Abstract
Aims
The processes linking long-term bisphosphonate treatment to atypical fracture remain elusive. To establish a means of exploring this link, we have examined how long-term bisphosphonate treatment with prior ovariectomy modifies femur fracture behaviour and tibia mass and shape in murine bones.
Methods
Three groups (seven per group) of 12-week-old mice were: 1) ovariectomized and 20 weeks thereafter treated weekly for 24 weeks with 100 μm/kg subcutaneous ibandronate (OVX+IBN); 2) ovariectomized (OVX); or 3) sham-operated (SHAM). Quantitative fracture analysis generated biomechanical properties for the femoral neck. Tibiae were microCT scanned and trabecular (proximal metaphysis) and cortical parameters along almost its whole length measured.
Results
Fracture analyses revealed that OVX+IBN significantly reduced yield displacement (vs SHAM/OVX) and resilience, and increased stiffness (vs SHAM). OVX+IBN elevated tibial trabecular parameters and also increased cortical cross-sectional area and second moment of area around minor axis, and diminished ellipticity proximally.
Conclusion
These data indicate that combined ovariectomy and bisphosphonate generates cortical changes linked with greater bone brittleness and modified fracture characteristics, which may provide a basis in mice for interrogating the mechanisms and genetics of atypical fracture aetiology.
Cite this article: Bone Joint Open 2020;1-9:512–519.
Introduction
Atypical fractures (AF) are a rare yet catastrophic phenomenon involving failure following minimal bone trauma. Although incidence correlates strongly with long-term bisphosphonate use, AF pathophysiology is poorly described, and treatment and prevention strategies are elusive.1-4 It is speculated that suppression of remodelling by long-term bisphosphonate use increases bone brittleness, resulting in inefficient dissipation of the energy imposed by even relatively minor loads and hence crack propagation and fracture.1-3,5
Bisphosphonates inhibit resorption and can rapidly modify bone architecture and quality.6,7 Use in rodents has demonstrated their role in preserving cortical and trabecular microstructure.8,9 Their prolonged administration can, however, generate contrary outcomes, with brittle bone susceptible to failure with minimal deformation.1,2,5,7,10 There are various explanations for bisphosphonate-related weakening; observations in osteoporotic patients suggest that long-term bisphosphonate administration impairs osteoclast-mediated repair of microdamage to promote microcrack accumulation.2,3,5,11 Treatment is also likely to influence the bone’s properties directly, increasing mineralization levels and altering intrinsic resistance to bending without failure.1,2,4 Bisphosphonates induce similar deleterious energy absorption and toughness reductions in rat femoral bone and alter bone mechanical properties in dog models.4,10 Suppression of bone turnover in these models also leads to bone matrix collagen crosslinking to negatively impact biomechanical properties, particularly post-yield deformation.4,12
Clinical observations in bisphosphonate-treated patients highlight that changes in bone shape could be another risk factor,1,2 as have specific gene polymorphisms in human cohort-based analyses.13 There are reports of modified geometry and increased femoral bowing, which influence local mechanical strain directly,1,2,14 emphasizing the role of modelling activity in maintaining shape and mechanical integrity of normal, fracture-resistant bone.15 The precise shape changes driven by long-term bisphosphonates, and their mechanical consequences, need therefore to be explored to gain a thorough understanding of AF susceptibility.2,14,15 Imaging advances now allow investigation of subtle changes in murine tibia shape,16,17 and their relevance to age-related bone loss in humans has been emphasized.16 This makes the study of bone shape appealing, particularly in the experimental context where bisphosphonates may also provoke AF. Clinical observations would clearly be enriched by in vivo experiments in mice where all the stages predisposing to AF development could be studied.
AF is currently only limited by imposing a bisphosphonate ‘drug holiday’ for microcrack repair and shape correction. An animal model that compellingly recapitulates events which contribute to AF would clearly improve prevention and treatment strategies, and would provide a basis from which gene polymorphisms.18,19 Herein, we address the hypothesis that long-term bisphosphonate exposure in ovariectomized mice provokes changes in bone strength, quantity and geometry that effectively mimic those culminating in AF susceptibility. We examine whether such treatment provokes bone strength changes that culminate in modified fracture behaviours in an established model of ovariectomy-induced osteoporosis, and whether this combined treatment induces tibia shape changes consistent with an increased mechanical fracture vulnerability.
Methods
Animals, anaesthesia, surgery, and treatment
In all, 21 12-week-old female Swiss mice were randomly allocated into three groups (seven per group), housed in groups of seven in polypropylene cages with temperature maintained between 19°C to 23°C, 12-hour light/dark cycle with ad libitummaintenance mice diet (rats and mice, Presence, Paulinia, Brazil) and water. Surgery involved pre-medication with 10 mg/kg meperidine (Dolosal 50 mg/ml; Cristália Itapira, Brazil), 2 mg/kg meloxicam (Maxican 2%; Ourofino Pet Cravinhos, Brazil) delivered subcutaneously with isoflurane-induced anaesthesia (Isoforine; Cristália Itapira, Brazil), followed by right flank incision and abdominal cavity access without ovariectomy (SHAM), removal of ovaries (OVX), or ovary removal followed 20 weeks post-surgery (aged 32 weeks) by an additional 24-week ibandronate treatment (OVX+IBN). SHAM and OVX groups received weekly subcutaneous saline injection, while OVX+IBN received 100 μg/kg weekly subcutaneous ibandronate (Bonviva 3 mg/3 ml; Roche, Rio de Janeiro, Brazil).18,20 All mice were killed by cervical dislocation aged 56 weeks, weighed and the femur and right tibia subsequently dissected free of soft tissue and frozen (-80°C) or fixed in neutral-buffered formaldehyde for 24 hours before storage in 70% EtOH, respectively.
Assessment of femoral head biomechanical properties
Femoral neck strength was evaluated by compression until failure, using established methods.21,22 Briefly, after defrosting, the distal half of the femur was dissected and the proximal segment diaphysis embedded in acrylic base, allowing loading of femur head parallel to the diaphysis (Figure 1).22 Fracture tests were performed in a universal testing machine (Instron Model 5565, Canton, Massachusetts, USA) using a cylindrical jig with the femoral head travelling at 0.5 mm/min until neck failure with 2 Hz data sampling. Displacement and force were recorded and yield displacement (YD), stiffness, ultimate load (UL) before fracture and resilience calculated for each sample. Stiffness was evaluated from the linear elastic phase of the force-displacement curves (angular coefficient, R2 > 0.99 was considered appropriate) and resilience, via YD and force (resilience = (YD× yield force)/2).
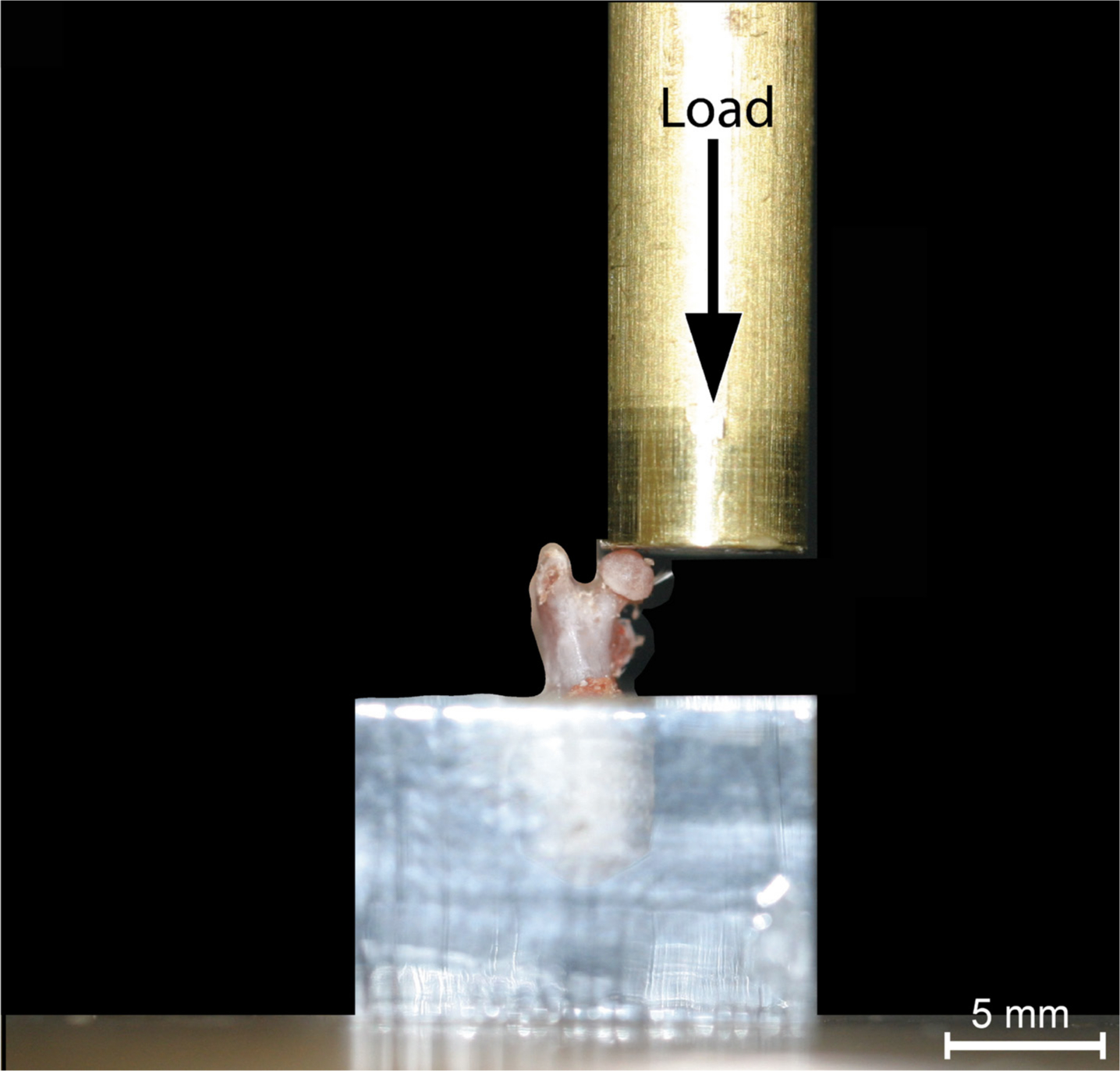
Fig. 1
The positioning of the femur in the universal materials testing machine prior to compression until failure. The black arrow represents the direction of force applied.
Assessment of bone mass and shape changes in tibial cortical and trabecular compartments
To evaluate treatment effects on bone shape and mass, each entire tibia was scanned using radiograph microcomputed tomography (microCT; Skyscan 1172, Kontich, Belgium) with 0.5 mm aluminium filter, medium camera, 5 μm voxel size, tube operated at 50kv/200μa and 960 ms exposure time. Slices were reconstructed using NRecon 1.7.1.0 and length measured with CTAn software. Cortical bone quantity and shape along the tibia length (15% to 85%, proximodistally) was also evaluated.17 After alignment in DataViewer, images were imported to ImageJ and the fibula removed. BoneJ plugin was used (grayscale threshold of 100) to measure cross-sectional area (CSA), average cortical thickness (Ct.Th), second moment of area around major/minor axes (Imin/Imax), predicted resistance to torsion (J) and ellipticity. Tissue mineral density (TMD) was measured following appropriate calibration.
For trabecular bone, the appearance of the trabecular ‘bridge’ connecting the paired primary spongiosa ‘islands’ was set as the reference point for analysis of proximal metaphyseal bone; 5% of total bone length (from this point towards diaphysis) was selected for evaluation of trabecular number (Tb.N), thickness (Tb.Th), separation (Tb.Sp), bone volume/trabecular volume (BV/TV) and mineral density (BMD), by calibrated microCT.17
Statistical analysis
All data (except body mass and length) were weight-corrected using a linear regression method,23,24 tested by Shapiro-Wilk to verify adherence to normal distribution and differences in body mass, femoral YD, stiffness, UL, and resilience, and tibia trabecular parameters evaluated by one-way analysis of variance (ANOVA) followed by Tukey’s post-hoc test. Parameters failing normality tests (p < 0.05) were analyzed by Kruskal-Wallis test followed by a Dunn’s test. All analyses were performed using Prism 7 (GraphPad Software, San Diego, California, USA) and significance set at p < 0.05. Tibia cortical parameters were assessed by one-way ANOVA followed by Tukey’s post-test (R software, version 3.4.4.4) and distinct levels of significance set at p < 0.001, 0.001< p < 0.01 and 0.01 < p < 0.05.
Results
Tibia length measurements showed that treatment did not modify the extent of longitudinal growth during the 44-week study duration (SHAM, OVX, and OVX+IBN were 20.08 mm ± 0.12 mm, 20.23 mm ± 0.16 mm, and 20.27 mm ± 0.14 mm respectively). OVX and particularly OVX+IBN groups did, however, show a tendency for raised body mass (SHAM, OVX, and OVX+IBN were 43.86 gm ± 2.83 gm, 50.57 gm ± 3.1 gm, and 52.71 gm ± 0.96 gm respectively); accordingly, all further evaluations were subjected to weight-correction using a linear regression method.23
Combined ibandronate/ovariectomy increases femoral brittleness
Evaluation of force/displacement curves from a compressive load fracture method showed that femora in the OVX+IBN group exhibited ~40% lower YD (vs SHAM/OVX, reduction) and greater stiffness (vs SHAM; OVX vs SHAM not different; Figure 2a and b). Ultimate (maximum) load-to-fracture – a measure of strength – did not differ in OVX or OVX+IBN groups compared to SHAM (Figure 2c). Consistent with greater brittleness, OVX+IBN treatment significantly elevates neck stiffness (vs SHAM; Figure 2d). Femur resilience was also significantly lower (~47% reduction) in OVX+IBN than SHAM group (Figure 3).
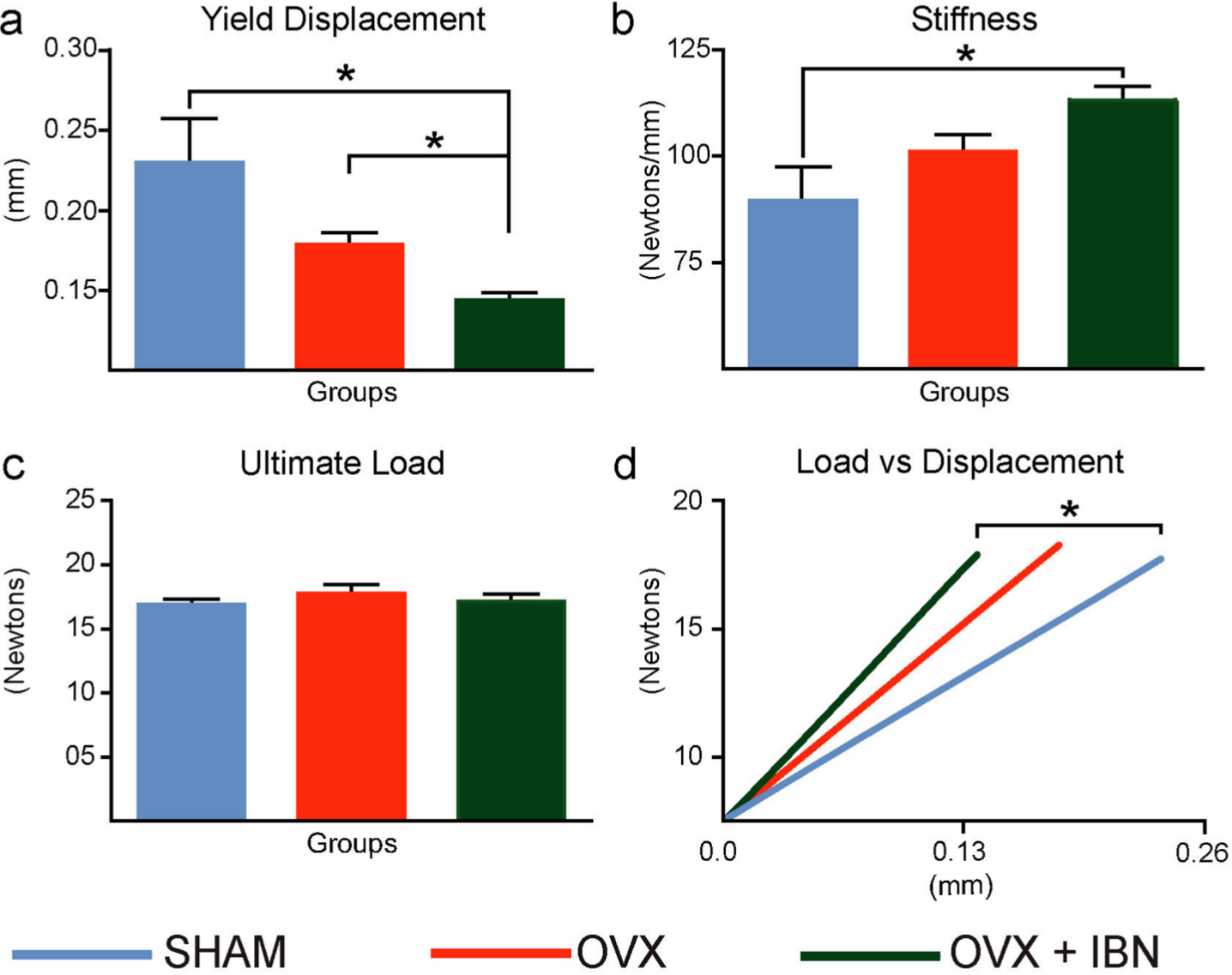
Fig. 2
Effect of ibandronate/ovariectomy treatmet on biomechanical properties. Measurement (mean ± SE) of yield displacement (mm): (a) stiffness (Newtons/mm), (b), ultimate load (Newtons, (c) and average load, displayed as a function of displacement, across a linear portion of the load/displacement curve (d) in mouse femoral neck fracture model in sham-operated mice (SHAM), ovariectomized (OVX) mice receiving subcutaneous saline solution and ovariectomized mice treated weekly, from 20 weeks, for 24 weeks with subcutaneous ibandronate (Bonviva, 1 mg/ml, Roche, 100 μm/kg; OVX+IBN). Group size was seven per group. Stiffness corresponded to the Shapiro-Wilk test for normality and was analyzed through ANOVA followed by Tukey test. Yield displacement and ultimate load failed to normality tests (p < 0.05) and were analyzed by Kruskal-Wallis test followed by a Dunn’s test. *Statistical significance (p < 0.05).
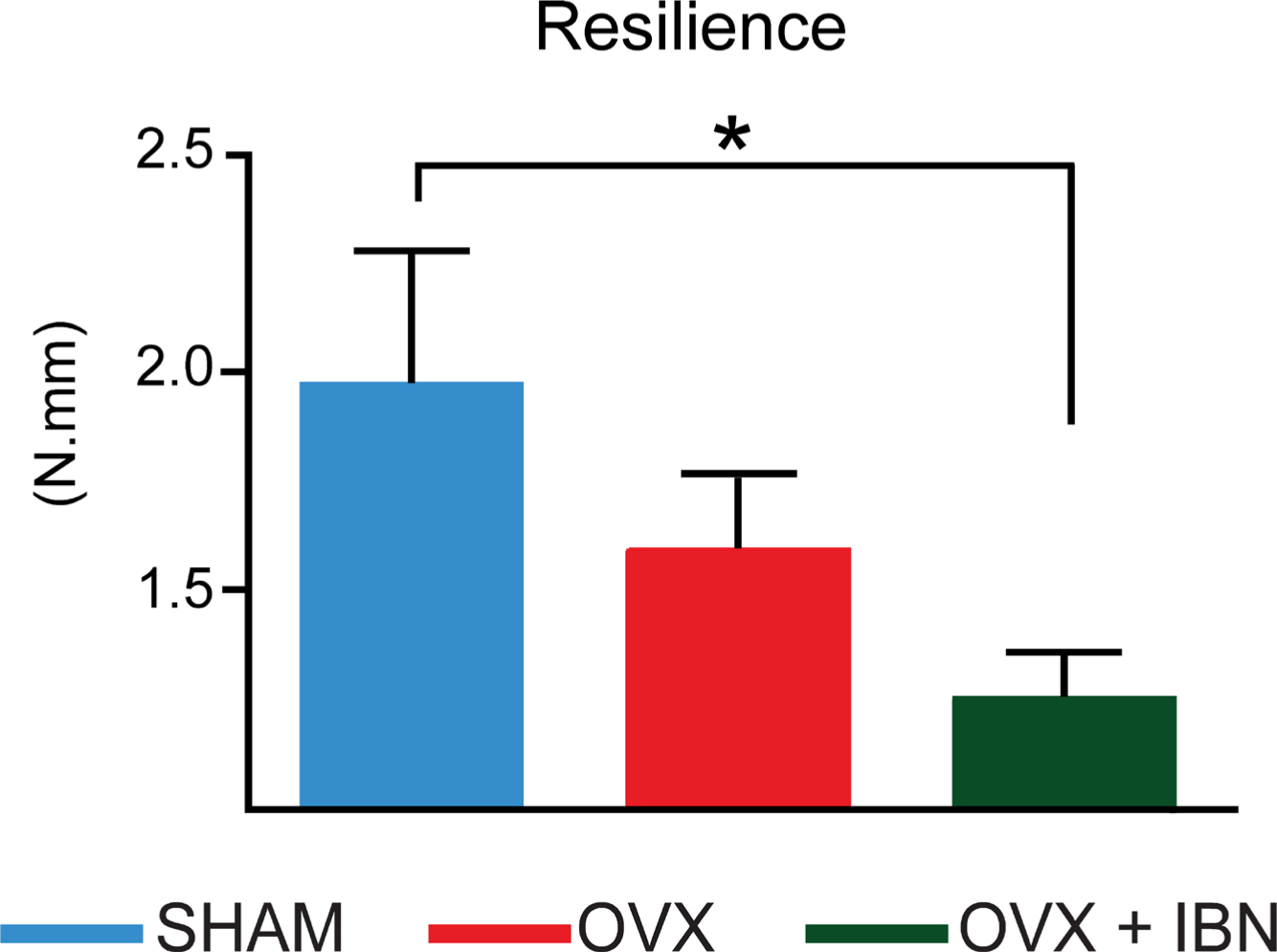
Fig. 3
Effect of ibandronate/ovariectomy treatment on biomechanical properties. Measurement (mean ± SE) of resilience (N.mm) in mouse femoral neck fracture model in sham-operated mice (SHAM), ovariectomized (OVX) mice receiving subcutaneous saline solution and ovariectomized mice treated weekly, from 20 weeks, for 24 weeks with subcutaneous ibandronate (Bonviva®, 1 mg/ml, Roche, 100 μm/kg; OVX+IBN). Group size was seven per group. Resilience failed to normality tests (p < 0.05) and was analyzed by Kruskal-Wallis test followed by a Dunn’s test. *Statistical significance (p < 0.05).
Combined ibandronate/ovariectomy modifies cortical mass and shape in specific tibia regions
Evaluation along tibial length revealed that OVX+IBN elevated cortical CSA (vs OVX) in the proximal diaphysis (~20% to 30%; Figure 4a). In contrast, neither Ct.Th nor TMD were modified by OVX+IBN or OVX treatment. Superimposition of changes at 35% of length highlight cortical shape changes between SHAM, OVX and OVX+IBN groups (Figure 4b); while Imin and J did not differ across these groups, Imax was significantly greater, and ellipticity lower along proximal regions in OVX+IBN mice (~30% to 45% vs OVX/SHAM; Figure 5a and b). These data indicate that OVX+IBN generates greater cortical mass and straighter shape at localized tibial regions.
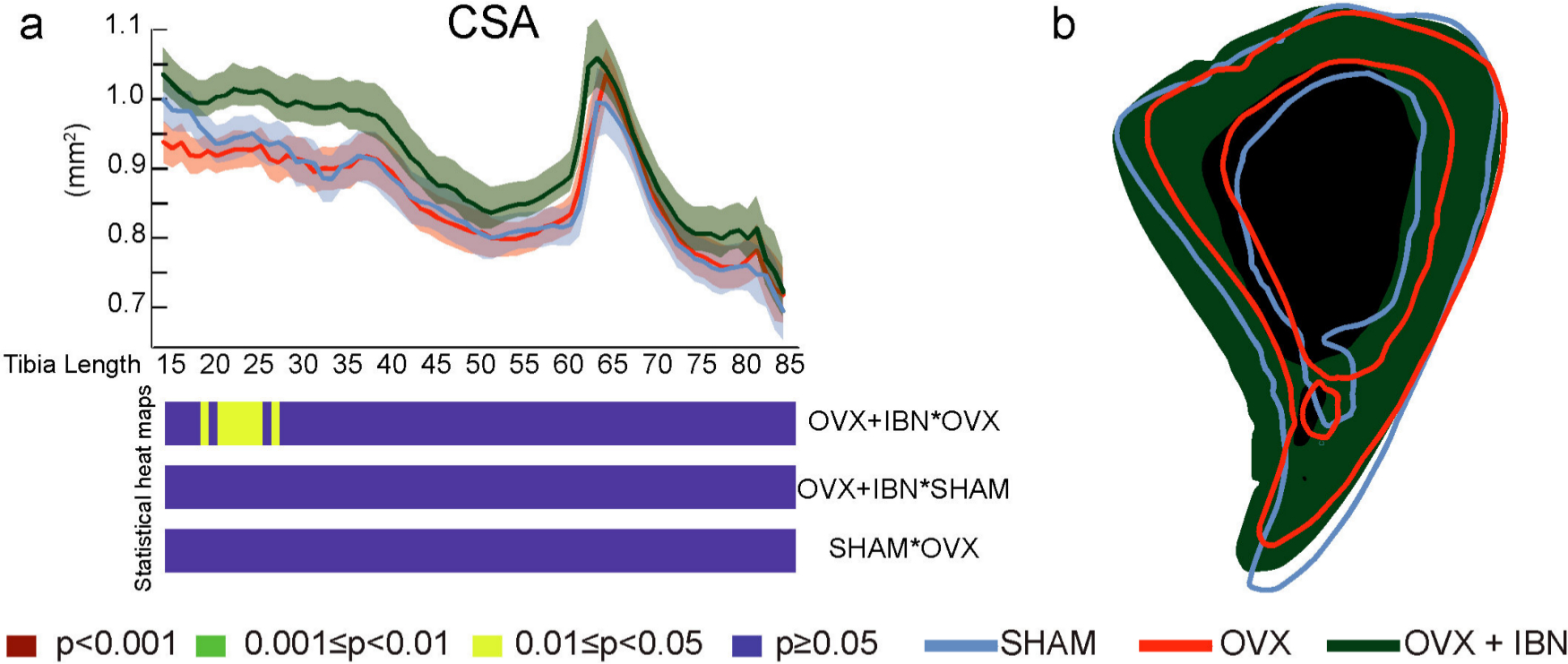
Fig. 4
Effects of ibandronate/ovariectomy treatment on cortical mass in the tibia. Measurement (mean ± SE) and t-test heat map of cross-sectional area (CSA, mm2, a) in mouse tibia along its diaphyseal length (15% to 85%). b: slice at 35% of length of representative tibia from sham-operated mice (SHAM, blue), ovariectomized (OVX, red) mice receiving subcutaneous saline solution and ovariectomized mice treated weekly, from 20 weeks, for 24 weeks with subcutaneous ibandronate (Bonviva, 1 mg/ml, Roche, 100 μm/kg; OVX + IBN, green). Group size was seven per group; All data corresponded to the Shapiro-Wilk test for normality and was analyzed through ANOVA followed by Tukey test. Levels of statistical significance set at p < 0.001 (red), 0.001 < p <0.01 (green) and 0.01< p <0.05 (yellow); p > 0.05 (blue).
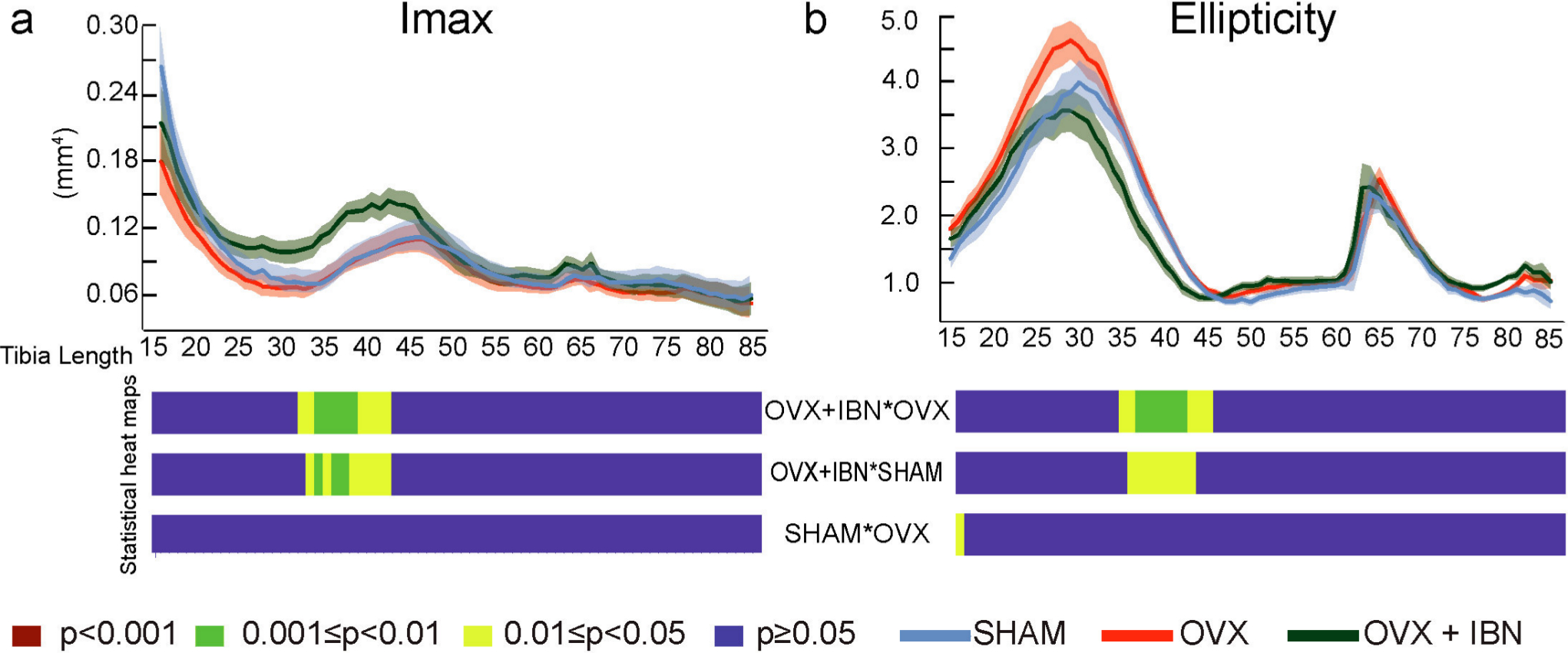
Fig. 5
Effect of ibandronate/ovariectomy treatment on the cortical bone shape in the tibia. Measurement (mean ± SE) and t-test heat map for second moment of area around minor axes (Imax, mm4, a) and ellipticity (b) at 15% to 85% of tibia length in sham-operated mice (SHAM), ovariectomized mice receiving subcutaneous saline solution (OVX) and ovariectomized mice treated weekly, from 20 weeks, for 24 weeks with subcutaneous ibandronate (Bonviva®, 1 mg/ml, Roche, 100 μm/kg; OVX+IBN). Group size was seven per group; All data corresponded to the Shapiro-Wilk test for normality and was analyzed through ANOVA followed by Tukey test. Levels of statistical significance set at p < 0.001 (red), 0.001< p < 0.01 (green) and 0.01 < p < 0.05 (yellow); p > 0.05 (blue).
Combined ibandronate/ovariectomy increases trabecular mass and mineral density
Trabecular architecture was not significantly modified 44 weeks after ovariectomy (vs SHAM) and only Tb.Sp was significantly different in OVX+IBN compared with SHAM (Table I). In contrast, OVX+IBN resulted in significantly greater BV/TV, Tb.N and BMD, and less Tb.Sp than was evident on OVX mice.
Table I.
Combined ibandronate/ovariectomy treatment increases trabecular bone mass and mineral density.
Parameter | SHAM, mean ± SE | OVX, mean ± SE | OVX+IBN, mean ± SE |
---|---|---|---|
Tb.N (mm-1) | 1.04 ± 0.23*† | 0.89 ± 0.30* | 1.33 ± 0.24† |
Tb.Th (mm) | 0.05 ± 0.00* | 0.05 ± 0.00* | 0.05 ± 0.00* |
Tb.Sp (mm) | 0.48 ± 0.07* | 0.51 ± 0.07* | 0.33 ± 0.05† |
BV/TV (%) | 5.81 ± 1.46*† | 5.05 ± 1.47* | 7.39 ± 1.26† |
BMD (g/cm-3) | 0.09 ± 0.03*† | 0.07 ± 0.02* | 0.12 ± 0.01† |
-
Group size seven per group. Mice receiving subcutaneous saline solution and ovariectomized mice treated weekly, from 20 weeks, for 24 weeks with subcutaneous Ibandronate (Bonviva, 1 mg/ml, Roche, 100 μm/kg; OVX+IBN). All data corresponded to the Shapiro-Wilk test for normality and was analyzed through ANOVA followed by Tukey test. Groups annotated with the same symbol are not statistically different; p < 0.05 in groups annotated with different symbols.
-
BMD, bone mineral density; BV/TV; bone volume/trabecular volume; OVX, ovariectomized; SE, standard error; SHAM, sham-operated mice; Tb.N, trabecular number; Tb.Sp, trabecular separations; Tb.Th, trabecular thickness.
Discussion
Our data show that combined OVX+IBN treatment reduces YD and resilience, and increases femoral stiffness/brittleness without modifying ultimate load. We also report greater tibial CSA and Imax, and decreased ellipticity proximal to the mid-shaft in OVX+IBN treated mice, consistent with regional cortical mass and shape modification. OVX-induced trabecular bone loss was, as expected, restricted by additional IBN treatment. Emergence of greater brittleness, cortical thickening, and shape changes make it tempting to speculate that OVX+IBN treatment in mice allows for the study of AF-type pathophysiology.
These skeletal deficiencies emerge 20 weeks after OVX, followed by an additional 24-week ibandronate treatment period. Despite immaturity at study onset, mice will have attained skeletal maturity while estrogen-deplete, before ibandronate treatment, which only finishes at ~one-year-old.16 It is difficult to fully reconcile our observations as studies into the influence of bisphosphonates on mouse bone fracture properties are limited. Elegant studies by Aref et al25 used femoral four-point bending to explore if bone properties were modified by zoledronate in divergent mouse strains, finding that C57/Bl6 but not A/J mice exhibit changes in YD.25 As C57/B6 also exhibited greater femoral bone area, it was suggested that geometry was a determinant of bone’s response to bisphosphonate. Our studies support this conclusion, demonstrating that OVX+IBN increases brittleness and reduces femoral YD more than OVX alone.
It is noteworthy that our studies apply loads parallel to the diaphysis and thus evaluate femoral biomechanical properties under more physiological conditions.21,22 We tentatively suggest that OVX+IBN will help identify strength/geometry changes that occur during AF development. If mouse genotype is a major determinant,25,26 future studies would ideally examine bisphosphonate responses in divergent strains and endorse a genetic predisposition to AF.13
Lower YD and resilience with greater stiffness after OVX+IBN is consistent with the greater brittleness reported after long-term bisphosphonate treatment in humans.1,5,11 They are also in keeping with AF following long-term ibandronate in other patient groups;27,28 with femoral neck AF after long-term risedronate delivery29 and the notion that highly mineralised bone has less toughness and faster fracture propagation.1,5,11,30 This may be an amalgamation of mineral content, mass, and shape changes, and since we were not able to microCT the femora, we cannot rule out shape change contributions to OVX+IBN-related alterations in fracture characteristics. Examination of rat femur fracture properties after ovariectomy demonstrates that stiffness changes could be metabolically underpinned.21 It is therefore conceivable that associated bone shape changes will be discernible elsewhere in the skeleton. Our studies show that there are indeed tibial shape changes, with greater CSA and Imax following OVX+IBN. Future studies will need to correlate changes in femoral brittleness to analysis of femoral neck bone mass and shape after OVX+IBN treatment, and also include studies in SHAM-IBN mice in order to allow for more definitive conclusions to be drawn.
Likely impact of tibia-femoral angles in femur AF led us to explore tibia architectural changes with OVX+IBN.14 Bone shape deviation can result from modified mechanical strains, pharmacological influences on modelling or pathology.15,31 Indeed, bisphosphonates can alter mouse bone modelling, leading to femoral cortex shape changes.18 In agreement, we find that OVX+IBN generates greater CSA and Imax, as well as lower ellipticity (straightening) – shape changes linked to lower load predictability.32 Finite element analyses have shown that the site of AF in human femora coincides with region of high strain.33 It is intriguing that the tibia shape changes we find also coincide with reported high strain regions.34 As human femora with particular shape profiles are predisposed to AF,1,2,33 we speculate that the shape changes after OVX+IBN likewise reduce load predictability to increase fracture risk, affording a model for checking new therapies to limit AF development.19 OVX+IBN treatment of murine models with known genetic AF predisposition coupled with the monitoring of these shape changes may help to refine our understanding of AF risks.
We also examined proximal tibia metaphysis trabecular bone; a focus region for many studies. Song et al35 found most marked effects after eight to 16 weeks of OVX in this region (in eight-week-old mice), which were much less marked later, suggesting that prolongation does not expand the bone losses. Advanced age and the 44-week timescale post-OVX16,35 in our study may not, therefore, be ideal and may underpin the lack of any overt OVX-induced difference. It is key, however, that we evaluated long-term bisphosphonate effects as our aim was to discern whether this aligned with clinical observations of modified strength and fracture susceptibility. In osteoporotic patients, bisphosphonates limit remodelling to restrict further losses in bone mass.6,7 This aligns with ibandronate-related protection against OVX-induced bone changes in both femora and vertebrae in aged rats.9 In our study, ibandronate delivery commencing 20 weeks after OVX similarly sustains trabecular number, BV/TV and BMD at SHAM levels; consistent with the notion that ibandronate limits rapid declines in mass and quality that follow OVX.
The methods used to measure bone mass and mechanical properties are of central importance; for example, both are influenced by body mass.23,24 We have used the methods of Jepsen et al23 to control for body mass, his normalization employs linear regression to correct for even small mass differences,23 and is precautionary since tibia length is not modified between groups and correction based upon length would be superfluous. We studied responses to compressive load in the femoral neck and performed whole bone microCT analysis in tibiae, as the latter aligned with previous studies examining response to pharmacological treatment.22
There are several caveats pertinent to our findings. First, it is difficult to fully appreciate the OVX+IBN effects, without a group treated with IBN alone and the lack of trabecular or cortical changes in OVX mice. Our aim, however, was to recapitulate post-menopausal osteopenia treated clinically with long-term ibandronate, rather than to explore OVX or IBN effects; whether modified fracture properties and greater brittleness are induced by long-term IBN alone remains to be seen. The modified mechanical properties in OVX+IBN are nonetheless consistent with an AF-like phenotype. Secondly, many human AF studies have centred upon microcracks.5,11 In an attempt to pinpoint microcrack incidence in OVX+IBN treated bones, we scanned at high resolution (1.3 μm voxel as used previously;11 tibia shaft regions showing distinct shape responses (25% to 45% and 60% to 85%)) to OVX+IBN, yet this failed to disclose microcracks. This aligns with studies reporting microdamage as extremely rare in mice.25 Regardless, long-term bisphosphonates in humans precipitate an accumulation of microcracks that may diminish fracture strength.2,5,11 Future work should explore if microdamage, stress fracture, or periosteal reactions are seen after OVX+IBN treatment. Thirdly, the necessity that biomechanical analyses require fresh, unfixed femora36 precludes their use prior microCT evaluation, which itself requires samples are fixed to preserve integrity; lack of co-registration of mechanical and structural properties in a single bone is clearly a limitation. Finally, absence of murine intracortical remodelling makes direct comparison to human bone impossible.2,4,16 Nonetheless, mice model their bones to maintain shape,15 and long-term bisphosphonates effectively exert effects on the incumbent resorption. Mouse models have served an invaluable role in osteoporosis drug development and it is tempting to speculate that predictions regarding ‘drug holiday’ effectiveness may be made by monitoring of modelling related shape changes. Mice harbouring genetic AF predisposing polymorphisms or gene mutations can also be studied using our approach to resolve possible roles for genetics in AF aetiology.
Our data show that extended ibandronate treatment of previously ovariectomized mice modifies bone biomechanical properties to generate a stiffer, more brittle femur neck. OVX+IBN-treated mice also show tibia mass and shape changes consistent with bone straightening and deficient predicted strength. These findings make it tempting to speculate that OVX+IBN treatment in mice is an appropriate experimental setting for the study of AF pathophysiology.
References
1. Ettinger B , Burr DB , Ritchie RO . Proposed pathogenesis for atypical femoral fractures: lessons from materials research . Bone . 2013 ; 55 ( 2 ): 495 – 500 . Crossref PubMed Google Scholar
2. Black DM , Abrahamsen B , Bouxsein ML , et al. Atypical femur fractures: review of epidemiology, relationship to bisphosphonates, prevention, and clinical management . Endocr Rev . 2019 ; 40 ( 2 ): 333 – 368 . Crossref PubMed Google Scholar
3. Shane E , Burr D , Abrahamsen B , et al. Atypical subtrochanteric and diaphyseal femoral fractures: second report of a task force of the American Society for bone and mineral research . J Bone Miner Res . 2014 ; 29 ( 1 ): 1 – 23 . Crossref PubMed Google Scholar
4. Allen MR . Recent advances in understanding bisphosphonate effects on bone mechanical properties . Curr Osteoporos Rep . 2018 ; 16 ( 2 ): 198 – 204 . Crossref PubMed Google Scholar
5. Ma S , Goh EL , Jin A , et al. Long-Term effects of bisphosphonate therapy: perforations, microcracks and mechanical properties . Sci Rep . 2017 ; 7 : 43399 . Crossref PubMed Google Scholar
6. Bock O , Börst H , Beller G , et al. Impact of oral ibandronate 150mg once monthly on bone structure and density in post-menopausal osteoporosis or osteopenia derived from in vivo μCT . Bone . 2012 ; 50 ( 1 ): 317 – 324 . Google Scholar
7. Ward J , Wood C , Rouch K , et al. Stiffness and strength of bone in osteoporotic patients treated with varying durations of oral bisphosphonates . Osteoporos Int . 2016 ; 27 ( 9 ): 2681 – 2688 . Crossref PubMed Google Scholar
8. Takeda S , Sakai S , Tanaka K , et al. Intermittent ibandronate maintains bone mass, bone structure, and biomechanical strength of trabecular and cortical bone after discontinuation of parathyroid hormone treatment in ovariectomized rats . Calcif Tissue Int . 2017 ; 101 ( 1 ): 65 – 74 . Crossref PubMed Google Scholar
9. Bauss F , Lalla S , Endele R , Hothorn LA . Effects of treatment with ibandronate on bone mass, architecture, biomechanical properties, and bone concentration of ibandronate in ovariectomized aged rats . J Rheumatol . 2002 ; 29 ( 10 ): 2200 – 2208 . PubMed Google Scholar
10. Smith ER , Allen MR . Bisphosphonate-Induced reductions in rat femoral bone energy absorption and toughness are testing rate-dependent . J Orthop Res . 2013 ; 31 ( 8 ): 1317 – 1322 . Crossref PubMed Google Scholar
11. Jin A , Cobb J , Hansen U , et al. The effect of long-term bisphosphonate therapy on trabecular bone strength and microcrack density . Bone Joint Res . 2017 ; 6 ( 10 ): 602 – 609 . Crossref PubMed Google Scholar
12. Allen MR , Gineyts E , Leeming DJ , et al. Bisphosphonates alter trabecular bone collagen cross-linking and isomerization in beagle dog vertebra . Osteoporos Int . 2008 ; 19 ( 3 ): 329 – 337 . Crossref PubMed Google Scholar
13. Nguyen HH , van de Laarschot DM , Verkerk AJMH , et al. Genetic risk factors for atypical femoral fractures (AFFs): a systematic review. . JBMR Plus . 2018 ; 2 ( 1 ): 1 – 11 . Crossref PubMed Google Scholar
14. Haider IT , Schneider PS , Edwards WB . The role of lower-limb geometry in the pathophysiology of atypical femoral fracture . Curr Osteoporos Rep . 2019 ; 17 ( 5 ): 281 – 290 . Crossref PubMed Google Scholar
15. Allen MR , Burr DB . Bone modelling and remodelling . In : Burr DB , Allen MR , eds . Basic and applied bone biology . London : Academic Press , 2014 : 75 – 90 . Google Scholar
16. Jilka RL . The relevance of mouse models for investigating age-related bone loss in humans . J Gerontol A Biol Sci Med Sci . 2013 ; 68 ( 10 ): 1209 – 1217 . Crossref PubMed Google Scholar
17. Javaheri B , Razi H , Piles M , et al. Sexually dimorphic tibia shape is linked to natural osteoarthritis in STR/Ort mice . Osteoarthritis Cartilage . 2018 ; 26 ( 6 ): 807 – 817 . Crossref PubMed Google Scholar
18. Ko FC , Karim L , Brooks DJ , et al. Bisphosphonate withdrawal: effects on bone formation and bone resorption in maturing male mice . J Bone Miner Res . 2017 ; 32 ( 4 ): 814 – 820 . Crossref PubMed Google Scholar
19. Dell R , Greene D . A proposal for an atypical femur fracture treatment and prevention clinical practice guideline . Osteoporos Int . 2018 ; 29 ( 6 ): 1277 – 1283 . Crossref PubMed Google Scholar
20. Mühlbauer RC , Bauss F , Schenk R , et al. Bm 21.0955, a potent new bisphosphonate to inhibit bone resorption . J Bone Miner Res . 1991 ; 6 ( 9 ): 1003 – 1011 . Crossref PubMed Google Scholar
21. Bagi CM , DeLeon E , Ammann P , et al. Histo-anatomy of the proximal femur in rats: impact of ovariectomy on bone mass, structure, and stiffness . Anat Rec . 1996 ; 245 ( 4 ): 633 – 644 . Crossref PubMed Google Scholar
22. Jämsä T , Tuukkanen J , Jalovaara P . Femoral neck strength of mouse in two loading configurations: method evaluation and fracture characteristics . J Biomech . 1998 ; 31 ( 8 ): 723 – 729 . Crossref PubMed Google Scholar
23. Jepsen KJ , Silva MJ , Vashishth D , et al. Establishing biomechanical mechanisms in mouse models: practical guidelines for systematically evaluating phenotypic changes in the diaphyses of long bones . J Bone Miner Res . 2015 ; 30 ( 6 ): 951 – 966 . Crossref PubMed Google Scholar
24. Silva MJ , Eekhoff JD , Patel T , et al. Effects of high-fat diet and body mass on bone morphology and mechanical properties in 1100 advanced intercross mice . Journal of Bone and Mineral Research . 2019 ; 34 ( 4 ): 711 – 725 . Crossref PubMed Google Scholar
25. Aref MW , McNerny EMB , Brown D , et al. Zoledronate treatment has different effects in mouse strains with contrasting baseline bone mechanical phenotypes . Osteoporos Int . 2016 ; 27 ( 12 ): 3637 – 3643 . Crossref PubMed Google Scholar
26. Bassett JHD , Gogakos A , White JK , et al. Rapid-throughput skeletal phenotyping of 100 knockout mice identifies 9 new genes that determine bone strength . PLoS Genet . 2012 ; 8 : e1002858 . Crossref PubMed Google Scholar
27. Patel VC , Lazzarini AM . Bilateral simultaneous femoral diaphyseal fractures in a patient with long-term ibandronate use . Orthopedics . 2010 ; 33 ( 10 ): 775 . Crossref PubMed Google Scholar
28. Espey R , Grimes S , Heyburn G , Kealey WD . The first reported case of atypical femoral fracture caused by daily ibandronate prescribed for bone metastases in breast cancer . BMJ Case Rep . 2017 ; 2017 : bcr-2016-217489 . Crossref PubMed Google Scholar
29. Suh Y-S , Jang B-W , Nho J-H , et al. Atypical incomplete femoral neck fracture in patients taking long-term bisphosphonate: case report, a report of 2 cases . Medicine . 2019 ; 98 ( 9 ): e14701 . Crossref PubMed Google Scholar
30. Lloyd AA , Gludovatz B , Riedel C , et al. Atypical fracture with long-term bisphosphonate therapy is associated with altered cortical composition and reduced fracture resistance . Proc Natl Acad Sci U S A . 2017 ; 114 ( 33 ): 8722 – 8727 . Crossref PubMed Google Scholar
31. Langdahl B , Ferrari S , Dempster DW . Bone modeling and remodeling: potential as therapeutic targets for the treatment of osteoporosis . Ther Adv Musculoskelet Dis . 2016 ; 8 ( 6 ): 225 – 235 . Crossref PubMed Google Scholar
32. Bertram JE , Biewener AA . Bone curvature: sacrificing strength for load predictability? J Theor Biol . 1988 ; 131 ( 1 ): 75 – 92 . Crossref PubMed Google Scholar
33. Martelli S , Pivonka P , Ebeling PR . Femoral shaft strains during daily activities: implications for atypical femoral fractures . Clin Biomech . 2014 ; 29 ( 8 ): 869 – 876 . Crossref PubMed Google Scholar
34. Yang H , Butz KD , Duffy D , et al. Characterization of cancellous and cortical bone strain in the in vivo mouse tibial loading model using microCT-based finite element analysis . Bone . 2014 ; 66 : 131 – 139 . Crossref PubMed Google Scholar
35. Song L , Bi Y-N , Zhang P-Y , et al. Optimization of the time window of interest in ovariectomized imprinting control region mice for Antiosteoporosis research . Biomed Res Int . 2017 ; 2017 : 1 – 10 . Crossref PubMed Google Scholar
36. Unger S , Stefan U , Blauth M , et al. Effects of three different preservation methods on the mechanical properties of human and bovine cortical bone . Bone . 2010 ; 47 ( 6 ): 1048 – 1053 . Crossref PubMed Google Scholar
Author contributions
S. Monzem: Designed the study, Contributed to the experimental work, Carried out image and statistical analyses of the data, Contributed to the interpretation of data, Prepared first draft and critically revised the manuscript, Accountable for addessing questions relating to accuracy and integrity of the manuscript, ensuring they were investigated and resolved.
R. Y. Ballester: Designed the study, Contributed to the experimental work and interpretation of data, Critically revised the manuscript, Accountable for addessing questions relating to accuracy and integrity of the manuscript, ensuring they were investigated and resolved.
B. Javaheri: Designed the study, Carried out image and statistical analyses of data, Contributed to interpretation of data, Critically revised the manuscript, Accountable for addessing questions relating to accuracy and integrity of the manuscript, ensuring they were investigated and resolved.
B. Poulet: Contributed to interpretation of data, Critically revised the manuscript, Accountable for addessing questions relating to accuracy and integrity of the manuscript, ensuring they were investigated and resolved.
D. A. Sônego: Contributed to the experimental work, Critically revised the manuscript, Accountable for addessing questions relating to accuracy and integrity of the manuscript, ensuring they were investigated and resolved.
A. A. Pitsillides: Contributed to the interpretation of data, Prepared the first draft, critically revised, and approved the manuscript, Accountable for addessing questions relating to accuracy and integrity of the manuscript, ensuring they were investigated and resolved.
R. L. Souza: Designed the study, Critically revised the manuscript, Accountable for addessing questions relating to accuracy and integrity of the manuscript, ensuring they were investigated and resolved.
R. Y. Ballester and B. Javaheri are joint second author.
Funding statement
No benefits in any form have been received or will be received from a commercial party related directly or indirectly to the subject of this article.
ICMJE COI statement
R. L. Souza reports consulting fee or honorarium from CNPq (National Council for Scientific and Technological Development), which is related to this manuscript.
Acknowledgements
This work was carried out with support of CAPES (coordination of improvement of personnel of superior level, Brazil) - Finance Code 001, Veterinary Hospital of Federal University of Mato Grosso, Faculty of Dentistry of São Paulo University and Royal Veterinary College. We wish to thank Dr Karl Jepsen and Dr Yu-Mei Chang for their valuable contributions.
Ethical review statement
This study agrees with the Ethical Principles for Animal Research, National Council for Control of Animal Experimentation, and was approved by the Institutional Committee for Ethics in Animal Use (Federal University of Mato Grosso-UFMT) number 23.108.188855/2016-26.
© 2020 Author(s) et al. This is an open-access article distributed under the terms of the Creative Commons Attributions licence (CC-BY-NC-ND), which permits unrestricted use, distribution, and reproduction in any medium, but not for commercial gain, provided the original author and source are credited.