The strength of this study is that it incorporates only RCTs, which gives level I evidence. To the authors’ knowledge, this study also has the largest number of RCTs to date and gives the latest updated evidence of 3D printing in the field of orthopaedics. It is also the first meta-analysis to assess the follow-up function, accuracy, and complications of studies, which provides valuable data in the effectiveness of 3D printing in the long term. Furthermore, we have analyzed the preoperative and intraoperative effectiveness, as well as regions of surgery in various parameters. However, surgeons should be cautious when using 3D printing for simulation and personalized cutting jigs, as CT image reconstruction may not fully reflect the thickness of the periosteum and soft-tissue on bone, which may result in improper placement of the cutting jig. This could potentially affect the accuracy of various surgeries including fracture fixation, deformity corrections, tumour excision, and arthroplasties. Furthermore, when using personalized jigs, there may be more soft-tissue dissection to ensure accurate placements.
The limitation of this study is the level of heterogeneity of studies, and the fact that different surgeries were performed in the selected RCTs. Furthermore, for the pooled data for bone union time and pain, there were only five studies that were available for each analysis. More convincing evidence would be available if future studies concentrate in these parameters.
In conclusion, 3D printing is a rapidly developing field in orthopaedics. This study provides level I quality evidence of its current applications and effectiveness. Our findings show that 3D printing is advantageous in terms of operating time, blood loss, fluoroscopy times, bone union time, accuracy, function, and pain. The use of 3D printing is still in its early stages and development in medical teaching and orthotics. Further RCTs will delineate its full potential in these areas.
References
1. Giannopoulos AA , Mitsouras D , Yoo SJ , Liu PP , Chatzizisis YS , Rybicki FJ . Applications of 3D printing in cardiovascular diseases . Nat Rev Cardiol . 2016 ; 13 ( 12 ): 701 – 718 . Crossref PubMed Google Scholar
2. Wang M . 3D printing aids transplantation planning . Nat Rev Nephrol . 2018 ; 14 ( 12 ): 721 . Crossref PubMed Google Scholar
3. Colaco M , Igel DA , Atala A . The potential of 3D printing in urological research and patient care . Nat Rev Urol . 2018 ; 15 ( 4 ): 213 – 221 . Crossref PubMed Google Scholar
4. Chen MY , Skewes J , Desselle M , et al. Current applications of three-dimensional printing in urology . BJU Int . 2020 ; 125 ( 1 ): 17 – 27 . Crossref PubMed Google Scholar
5. Vukicevic M , Mosadegh B , Min JK , Little SH . Cardiac 3D Printing and its Future Directions . JACC Cardiovasc Imaging . 2017 ; 10 ( 2 ): 171 – 184 . Crossref PubMed Google Scholar
6. Wengerter BC , Emre G , Park JY , Geibel J . Three-dimensional Printing in the Intestine . Clin Gastroenterol Hepatol . 2016 ; 14 ( 8 ): 1081 – 1085 . Crossref PubMed Google Scholar
7. Ventola CL . Medical Applications for 3D Printing: Current and Projected Uses . P T . 2014 ; 39 ( 10 ): 704 – 711 . PubMed Google Scholar
8. Jiang M , Chen G , Coles-Black J , Chuen J , Hardidge A . Three-dimensional printing in orthopaedic preoperative planning improves intraoperative metrics: a systematic review . ANZ J Surg . 2020 ; 90 ( 3 ): 243 – 250 . Crossref PubMed Google Scholar
9. Levesque JN , Shah A , Ekhtiari S , Yan JR , Thornley P , Williams DS . Three-dimensional printing in orthopaedic surgery: a scoping review . EFORT Open Rev . 2020 ; 5 ( 7 ): 430 – 441 . Crossref PubMed Google Scholar
10. Vaishya R , Patralekh MK , Vaish A , Agarwal AK , Vijay V . Publication trends and knowledge mapping in 3D printing in orthopaedics . J Clin Orthop Trauma . 2018 ; 9 ( 3 ): 194 – 201 . Crossref PubMed Google Scholar
11. Higgins JPT , Altman DG , Gøtzsche PC , et al. The Cochrane Collaboration’s tool for assessing risk of bias in randomised trials . BMJ . 2011 ; 343 : d5928 . Google Scholar
12. Maher CG , Sherrington C , Herbert RD , Moseley AM , Elkins M . Reliability of the PEDro scale for rating quality of randomized controlled trials . Phys Ther . 2003 ; 83 ( 8 ): 713 – 721 . PubMed Google Scholar
13. Chen C , Cai L , Zheng W , Wang J , Guo X , Chen H . The efficacy of using 3D printing models in the treatment of fractures: a randomised clinical trial . BMC Musculoskelet Disord . 2019 ; 20 ( 1 ): 65 . Crossref PubMed Google Scholar
14. Zheng W , Chen C , Zhang C , Tao Z , Cai L . The feasibility of 3D printing technology on the treatment of pilon fracture and Its effect on doctor-patient ommunication . Biomed Res Int . 2018 ; 2018 : 8054698 . Google Scholar
15. Huang J-. H , Liao H , Tan X-. Y , et al. Surgical treatment for both-column acetabular fractures using pre-operative virtual simulation and three-dimensional printing techniques . Chin Med J . 2020 ; 133 ( 4 ): 395 – 401 . Crossref PubMed Google Scholar
16. You W , Liu LJ , Chen HX , et al. Application of 3D printing technology on the treatment of complex proximal humeral fractures (Neer3-part and 4-part) in old people . Orthop Traumatol Surg Res . 2016 ; 102 ( 7 ): 897 – 903 . Crossref PubMed Google Scholar
17. Kong L , Yang G , Yu J , et al. Surgical treatment of intra-articular distal radius fractures with the assistance of three-dimensional printing technique . Medicine (Baltimore) . 2020 ; 99 ( 8 ): e19259 . Crossref PubMed Google Scholar
18. Shuang F , Hu W , Shao Y , Li H , Zou H . Treatment of intercondylar humeral fractures with 3D-printed osteosynthesis lates . Medicine (Baltimore) . 2016 ; 95 ( 3 ): e2461 . Google Scholar
19. Zhang M , Li J , Fang T , et al. Evaluation of a three-dimensional printed guide and a polyoxymethylene thermoplastic regulator for percutaneous pedicle screw fixation in patients with thoracolumbar fracture . Med Sci Monit . 2020 ; 26 :: e920578 . Crossref PubMed Google Scholar
20. Maini L , Verma T , Sharma A , Sharma A , Mishra A , Jha S . Evaluation of accuracy of virtual surgical planning for patient-specific pre-contoured plate in acetabular fracture fixation . Arch Orthop Trauma Surg . 2018 ; 138 ( 4 ): 495 – 504 . Crossref PubMed Google Scholar
21. Zheng W , Tao Z , Lou Y , et al. Comparison of the conventional surgery and the surgery assisted by 3d printing technology in the treatment of calcaneal fractures . J Invest Surg . 2018 ; 31 ( 6 ): 557 – 567 . Crossref PubMed Google Scholar
22. Yang L , Shang X-W , Fan J-N , et al. Application of 3D printing in the surgical planning of trimalleolar fracture and doctor-patient communication . Biomed Res Int . 2016 ; 2016 : 2482086 . Crossref PubMed Google Scholar
23. Zheng W , Su J , Cai L , et al. Application of 3D-printing technology in the treatment of humeral intercondylar fractures . Orthop Traumatol Surg Res . 2018 ; 104 ( 1 ): 83 – 88 . Crossref PubMed Google Scholar
24. Hu X , Zhong M , Lou Y , et al. Clinical application of individualized 3D-printed navigation template to children with cubitus varus deformity . J Orthop Surg Res . 2020 ; 15 ( 1 ): 111 . Crossref PubMed Google Scholar
25. Feng S , Lin J , Su N , Meng H , Yang Y , Fei Q . 3-Dimensional printing templates guiding versus free hand technique for cervical lateral mass screw fixation: A prospective study . J Clin Neurosci . 2020 ; 78 : 252 – 258 . Crossref PubMed Google Scholar
26. Merc M , Drstvensek I , Vogrin M , Brajlih T , Recnik G . A multi-level rapid prototyping drill guide template reduces the perforation risk of pedicle screw placement in the lumbar and sacral spine . Arch Orthop Trauma Surg . 2013 ; 133 ( 7 ): 893 – 899 . Crossref PubMed Google Scholar
27. Wu Q , Yu T , Lei B , Huang W , Huang R . A New Individualized Three-Dimensional Printed Template for Lateral Ankle Ligament Reconstruction . Med Sci Monit . 2020 ; 26 : e922925 . Crossref PubMed Google Scholar
28. Hasan S , van Hamersveld KT , Marang-van de Mheen PJ , Kaptein BL , Nelissen RGHH , Toksvig-Larsen S . Migration of a novel 3D-printed cementless versus a cemented total knee arthroplasty: two-year results of a randomized controlled trial using radiostereometric analysis . Bone Joint J . 2020 ; 102-b ( 8 ): 1016 – 1024 . Crossref PubMed Google Scholar
29. Zhang YZ , Lu S , Zhang HQ , et al. Alignment of the lower extremity mechanical axis by computer-aided design and application in total knee arthroplasty . Int J Comput Assist Radiol Surg . 2016 ; 11 ( 10 ): 1881 – 1890 . Crossref PubMed Google Scholar
30. Sun M-L , Zhang Y , Peng Y , Fu D-J , Fan H-Q , He R . Accuracy of a novel 3D-printed patient-specific intramedullary guide to control femoral component rotation in total knee arthroplasty . Orthop Surg . 2020 ; 12 ( 2 ): 429 – 441 . Crossref PubMed Google Scholar
31. Du H , Tian X , Li T , et al. Use of patient-specific templates in hip resurfacing arthroplasty: experience from sixteen cases . Int Orthop . 2013 ; 37 ( 5 ): 777 – 782 . Crossref PubMed Google Scholar
32. Wei F , Xu N , Li Z , et al. A prospective randomized cohort study on 3D-printed artificial vertebral body in single-level anterior cervical corpectomy for cervical spondylotic myelopathy . Ann Transl Med . 2020 ; 8 ( 17 ): 1070 . Crossref PubMed Google Scholar
33. Yin H-W , Feng J-T , Yu B-F , Shen Y-D , Gu Y-D , Xu W-D . 3D printing-assisted percutaneous fixation makes the surgery for scaphoid nonunion more accurate and less invasive . J Orthop Translat . 2020 ; 24 : 138 – 143 . Crossref PubMed Google Scholar
34. Morgan C , Khatri C , Hanna SA , Ashrafian H , Sarraf KM . Use of three-dimensional printing in preoperative planning in orthopaedic trauma surgery: A systematic review and meta-analysis . World J Orthop . 2020 ; 11 ( 1 ): 57 – 67 . Crossref PubMed Google Scholar
35. Pham MT , Rajić A , Greig JD , Sargeant JM , Papadopoulos A , McEwen SA . A scoping review of scoping reviews: advancing the approach and enhancing the consistency . Res Synth Methods . 2014 ; 5 ( 4 ): 371 – 385 . Crossref PubMed Google Scholar
36. Yan L , Wang P , Zhou H . 3D Printing Navigation Template Used in Total Hip Arthroplasty for Developmental Dysplasia of the Hip . Indian J Orthop . 2020 ; 54 ( 6 ): 856 – 862 . Crossref PubMed Google Scholar
37. Di Laura A , Henckel J , Hothi H , Hart A . Can 3D surgical planning and patient specific instrumentation reduce hip implant inventory? A prospective study . 3D Print Med . 2020 ; 6 ( 1 ): 25 . Crossref PubMed Google Scholar
38. Hughes AJ , DeBuitleir C , Soden P , et al. 3D Printing Aids Acetabular Reconstruction in Complex Revision Hip Arthroplasty . Adv Orthop . 2017 ; 2017 : 8925050 . Crossref PubMed Google Scholar
39. Zeng C , Xing W , Wu Z , Huang H , Huang W . A combination of three-dimensional printing and computer-assisted virtual surgical procedure for preoperative planning of acetabular fracture reduction . Injury . 2016 ; 47 ( 10 ): 2223 – 2227 . Crossref PubMed Google Scholar
40. Bagaria V , Chaudhary K . A paradigm shift in surgical planning and simulation using 3Dgraphy: Experience of first 50 surgeries done using 3D-printed biomodels . Injury . 2017 ; 48 ( 11 ): 2501 – 2508 . Crossref PubMed Google Scholar
41. Ballard DH , Mills P , Duszak R , Weisman JA , Rybicki FJ , Woodard PK . Medical 3D Printing Cost-Savings in Orthopedic and Maxillofacial Surgery: Cost Analysis of Operating Room Time Saved with 3D Printed Anatomic Models and Surgical Guides . Acad Radiol . 2020 ; 27 ( 8 ): 1103 – 1113 . Crossref PubMed Google Scholar
42. Msallem B , Beiglboeck F , Honigmann P , Jaquiéry C , Thieringer F . Craniofacial Reconstruction by a Cost-Efficient Template-Based Process Using 3D Printing . Plast Reconstr Surg Glob Open . 2017 ; 5 ( 11 ): e1582 . Crossref PubMed Google Scholar
43. Wong RMY , Choy VMH , Li J , et al. Fibrinolysis as a target to enhance osteoporotic fracture healing by vibration therapy in a metaphyseal fracture model . Bone Joint Res . 2021 ; 10 ( 1 ): 41 – 50 . Crossref PubMed Google Scholar
44. Wong RMY , Ho WT , Wai LS , et al. Fragility fractures and imminent fracture risk in Hong Kong: one of the cities with longest life expectancies . Arch Osteoporos . 2019 ; 14 ( 1 ): 104 . Crossref PubMed Google Scholar
45. Shen S , Wang P , Li X , Han X , Tan H . Pre-operative simulation using a three-dimensional printing model for surgical treatment of old and complex tibial plateau fractures . Sci Rep . 2020 ; 10 ( 1 ): 6044 . Crossref PubMed Google Scholar
46. Wan RC , Chau WW , Tso CY , et al. Occupational hazard of fluoroscopy: An invisible threat to orthopaedic surgeons . Journal of Orthopaedics, Trauma and Rehabilitation . 2021 ; 28 : 221049172110355 . Google Scholar
47. Hayda RA , Hsu RY , DePasse JM , Gil JA . Radiation Exposure and Health Risks for Orthopaedic Surgeons . J Am Acad Orthop Surg . 2018 ; 26 ( 8 ): 268 – 277 . Crossref PubMed Google Scholar
48. Kaplan DJ , Patel JN , Liporace FA , Yoon RS . Intraoperative radiation safety in orthopaedics: a review of the ALARA (As low as reasonably achievable) principle . Patient Saf Surg . 2016 ; 10 : 27 . Crossref PubMed Google Scholar
49. The 2007 Recommendations of the International Commission on Radiological Protection. ICRP publication 103 . Ann ICRP . 2007 ; 37 ( 2–4 ): 1 – 332 . Crossref PubMed Google Scholar
50. Rajagopal K , Ramesh S , Walter NM , Arora A , Katti DS , Madhuri V . In vivo cartilage regeneration in a multi-layered articular cartilage architecture mimicking scaffold . Bone Joint Res . 2020 ; 9 ( 9 ): 601 – 612 . Crossref PubMed Google Scholar
51. Filardo G , Petretta M , Cavallo C , et al. Patient-specific meniscus prototype based on 3D bioprinting of human cell-laden scaffold . Bone Joint Res . 2019 ; 8 ( 2 ): 101 – 106 . Crossref PubMed Google Scholar
52. McMenamin PG , Quayle MR , McHenry CR , Adams JW . The production of anatomical teaching resources using three-dimensional (3D) printing technology . Anat Sci Educ . 2014 ; 7 ( 6 ): 479 – 486 . Crossref PubMed Google Scholar
53. Garcia J , Yang Z , Mongrain R , Leask RL , Lachapelle K . 3D printing materials and their use in medical education: a review of current technology and trends for the future . BMJ Simul Technol Enhanc Learn . 2018 ; 4 ( 1 ): 27 – 40 . Crossref PubMed Google Scholar
54. McMenamin PG , Quayle MR , McHenry CR , Adams JW . The production of anatomical teaching resources using three-dimensional (3D) printing technology . Anat Sci Educ . 2014 ; 7 ( 6 ): 479 – 486 . Crossref PubMed Google Scholar
55. Fasel JHD , Aguiar D , Kiss-Bodolay D , et al. Adapting anatomy teaching to surgical trends: a combination of classical dissection, medical imaging, and 3D-printing technologies . Surg Radiol Anat . 2016 ; 38 ( 3 ): 361 – 367 . Crossref PubMed Google Scholar
56. Tack P , Victor J , Gemmel P , Annemans L . 3D-printing techniques in a medical setting: a systematic literature review . Biomed Eng Online . 2016 ; 15 ( 1 ): 115 . Crossref PubMed Google Scholar
57. Wang C , Zhang L , Qin T , et al. 3D printing in adult cardiovascular surgery and interventions: a systematic review . J Thorac Dis . 2020 ; 12 ( 6 ): 3227 – 3237 . Crossref PubMed Google Scholar
58. Kong X , Nie L , Zhang H , et al. Do 3D printing models improve anatomical teaching about hepatic segments to medical students? a randomized controlled study . World J Surg . 2016 ; 40 ( 8 ): 1969 – 1976 . Crossref PubMed Google Scholar
59. Huang W , Zhang X . 3D Printing: Print the future of ophthalmology . Invest Ophthalmol Vis Sci . 2014 ; 55 ( 8 ): 5380 – 5381 . Crossref PubMed Google Scholar
Author contributions
R. M. Y. Wong: Principal investigator. Conceptualization, Data curation, Formal analysis, Methodology, Validation, Writing – original draft, Writing – review & editing, Supervision.
P. Y. Wong: Writing – original draft, Formal analysis, Data curation, Writing – review & editing.
C. Liu: Formal analysis, Writing – original draft, Writing – review & editing.
Y. L. Chung: Writing – review & editing, Formal analysis.
K. C. Wong: Validation, Supervision, Writing – review & editing, Conceptualization.
C. Y. Tso: Validation, Writing – review & editing, Conceptualization.
S. K. Chow: Conceptualization, Validation, Writing – review & editing.
W. Cheung: Validation, Writing – review & editing.
P. S-H. Yung: Supervision, Writing – review & editing.
C. S. Chui: Supervision, Validation, Writing – review & editing.
S. W. Law: Supervision, Writing – review & editing, Visualization.
Funding statement
This research received no specific grant from any funding agency in the public, commercial or not-for-profit sectors. No benefits in any form have been received or will be received from a commercial party related directly or indirectly to the subject of this article.
ICMJE COI statement
No conflict of interest exits in the submission of this manuscript, and the manuscript is approved by all authors for publication.
Supplementary material
The characteristics of the randomized controlled trial studies, subgroup analysis figures, and trial sequential analysis figure.
Open access funding
The authors confirm that the open access fee for this study was self-funded.