Abstract
Objectives
Excessive acetabular coverage is the most common cause of pincer-type femoroacetabular impingement. To date, an association between acetabular over-coverage and genetic variations has not been studied. In this study we investigated the association between single nucleotide polymorphisms (SNPs) of paralogous Homeobox (HOX)9 genes and acetabular coverage in Japanese individuals to identify a possible genetic variation associated with acetabular over-coverage.
Methods
We investigated 19 total SNPs in the four HOX9 paralogs, then focused in detail on seven of those located in the 3’ untranslated region of HOXB9 (rs8844, rs3826541, rs3826540, rs7405887, rs2303485, rs2303486, rs79931349) using a case-control association study. The seven HOXB9 SNPs were genotyped in 316 subjects who had all undergone radiological examination. The association study was performed by both single-locus and haplotype-based analyses.
Results
The genotype and allele frequencies of the five HOXB9 SNPs showed significant association with acetabular over-coverage compared with controls (rs7405887 OR = 3.16, p = 5.29E-6, 95% CI 1.91 to 5.25). A significant difference was also detected when haplotypes were evaluated (OR = 2.59, p = 2.61E-5, 95% CI 1.65 to 4.08). The two HOXB9 SNPs (rs2303485, rs2303486) were associated with decreased acetabular coverage (rs2303485 OR = 0.524, p = 0.0091, 95% CI 0.322 to 0.855; rs2303486 OR = 0.519, p = 0.011, 95% CI 0.312 to 0.865).
Conclusions
The five HOXB9 SNPs (rs8844, rs3826541, rs3826540, rs7405887, rs79931349) were associated with acetabular over-coverage. On the other hand, the two SNPs (rs2303485 and rs2303486) were associated with the lower acetabular coverage. The association of rs2303486 would be consistent with the previous study. Therefore, the HOXB9 SNPs might be involved in the morphogenesis of acetabular coverage, and could be an independent risk factor for developing pincer-type femoroacetabular impingement.
Cite this article: Bone Joint Res 2015;4:50–5.
Article focus
To date, an association between acetabular over-coverage and genetic variations has not been studied. We investigated the association between single nucleotide polymorphisms (SNPs) of paralogous HOX9 genes and acetabular over-coverage.
Key messages
The HOXB9 SNPs might be involved in the morphogenesis of acetabular coverage, and could be an independent risk factor for developing pincer-type femoroacetabular impingement.
Strengths and limitations
Strengths: This is the first report on the association of genetic variations in acetabular over-coverage. The HOXB9 SNPs showed significant association with acetabular over-coverage compared with controls.
Limitations: More studies should be conducted with larger sample numbers and using different ethnic groups.
Introduction
The hip is a ball-and-socket joint. The socket is formed by the acetabulum, which is part of the large bone of the pelvis. The degree of acetabular coverage is correlated with several hip diseases, such as acetabular dysplasia (AD), femoroacetabular impingement (FAI), labral tears, cartilage damage, and hip osteoarthritis (OA).1-3 AD is a major and common cause of secondary hip OA, particularly in women.1 It is characterised by a shallow acetabulum and decreased coverage of the femoral head. This condition appears to be related to a number of different factors.2 Several studies have investigated the association between genetic variations and AD.4-10 On the other hand, the morphogenesis of acetabular over-coverage (AO) remains poorly understood. Excessive acetabular coverage has been reported to be related to FAI (pincer morphological features).3,11,12
FAI is characterised by contact arising from abnormal morphological features of the hip joint, and has been associated with early OA of the hip. Patients with FAI are young, usually in their 20s to 40s. The estimated prevalence of FAI is 10% to 15%.13 The morphological mechanism of FAI is well established.3 However, the genetic predisposition in the pathogenesis of FAI remains unknown. Two main types of FAI have been described in relation to morphological variations of the acetabulum and femoral head, namely: pincer-type and cam-type impingement. Pincer-type impingement occurs on the excessive acetabular coverage, and is more common in women.11 AO is correlated with the radiologic depth of the acetabular fossa. To date, an association between AO and genetic variations has not been studied. Considering the involvement of genetic variations in several examples of AD studies, it is worth investigating whether any genetic variation is associated with susceptibility to AO.
Homeobox (HOX) genes encode a family of transcription factors of fundamental importance for body patterning along the anteroposterior (AP) axis during skeletal development.14,15 Particularly, paralogous Hox9 genes were shown to have an essential role in the development of the limb buds.16-19 In the present study, to identify a possible genetic variation associated with AO, we investigated the association between single nucleotide polymorphisms (SNPs) of paralogous HOX9 genesand AO.
Patients and Methods
Study subjects and radiographs
The study examined 316 unrelated Japanese subjects who came to our hospital and underwent radiological examination. To exclude some diseases, all subjects completed a standard questionnaire regarding their medical history. All cases were sporadic in nature. Some individuals were under medical treatment and/or had received operative therapy. The evaluation of acetabular coverage was based on radiological findings. Radiographs were taken with a tube-to-film distance of 100 cm. The pelvises were positioned supine, with their frontal anatomical plane parallel to the film plate. The central beam was directed to the midpoint between the pubic symphysis and a horizontal line connecting both anterior and superior iliac spines. All the radiographs fulfilled the criteria for correct pelvic positioning with regard to both the axial and the transverse pelvic rotation.3 The angle formed by a line from the inferior pelvic teardrop to the superior lateral edge of the acetabulum, and a line horizontal from the inferior pelvic teardrop constituted the Sharp angle.20 In this study, AO was defined as a Sharp angle < 42° on at least one side. Two authors (TS and EC) independently measured the Sharp angle twice.
The mean age of the 130 subjects (125 females and five males) with AO was 29.3 years (sd 10.5; 20 to 88). The mean Sharp angle on the AO side was 38.9° (sd 2.1; 32.2° to 41.9°). The mean age of the 186 control subjects (with a Sharp angle ≥ 42° on both sides; 176 females and ten males) was 42.1 years (sd 16.1; 17 to 72). The mean Sharp angle on the side with more coverage was 46.4° (sd 3.4; 42.1º to 60.0°) (Table I). DNA was extracted from peripheral blood leukocytes. All participants gave written informed consent for the genetic analysis. The Medical Ethics Committee of the University of Miyazaki approved the study.
Table I
Characteristics of AO cases and controls
Group | Subjects (n) | Age (yrs) | Gender (%, female) | Sharp angle20 | AR (%) |
---|---|---|---|---|---|
AO | 130 | 29.3 (sd 10.5) | 96.2 | 38.9° (sd 2.1)* | 32.3† |
Control | 186 | 42.1 (sd 16.1) | 94.6 | 46.4° (sd 3.4) | 6.5 |
-
* p < 0.001versus controls, Mann–Whitney U test † Odds ratio = 6.92, p = 1.87E-9, 95% CI 3.47 to 13.8, chi-squared test AO, acetabular overcoverage; AR, acetabular retroversion; sd, standard deviation
Genotyping
Following extraction of genomic DNA, genotyping of the polymorphisms was performed by PCR amplification of 19 total SNPs in four HOX9 paralogs (HOXA9, HOXB9, HOXC9, HOXD9) using forward and reverse primers 1, 2 and 3. PCR products were directly sequenced using an ABI Prism 3130 sequencer (Applied Biosystems, Foster City, California). Genotype and allele frequencies of the SNPs were determined by direct counting. The pair-wise linkage disequilibrium (LD) patterns and block partition of the SNPs identified in the 3’ untranslated region (UTR) of HOXB9 gene were analysed using Haploview version 4.2.21 Japanese HapMap genotyping data of the HOX9 SNPs were retrieved from the International HapMap Project22,23 website by querying the HapMap Data Release #28. In this study, we selected seven SNPs in the 3’ UTR of HOXB9. This is because in previous studies, Rouault et al24 and Hao et al10 selected two SNPs in the 3’UTR of HOXB9 (rs8844, rs2303486), which were included in these seven SNPs, to investigate an association with congenital dislocation of the hip (CDH) using French and Chinese-based populations, respectively, and in the HapMap Data Release #28, the Japanese genotype and allele frequency data of HOXB9 SNPs were shown mainly in the 3’ UTR region (rs8844, rs2303486 and rs4239158).
Statistical analysis
All numerical values are expressed as the mean with standard deviation. The difference in the Sharp angle between cases and controls was analysed using a Mann–Whitney U test. The frequency of acetabular retroversion (AR) was assessed by the chi-squared test. The Hardy–Weinberg equilibrium for each group and the comparisons of genotype and allele frequencies between groups were performed using the chi-squared test with SNPAlyze (ver.7 DYNACOM Co., Ltd., Chiba, Japan). Values of p < 0.05 were considered statistically significant. A statistical analysis for power was performed using G*Power3 version 3.1.925-28under the following conditions: Power (1-beta); 80%, Test family; chi-squared tests, Effect size; 0.158, Type I error rate; 0.05, Df; 1.
Corrected p-values were used, derived from the Bonferroni correction for multiple comparisons, where appropriate.
Results
In 316 subjects, 130 were AO-positive cases and 186 were controls. As shown in Table I, 32.3% of AO cases had acetabular retroversion (AR OR = 6.92, p = 1.87E-9, 95% CI 3.47 to 13.8). We performed genotyping of 19 SNPs in the four HOX9 paralogs (Table II) by PCR amplification using forward and reverse primers. We found a novel SNP located in the 3’ UTR of HOXB9, c1486G> A (rs79931349). In this study, we focused on seven specific SNPs located in the 3’ UTR of HOXB9 that were significantly associated with AO (Tables III and IV). The minor allele frequencies for these SNPs were all > 0.05. The statistical power was 80% at the effect size 0.158.25-28 The remaining 12 SNPs in the HOX9 paralogs did not show a significant association with AO (data not shown).
Table II
Polymorphisms of the paralogous HOX9 genes investigated in this study
Gene | dbSNP name | Region | Alleles | Position | Primer | Japanese HapMap* | |
---|---|---|---|---|---|---|---|
Allele | MAF | ||||||
HOXA9 | rs3801776 | promoter | G/A | 26500955 | for 1/ rev 1 | A | 0.257 |
rs3839805 | promoter | A/C | 26501106 | for 1/ rev 1 | |||
rs886340 | promoter | A/G | 26503174 | for 2/ rev 2 | G | 0.140 | |
rs2237336 | promoter | T/C | 26503691 | for 2/ rev 2 | |||
rs11978680 | exon1 | C/A | 26500538 | for 3/ rev 3 | |||
rs11975265 | exon1 | A/C | 26500666 | for 3/ rev 3 | |||
HOXB9 | rs8844 | 3’ UTR | C/T | 5351988 | for 1/ rev 1 | T | 0.155 |
rs3826541 | 3’ UTR | C/A | 5352234 | for 1/ rev 1 | |||
rs3826540 | 3’ UTR | T/C | 5352296 | for 1/ rev 1 | |||
rs7405887 | 3’ UTR | G/C | 5352794 | for 2/ rev 2 | |||
rs2303485 | 3’ UTR | G/A | 5352841 | for 2/ rev 2 | |||
rs2303486 | 3’ UTR | A/T | 5352947 | for 2/ rev 2 | T | 0.409 | |
rs79931349 | 3’ UTR | G/A | 5353011 | for 2/ rev 2 | |||
HOXC9 | rs11829948 | exon1 | T/C | 16537643 | for 1/ rev 1 | ||
rs7137528 | exon1 | G/C | 16537773 | for 1/ rev 1 | |||
rs2241820 | exon1 | T/C | 16537803 | for 1/ rev 1 | C | 0.252 | |
HOXD9 | rs3731794 | promoter | C/A | 27195846 | for 1/ rev 1 | ||
rs711821 | exon1 | A/C | 27197432 | for 2/ rev 2 | |||
rs10665265 | exon1 | C/A | 27197710 | for 2/ rev 2 | |||
Table III
Genotype and allele frequencies of the HOXB9 SNPs in the 130 AO cases and 186 controls
dbSNP | Genotypes (%) | Alleles (%) | MAF | HWE exact | |||||||
---|---|---|---|---|---|---|---|---|---|---|---|
Groups | p-value | ||||||||||
rs8844 | CC | CT | TT | C | T | ||||||
AO | 76 (58.5) | 49 (37.7) | 5 (3.8) | 201 (77.3) | 59 (22.7) | ||||||
Control | 151 (81.2) | 33 (17.7) | 2 (1.1) | 335 (90.1) | 37 (9.9) | 0.152 | 0.696 | ||||
rs3826541 | CC | CA | AA | C | A | ||||||
AO | 76 (58.5) | 49 (37.7) | 5 (3.8) | 201 (77.3) | 59 (22.7) | ||||||
Control | 151 (81.2) | 33 (17.7) | 2 (1.1) | 335 (90.1) | 37 (9.9) | 0.152 | 0.696 | ||||
rs3826540 | TT | TC | CC | T | C | ||||||
AO | 77 (59.2) | 48 (36.9) | 5 (3.8) | 202 (77.7) | 58 (22.3) | ||||||
Control | 151 (81.2) | 33 (17.7) | 2 (1.1) | 335 (90.1) | 37 (9.9) | 0.150 | 0.696 | ||||
rs7405887 | CC | CG | GG | C | G | ||||||
AO | 75 (57.7) | 50 (38.5) | 5 (3.8) | 200 (76.9) | 60 (23.1) | ||||||
Control | 151 (81.2) | 32 (17.2) | 3 (1.6) | 334 (89.8) | 38 (10.2) | 0.155 | 0.411 | ||||
rs2303485 | GG | GA | AA | G | A | ||||||
AO | 96 (73.8) | 29 (22.3) | 5 (3.8) | 221 (85.0) | 39 (15.0) | ||||||
Control | 111 (59.7) | 67 (36.0) | 8 (4.3) | 289 (77.7) | 83 (22.3) | 0.193 | 0.677 | ||||
rs2303486 | AA | AT | TT | A | T | ||||||
AO | 43 (33.1) | 56 (43.1) | 31 (23.8) | 142 (54.6) | 118 (45.4) | ||||||
Control | 38 (20.4) | 101 (54.3) | 47 (25.3) | 177 (47.6) | 195 (52.4) | 0.495 | 0.303 | ||||
rs79931349 | GG | GA | AA | G | A | ||||||
AO | 76 (58.5) | 50 (38.5) | 4 (3.1) | 202 (77.7) | 58 (22.3) | ||||||
Control | 151 (81.2) | 33 (17.7) | 2 (1.1) | 335 (90.1) | 37 (9.9) | 0.150 | 0.696 | ||||
-
AO, acetabular overcoverage; MAF, minor allele frequency; HWE, Hardy–Weinberg equilibrium
Table IV
Association of the HOXB9 SNPs and haplotypes in the AO cases and controls
Genotypes | Alleles | ||||||||
---|---|---|---|---|---|---|---|---|---|
dbSNP | OR | p-value | 95% CI | OR | p-value | 95% CI | |||
rs8844 | CC vs CT+TT | 3.07 | 9.93E-6 | 1.85 to 5.09 | T vs C | 2.66 | 1.12E-5 | 1.70 to 4.15 | |
rs3826541 | CC vs CA+AA | 3.07 | 9.93E-6 | 1.85 to 5.09 | A vs C | 2.66 | 1.12E-5 | 1.70 to 4.15 | |
rs3826540 | TT vs TC+CC | 2.97 | 1.84E-5 | 1.79 to 4.93 | C vs T | 2.60 | 1.88E-5 | 1.66 to 4.07 | |
rs7405887 | CC vs CG+GG | 3.16 | 5.29E-6 | 1.91 to 5.25 | G vs C | 2.64 | 1.10E-5 | 1.69 to 4.11 | |
rs2303485 | GG vs GA+AA | 0.524 | 0.00912 | 0.322 to 0.855 | A vs G | 0.614 | 0.0219 | 0.404 to 0.934 | |
rs2303486 | AA vs AT+TT | 0.519 | 0.01128 | 0.312 to 0.865 | T vs A | 0.754 | 0.0818 | 0.549 to 1.036 | |
rs79931349 | GG vs GA+AA | 3.07 | 9.93E-6 | 1.85 to 5.09 | A vs G | 2.60 | 1.88E-5 | 1.66 to 4.07 | |
Haplotypes | AO (%) | Control (%) | OR | p-value | 95% CI | ||||
C-C-T-C-○-●-G | 200 (76.9) | 333 (89.5) | |||||||
T-A-C-G-○-●-A | 56 (20.0) | 33 (9.7) | 2.59 | 2.61E-5 | 1.65 to 4.08 | ||||
-
SNP, single nucleotide polymorphism; AO, acetabular overcoverage; OR, odds ratio for recessive model; CI, confidence interval; ○,G/A; ●,A/T. The remaining five haplotypes (C-C-T-G-○-●-A, C-C-T-G-○-●-G, T-A-C-G-○-●-G, T-A-C-C-○-●-A, T-A-T-G-○-●-A) showed frequencies less than 0.05
In the single-locus association analysis, distributions of genotypes in AO cases and controls were all in accordance with the Hardy–Weinberg equilibrium (Table III). The genotype and allele frequencies of the five HOXB9 SNPs (rs8844, rs3826541, rs3826540, rs7405887, rs79931349) showed statistically significant differences between AO cases and controls (rs7405887 OR = 3.16, p = 5.29E-6, 95% CI 1.91 to 5.25). On the other hand, the two SNPs (rs2303485, rs2303486) were associated with the decreased acetabular coverage (rs2303485 OR = 0.524, p = 0.0091, 95% CI 0.322 to 0.855; rs2303486 OR = 0.519, p = 0.011, 95% CI 0.312 to 0.865) (Table IV). Subsequently, we carried out a haplotype-based association study using Haploview,21 which was used to visualise the structure of pair-wise LD between the SNPs, and to define haplotype blocks. A total of five SNP pairs showed high LD values, and constituted a single haplotype block in the 3’ UTR of HOXB9 (Fig. 1). Results of the haplotype analysis for HOXB9 are presented in Table IV. A total of seven possible haplotypes were obtained, five of which had a frequency < 5%; therefore, we analysed the two most prevalent haplotypes (C-C-T-C-G/A-A/T-G and T-A-C-G-G/A-A/T-A). The haplotype with minor alleles of five SNPs (T-A-C-G-G/A-A/T-A) showed a significant association with AO (OR = 2.59, p = 2.61E-5, 95% CI 1.65 to 4.08).
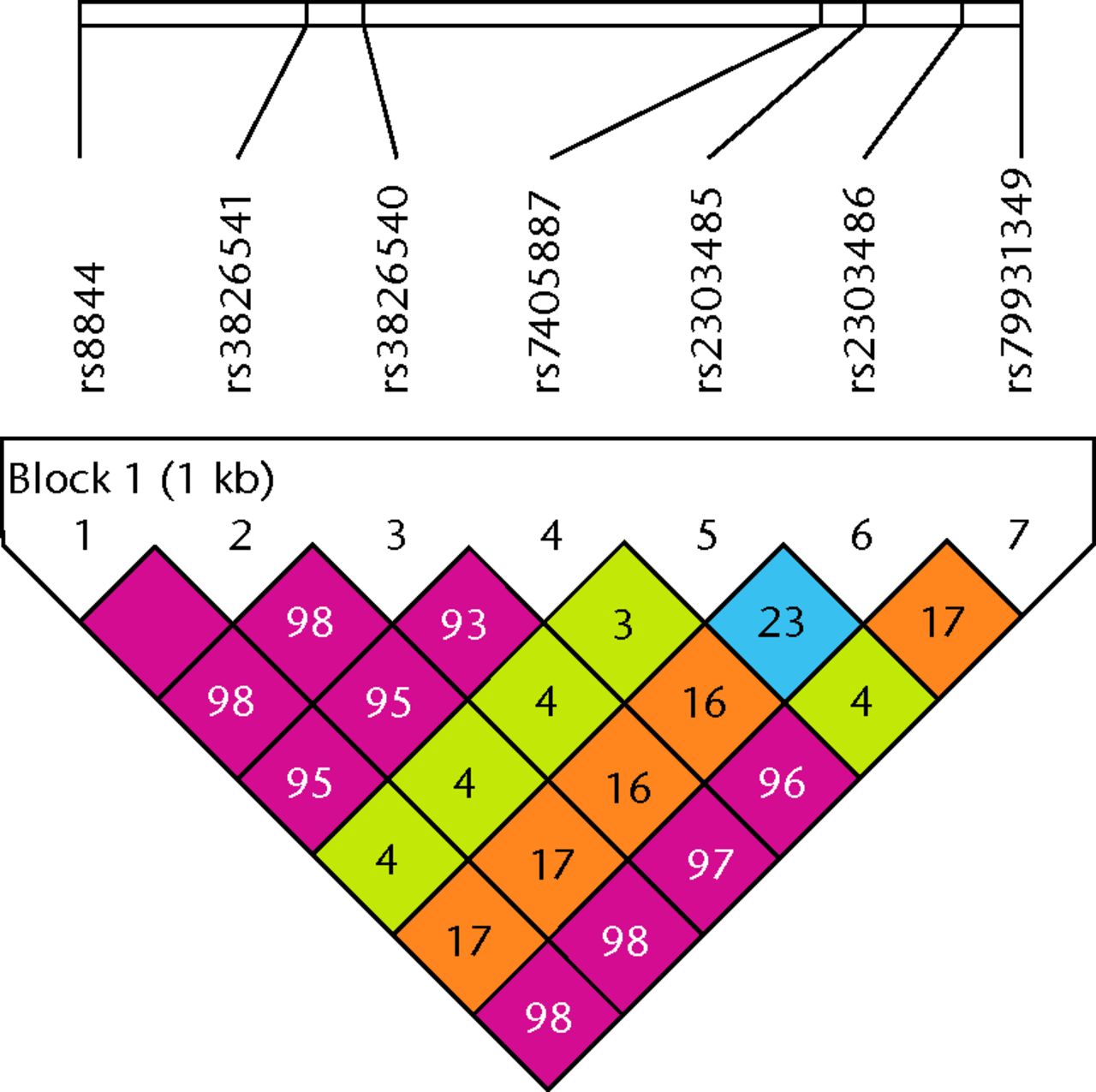
Fig. 1
LD plot of seven single nucleotide polymorphisms (SNPs) in the 3’ UTR of HOXB9. r2values that correspond to SNP pairs are expressed as percentages and shown within the respective squares. Higher r2 values are indicated in red. These five SNPs constitute a haplotype block that spans the 3’ UTR of the HOXB9 gene.
Discussion
Excessive acetabular coverage is thought to be the major cause of pincer-type FAI.3,11,12 While AD has been reported to be associated with several genetic variants,4-10 the gene variants of AO remain unclear. Nevertheless, prior to our study, no other studies have investigated the association between genetic variations and AO. To the best of our knowledge, this is the first report on the association of genetic variations of HOX9 in AO.
The mammalian Hox complex contains 39 genes organised into four different clusters, Hox A, B, C, and D. Based on DNA sequence and the position of the genes on their respective chromosomes, individual members of the four clusters have been classified into 13 paralogous families. Members of a paralogous family often share similar gene expression patterns. The chromosomal order of the HOX genes is collinear to the relative position of their expression domains along the AP axis of the developing embryo, such that the more 3’ the gene is located, the more anterior its expression boundary.14,15,29 Using gene targeting, abdominal B-related genes, which are paralogous to Hox9-13 genes, were shown to have an essential role in the development of the limb buds.16,30,31 In humans, Muragaki et al32 demonstrated that synpolydactyly, an inherited abnormality of the hands and feet, is caused by expansion of a polyalanine stretch in the amino–terminal region of HOXD13. Mortlock and Innis33 reported the identification of a HOXA13 nonsense mutation in a family with hand–foot–genital syndrome. Paralogous HOX9 genes are located in the most 3’ regions of the abdominal B-related gene regions in HOX complexes. Moreover, HOX9 genes are expressed specifically in the anterior portion of the developing limb buds.17 Therefore, HOX9 paralogsmight be involved in the development of the hip region. In 2009, Rouault et al24 investigated the association of the CDH with two HOXB9 SNPs (rs8844 and rs2303486) in a sample population from France. The case-control study revealed no significant association between CDH and HOXB9 SNPs.24 On the other hand, quite recently, Hao et al10 reported that rs2303486 was associated with CDH in a Chinese population. However, these studies did not evaluate a potential associated with AO. Human HOXB9 is located on the chromosome 17q21.3. HOXB9 cDNA is 2711 bp long with a 180 bp sequence element called the homeobox in exon2. HOXB9 encodes a 250 amino acid protein (NCBI Gene ID: 3219). Based upon genetic predisposition and the possible important function of HOX genes in the pathogenesis of skeletal disorders, it appears worthwhile to investigate whether HOXB9 gene polymorphisms are associated with susceptibility to AO.
In this case-control study, we identified the five SNPs (rs8844, rs3826541, rs3826540, rs7405887, rs79931349) located in the 3’ UTR of the HOXB9 gene that are associated with AO (rs 7405887 OR = 3.16, p = 5.29E-6, 95% CI 1.91 to 5.25; Table IV), and may be an independent risk factor for future development of AO. Interestingly, the two HOXB9 SNPs indicated OR < 1 respectively (rs2303485 OR = 0.524, p = 0.0091, 95% CI 0.322 to 0.855; rs2303486 OR = 0.519, p = 0.011, 95% CI 0.312 to 0.865) (Table IV). These two SNPs could be associated with decreased acetabular coverage. Lower acetabular coverage is a major and common cause of CDH, which is a contrasting disease to pincer-type FAI. The association of rs2303486 would be consistent with Hao et al’s report.10 Therefore, HOXB9 might be relevant to acetabular morphogenesis. However, the morphological mechanism of HOXB9 is not obvious, although HOX9 paralogs are critical during organogenesis, limb development and growth of tumour.18,19,34,35 Further studies are needed to clarify the mechanism in detail that links the HOXB9 SNPs with the acetabular morphology. As shown in Table I, 32.3% of AO cases had AR (OR = 6.92, p = 1.87E-9, 95% CI 3.47 to 13.8). AR appears to be related to AO. AR is defined as a ‘crossover’ sign,36,37 and is also thought to be the common cause of pincer-type FAI.3,11,12 The HOXB9 SNPs may be associated with the pathogenesis of AR in the same way as AO.
This study had several limitations, such as a small sample size and a lack of replication study. Therefore, more studies should be conducted with larger sample numbers and using different ethnic groups. These future studies on HOXB9 function and its relevance to AO will contribute not only to the elucidation of the pathogenic mechanism of pincer-type FAI, but also to the identification of a novel therapeutic target for the disease. The HOXB9 SNPs could be a predictive tool to identify AO, helping to delay or prevent the onset of pincer-type FAI.
1 Lehmann HP , HintonR, MorelloP, SantoliJ. Developmental dysplasia of the hip practice guideline: technical report. Committee on Quality Improvement, and Subcommittee on Developmental Dysplasia of the Hip. Pediatrics2000;105:57.CrossrefPubMed Google Scholar
2 Ziegler J , ThielemannF, Mayer-AthenstaedtC, GüntherKP. The natural history of developmental dysplasia of the hip. A meta-analysis of the published literature. Orthopade2008;37:515-516, 518-524. (In German). Google Scholar
3 Ganz R , ParviziJ, BeckM, et al.Femoroacetabular impingement: a cause for osteoarthritis of the hip. Clin Orthop Relat Res2003;417:112–120.CrossrefPubMed Google Scholar
4 Kizawa H , KouI, IidaA, et al.An aspartic acid repeat polymorphism in asporin inhibits chondrogenesis and increases susceptibility to osteoarthritis. Nat Genet2005;37:138–144.CrossrefPubMed Google Scholar
5 Iida A , KizawaH, NakamuraY, IkegawaS. High-resolution SNP map of ASPN, a susceptibility gene for osteoarthritis. J Hum Genet2006;51:151–154.CrossrefPubMed Google Scholar
6 Mabuchi A , NakamuraS, TakatoriY, IkegawaS. Familial osteoarthritis of the hip joint associated with acetabular dysplasia maps to chromosome 13q. Am J Hum Genet2006;79:163–168.CrossrefPubMed Google Scholar
7 Miyamoto Y , MabuchiA, ShiD, et al.A functional polymorphism in the 5′ UTR of GDF5 is associated with susceptibility to osteoarthritis. Nat Genet2007;39:529–533. Google Scholar
8 Dai J , ShiD, ZhuP, et al.Association of a single nucleotide polymorphism in growth differentiate factor 5 with congenital dysplasia of the hip: a case-control study. Arthritis Res Ther2008;10:R126.CrossrefPubMed Google Scholar
9 Sekimoto T , IshiiM, EmiM, et al.Segmental copy number loss in the region of Semaphorin 4D gene in patients with acetabular dysplasia. J Orthop Res2013;31:957–961.CrossrefPubMed Google Scholar
10 Hao Z , DaiJ, ShiD, et al.Association of a single nucleotide polymorphism in HOXB9 with developmental dysplasia of the hip: a case-control study. J Orthop Res2014;32:179–182.CrossrefPubMed Google Scholar
11 Tannast M , SiebenrockKA, AndersonSE. Femoroacetabular impingement: radiographic diagnosis--what the radiologist should know. AJR Am J Roentgenol2007;188:1540–1552.CrossrefPubMed Google Scholar
12 Anderson SE , SiebenrockKA, TannastM. Femoroacetabular impingement: evidence of an established hip abnormality. Radiology2010;257:8–13.CrossrefPubMed Google Scholar
13 Leunig M , GanzR. Femoroacetabular impingement: a common cause of hip complaints leading to arthrosis. Unfallchirurg2005;108:9-10, 12-17. (In German). Google Scholar
14 Krumlauf R . Hox genes in vertebrate development. Cell1994;78:191–201.CrossrefPubMed Google Scholar
15 McGinnis W , KrumlaufR. Homeobox genes and axial patterning. Cell1992;68:283–302.CrossrefPubMed Google Scholar
16 Fromental-Ramain C , WarotX, LakkarajuS, et al.Specific and redundant functions of the paralogous Hoxa-9 and Hoxd-9 genes in forelimb and axial skeleton patterning. Development1996;122:461–472.CrossrefPubMed Google Scholar
17 Nelson CE , MorganBA, BurkeAC, et al.Analysis of Hox gene expression in the chick limb bud. Development1996;122:1449–1466.CrossrefPubMed Google Scholar
18 Zakany J , DubouleD. The role of Hox genes during vertebrate limb development. Curr Opin Genet Dev2007;17:359–366.CrossrefPubMed Google Scholar
19 Xu B , WellikDM. Axial Hox9 activity establishes the posterior field in the developing forelimb. Proc Natl Acad Sci USA2011;108:4888–4891.CrossrefPubMed Google Scholar
20 Monazzam S , AgasheM, HosalkarHS. Reliability of overcoverage parameters with varying morphologic pincer features: comparison of EOS® and radiography. Clin Orthop Relat Res2013;471:2578–2585.CrossrefPubMed Google Scholar
21 Barrett JC , FryB, MallerJ, DalyMJ. Haploview: analysis and visualization of LD and haplotype maps. Bioinformatics2005;21:263–265.CrossrefPubMed Google Scholar
22 International HapMap Consortium. The International HapMap Project Nature2003;42:789–796. Google Scholar
23 International HapMap Consortium. Integrating ethics and science in the International HapMap Project Nat Rev Genet2004;5:467–475. Google Scholar
24 Rouault K , ScotetV, AutretS, et al.Do HOXB9 and COL1A1 genes play a role in congenital dislocation of the hip? Study in a Caucasian population. Osteoarthritis Cartilage2009;17:1099–1105.CrossrefPubMed Google Scholar
25 Cohen J. Statistical power analysis for the behavioral sciences. Second ed. Hillsdale, NJ: Lawrence Erlbaum; 1988. Google Scholar
26 Cohen J . A power primer. Psychol Bull1992;112:155–159.CrossrefPubMed Google Scholar
27 Faul F , ErdfelderE, LangAG, BuchnerA. G*Power 3: a flexible statistical power analysis program for the social, behavioral, and biomedical sciences. Behav Res Methods2007;39:175–191.CrossrefPubMed Google Scholar
28 Faul F , ErdfelderE, BuchnerA, LangAG. Statistical power analyses using G*Power 3.1: tests for correlation and regression analyses. Behav Res Methods2009;41:1149–1160.CrossrefPubMed Google Scholar
29 Sekimoto T , YoshinobuK, YoshidaM, et al.Region-specific expression of murine Hox genes implies the Hox code-mediated patterning of the digestive tract. Genes Cells1998;3:51–64.CrossrefPubMed Google Scholar
30 Davis AP , WitteDP, Hsieh-LiHM, PotterSS, CapecchiMR. Absence of radius and ulna in mice lacking hoxa-11 and hoxd-11. Nature1995;375:791–795.CrossrefPubMed Google Scholar
31 Fromental-Ramain C , WarotX, MessadecqN, et al.Hoxa-13 and Hoxd-13 play a crucial role in the patterning of the limb autopod. Development1996;122:2997–3011.CrossrefPubMed Google Scholar
32 Muragaki Y , MundlosS, UptonJ, OlsenBR. Altered growth and branching patterns in synpolydactyly caused by mutations in HOXD13. Science1996;272:548–551.CrossrefPubMed Google Scholar
33 Mortlock DP , InnisJW. Mutation ofHOXA13 in hand-foot-genital syndrome. Nat Genet1997;15:179–180. Google Scholar
34 Chen F , CapecchiMR. Targeted mutations in hoxa-9 and hoxb-9 reveal synergistic interactions. Dev Biol1997;181:186–196.CrossrefPubMed Google Scholar
35 Shrestha B , AnsariKI, BhanA, et al.Homeodomain-containing protein HOXB9 regulates expression of growth and angiogenic factors, facilitates tumor growth in vitro and is overexpressed in breast cancer tissue. FEBS J2012;279:3715–3726. Google Scholar
36 Reynolds D , LucasJ, KlaueK. Retroversion of the acetabulum. A cause of hip pain. J Bone Joint Surg [Br]1999;81-B:281–288.CrossrefPubMed Google Scholar
37 Jamali AA , MladenovK, MeyerDC, et al.Anteroposterior pelvic radiographs to assess acetabular retroversion: high validity of the “cross-over-sign”. J Orthop Res2007;25:758–765. Google Scholar
Funding statement:
This study was supported by a Grant-in-Aid from the Hip Joint Foundation of Japan. We thank W. Souma, I. Tsuchimochi, Y. Ozawa and A. Saito for their technical support in the laboratory.
Author contributions:
T. Sekimoto: Study design, Data collection and analysis, Writing the paper
S. Kurogi: Data collection and analysis, Editorial contribution
T. Funamoto: Data collection and analysis, Editorial contribution
T. Ota: Data collection and analysis, Editorial contribution
S. Watanabe: Data collection and analysis, Editorial contribution
T. Sakamoto: Data collection and analysis, Editorial contribution
H. Hamada: Data collection and analysis, Editorial contribution
E. Chosa: Study design, Editorial contribution
ICMJE Conflict of Interest:
None declared
©2015 The British Editorial Society of Bone & Joint Surgery. This is an open-access article distributed under the terms of the Creative Commons Attributions licence, which permits unrestricted use, distribution, and reproduction in any medium, but not for commercial gain, provided the original author and source are credited.
Supplementary material. A table showing the primers used in this study, as well as a figure of direct sequences of genomic DNA from Japanese individuals, showing the five HOXB9 SNPs (red boxes), are available alongside the online version of this article at www.bjr.boneandjoint.org.uk