Abstract
Objectives
Ligaments which heal spontaneously have a healing process that is similar to skin wound healing. Menopause impairs skin wound healing and may likewise impair ligament healing. Our purpose in this study was to investigate the effect of surgical menopause on ligament healing in a rabbit medial collateral ligament model.
Methods
Surgical menopause was induced with ovariohysterectomy surgery in adult female rabbits. Ligament injury was created by making a surgical gap in the midsubstance of the medial collateral ligament. Ligaments were allowed to heal for six or 14 weeks in the presence or absence of oestrogen before being compared with uninjured ligaments. Molecular assessment examined the messenger ribonucleic acid levels for collagens, proteoglycans, proteinases, hormone receptors, growth factors and inflammatory mediators. Mechanical assessments examined ligament laxity, total creep strain and failure stress.
Results
Surgical menopause in normal medial collateral ligaments initiated molecular changes in all the categories evaluated. In early healing medial collateral ligaments, surgical menopause resulted in downregulation of specific collagens, proteinases and inflammatory mediators at 6 weeks of healing, and proteoglycans, growth factors and hormone receptors at 14 weeks of healing. Surgical menopause did not produce mechanical changes in normal or early healing medial collateral ligaments. With or without surgical menopause, healing ligaments exhibited increased total creep strain and decreased failure stress compared with uninjured ligaments.
Conclusions
Surgical menopause did not affect the mechanical properties of normal or early healing medial collateral ligaments in a rabbit model. The results in this preclinical model suggest that menopause may result in no further impairment to the ligament healing process.
Cite this article: Bone Joint Res 2015;4:38–44
Article focus
The purpose of this study was to investigate the effect of surgical menopause on ligament healing in a rabbit medial collateral ligament model.
We hypothesised that surgical menopause would impair ligament healing and result in inferior mechanical properties.
Key messages
Surgical menopause did not affect the mechanical properties of normal or early healing medial collateral ligaments in a rabbit model.
The results in this preclinical model suggest that menopause may result in no further impairment to the ligament healing process.
Strengths and limitations
Established surgical, molecular and mechanical methods were used.
A preclinical model was used to evaluate ligament healing at different early healing intervals after surgical medial collateral ligament gap injury with or without surgical menopause.
Introduction
Some ligaments, such as the medial collateral ligament (MCL), heal spontaneously with a process that has three overlapping stages comprising bleeding and inflammation, cell proliferation with matrix production, and matrix remodeling.1 Ligament healing involves similar cellular and matrix processes to those for skin wound healing.1,2 Menopause impairs skin wound healing.3,4 Post-menopausal human female patients have a delayed rate of skin wound healing.3,4 In rodent models, oestrogen deficiency from surgical menopause induced by ovariectomy leads to impaired skin wound healing with delayed re-epithelialisation, increased wound size and decreased collagen deposition.3,4
Oestrogen receptors are present in ligaments, like the MCL and anterior cruciate ligament (ACL),5-7 in addition to being present in other knee connective tissues, such as the menisci,8 cartilage,9 and synovium.8 Furthermore, in vitro studies have indicated that oestrogen receptors are capable of influencing matrix metalloproteinase expression and oestrogen can modulate such expression.10 Surgical menopause induced by ovariectomy in a rabbit model resulted in reductions in serum oestrogen levels.11-14
Given the detrimental effect of oestrogen deficiency on skin wound healing, the similarities in the healing processes for skin and ligaments like the MCL, and the presence of oestrogen receptors in ligaments like the MCL and ACL, our purpose was to investigate the effect of surgical menopause on ligament healing in a rabbit MCL model. First, we examined the effect of surgical menopause on the molecular and mechanical properties of normal and healing ligaments. Second, we examined the effect of ligament healing with and without surgical menopause on the molecular and mechanical properties of the ligament. We hypothesised that surgical menopause would impair ligament healing and result in inferior mechanical properties.
Materials and Methods
A total of 57 female one-year-old (sexually and skeletally mature) New Zealand White rabbits were used in this study approved by the University of Calgary Animal Care Committee, and all experiments were conducted in compliance with Animal Care Committee approval. Molecular and mechanical assessments were performed on six groups with different combinations of ovariohysterectomy (OVH) surgery (No OVH, OVH) and/or bilateral MCL gap surgery (No scar, 6 week scar, 14 week scar) (Fig. 1) New Zealand White rabbits were used in this study approved by the University of Calgary Animal Care Committee, and all experiments were conducted in compliance with Animal Care Committee approval. Molecular and mechanical assessments were performed on six groups with different combinations of ovariohysterectomy (OVH) surgery (No OVH, OVH) and/or bilateral MCL gap surgery (No scar, 6 week scar, 14 week scar) (Fig. 1) surgery (No OVH, OVH) and/or bilateral MCL gap surgery (No scar, 6 week scar, 14 week scar) (Fig. 1) and/or bilateral MCL gap surgery (No scar, 6 week scar, 14 week scar) (Fig. 1) (Fig. 1): (1) No OVH–No scar, (2) No OVH–6 week scar, (3) No OVH–14 week scar; (4) OVH–No scar, (5) OVH–6 week scar, (6) OVH–14 week scar. For the OVH surgery, surgical menopause was induced by the removal of the ovaries and uterus.8,15 A minimum of eight weeks after the OVH surgery, the rabbits underwent the bilateral MCL gap surgery or underwent molecular and mechanical assessments (minimum eight weeks, maximum 15 weeks). For the bilateral MCL gap surgery, a small segment (2.0 mm ± 0.5 mm) of the MCL midsubstance from each hind limb was removed which resulted in a 4.0 mm ± 0.5 mm gap due to retraction of the ligament ends.16,17 The six week and 14 week healing intervals were assessed because these early healing intervals generally relate to the cell proliferation with matrix production and matrix remodeling stages, respectively.1
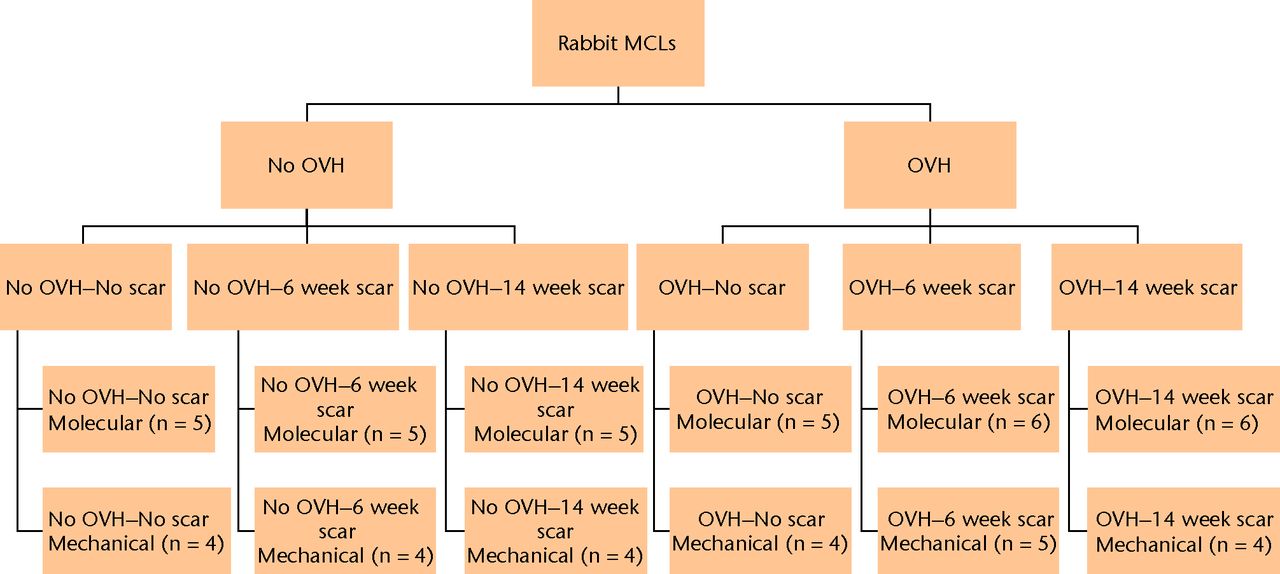
Fig. 1
Study flow chart. Molecular and mechanical assessments were performed on six groups with different combinations of ovariohysterectomy (OVH) surgery (No OVH, OVH) and/or bilateral medial collateral ligament (MCL) gap surgery (No scar, 6 week scar, 14 week scar).
Molecular
A total of 32 rabbits were assigned to molecular assessment (Fig. 1) in groups comprising (1) No OVH–No scar (n = 5), (2) No OVH–6 week scar (n = 5), (3) No OVH–14 week scar (n = 5), (4) OVH–No scar (n = 5), (5) OVH–6 week scar (n = 6), (6) OVH–14 week scar (n = 6). Baseline molecular data for No OVH–No scar and OVH–No scar MCLs were described in Hart and Achari.8
Immediately after the rabbits were killed, the MCL was removed and immediately frozen until processed.8 The TRIspin method was used to isolate total ribonucleic acid (RNA)18 which was quantified using SYBR Green reagent (Molecular Probes, Eugene, Oregon). Total RNA (1 μg) from each ligament was initially reverse transcribed with random primers and then assessed by polymerase chain reaction (PCR) using rabbit-specific primers.15,19,20 Aliquots of RNA were all reverse transcribed at the same time and later underwent PCR at the same time to minimise variation. Following amplicon separation on 2% agarose gel with ethidium bromide staining, Quantity 1 1-D analysis software (BioRad, Hercules, California) determined band density. The levels of the molecules of interest were normalised to the levels of the β-actin housekeeping gene. A second aliquot of RNA was analysed, and the results obtained were nearly identical to the results presented. As reported previously,15,21 real-time PCR analysis and results obtained as detailed above are comparable for a subset of the molecules assessed.
The 21 molecules of interest included matrix molecules where the collagens were collagen I, collagen III and collagen V, and the proteoglycans were biglycan and decorin.15 The proteinases were matrix metalloproteinases (MMP1, MMP3, MMP13)19,20 and cathepsin K proteinase (CathK).15 The hormone receptors were oestrogen receptor and progesterone receptor.15 The growth factors were connective tissue growth factor (CTGF), basic fibroblast growth factor (bFGF), insulin-like growth factor type 1 (IGF1), nerve growth factor (NGF), transforming growth factor beta (TGFβ) and vascular endothelial growth factor (VEGF).15 The inflammatory mediators were cyclooxygenase 2 (COX2), interleukin 1 beta (IL1β), inducible nitric oxide synthase (iNOS), and tumour necrosis factor alpha (TNFα).19
Mechanical
A total of 25 rabbits were assigned to mechanical testing (Fig. 1) in groups comprising (1) No OVH–No scar (n = 4), (2) No OVH–6 week scar (n = 4), (3) No OVH–14 week scar (n = 4), (4) OVH–No scar (n = 4), (5) OVH–6 week scar (n = 5), and (6) OVH–14 week scar (n = 4). One MCL from each animal was tested mechanically, and its contralateral MCL was used to tune the mechanical testing system or for a different study.
Immediately after the rabbits were killed, hind limbs were disarticulated at the hip and ankle, and soft tissues were removed except menisci and ligaments. The femur and tibia were cut to accommodate the grips for the mechanical testing system (MTS Systems Corporation, Minneapolis, Minnesota).16 The tibia was secured in the upper grip which was then attached to a 500 N load cell on the hydraulic actuator and positioned with the MCL aligned with the load axis of the actuator. Load was set to zero before the femur was secured in the lower grip, with the knee at 70° of flexion. Displacement was then set to zero.
The knee joint underwent two cycles of 5 N compression and 2 N tension at 1 mm/min. The lateral collateral ligament, menisci, and cruciate ligaments were removed to isolate the MCL. Two additional compression–tension cycles were stopped at 0.1 N tension to establish ‘ligament zero’ and measure ligament laxity. Ligament geometry (MCL length and cross-sectional area) was then measured.22,23 Once the environment chamber was installed and equilibrated at 37°C and 99% relative humidity, two compression–tension cycles were performed to re-establish ligament zero.
Cyclic creep testing loaded the ligament for 30 cycles at 1 Hz from ligament zero to a force corresponding to a stress of 4.1 MPa. Static creep testing loaded the ligament to a force corresponding to 4.1 MPa and held that force for 20 minutes. After a 20-minute recovery period, the ligament was elongated to failure at 20 mm/min.
Ligament laxity was defined as the displacement of the isolated MCL between where compression was first detected (0.1 N compression) and tension was first detected (0.1 N tension). Strain was defined as deformation divided by MCL length. Total creep strain was defined as the increase in strain from the peak of the first cycle in cyclic creep to the end of 20 minutes of static creep. Failure stress was defined as the maximum force achieved in the monotonic failure test divided by cross-sectional area.
Statistical analysis
The effect of surgical menopause compared OVH–No scar with No OVH–No scar, OVH–6 week scar with No OVH–6 week scar, and OVH–14 week scar with No OVH–14 week scar. The effect of ligament healing without surgical menopause compared No OVH–6 week scar with No OVH–No scar, and No OVH–14 week scar with No OVH–No scar. The effect of ligament healing with surgical menopause compared OVH–6 week scar with OVH–No scar, and OVH–14 week scar with OVH–No scar. Analysis of variance with linear contrasts for the specific comparisons of interest were performed, where significance was set at p ≤ 0.05.
Pilot data for the effect of surgical menopause on healing ligaments were not available, therefore we estimated the difference to detect for the effect of surgical menopause on healing ligaments to be 30% of the difference between normal and healing ligaments, based on previous data comparing normal MCLs and six week bilateral MCL scars for total creep strain24and failure stress.25 For total creep strain, we required n = 4 independent samples to detect a difference of 0.42, with a standard deviation of 0.21 (power = 0.80 and alpha = 0.05). For failure stress, we required n = 4 independent samples to detect a difference of 24, with a standard deviation of 12 (power = 0.80 and alpha = 0.05).
Results
Molecular
Surgical menopause in normal MCLs revealed eight significant differences comparing OVH–No scar with No OVH–No scar (p ≤ 0.05; Fig. 2). Surgical menopause in early healing MCLs revealed three significant differences comparing OVH–6 week scar with No OVH–6 week scar, and three separate significant differences comparing OVH–14 week scar with No OVH–14 week scar (p ≤ 0.05; Fig. 2). For normal MCLs, upregulation of messenger (m) RNA levels for collagen III, collagen V, biglycan, MMP3, oestrogen receptor, CTGF, VEGF, and COX2 were observed with surgical menopause. For early healing MCLs, collagen V, CathK and TNFα were downregulated for six week scars, and biglycan, progesterone receptor and VEGF were downregulated for 14 week scars with surgical menopause.
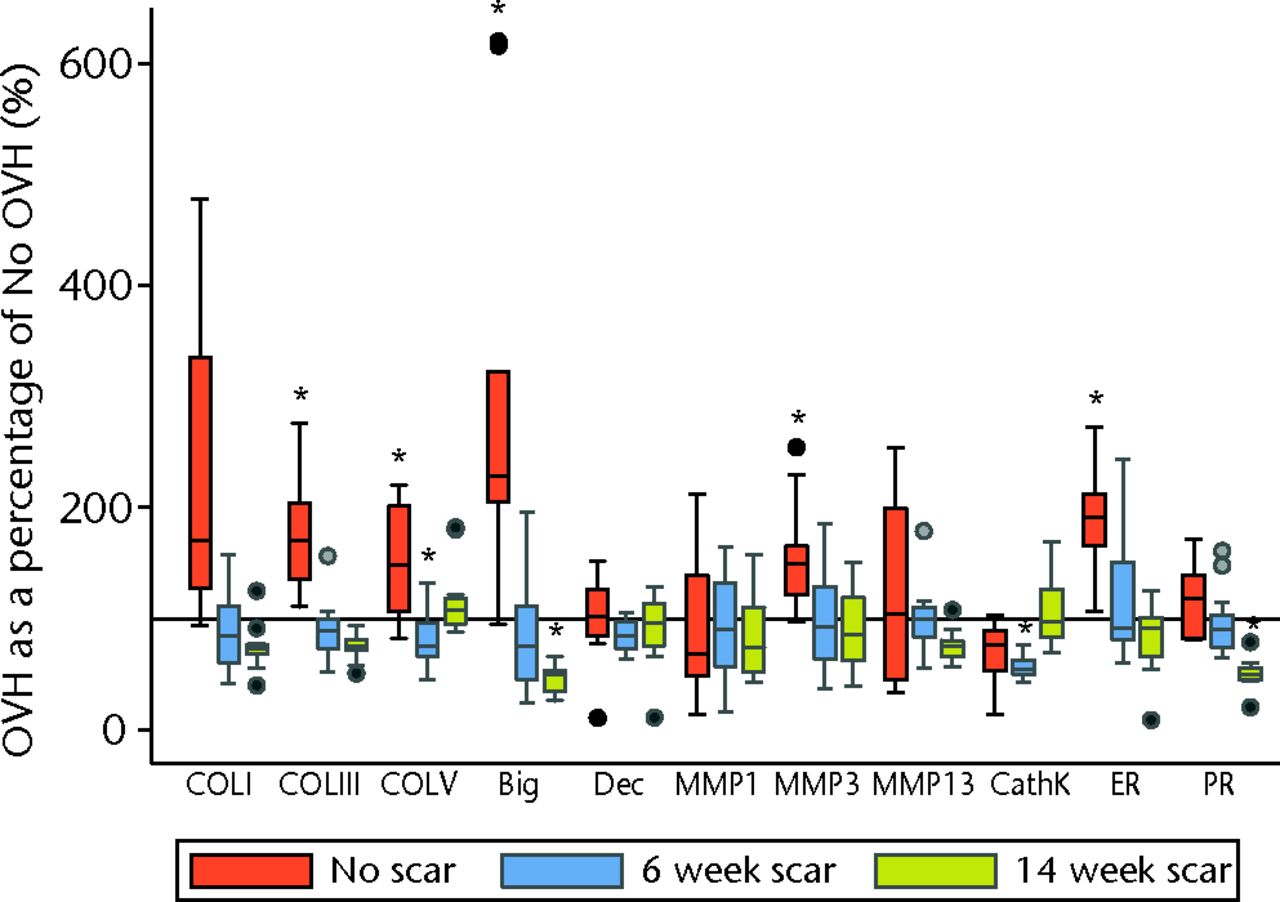
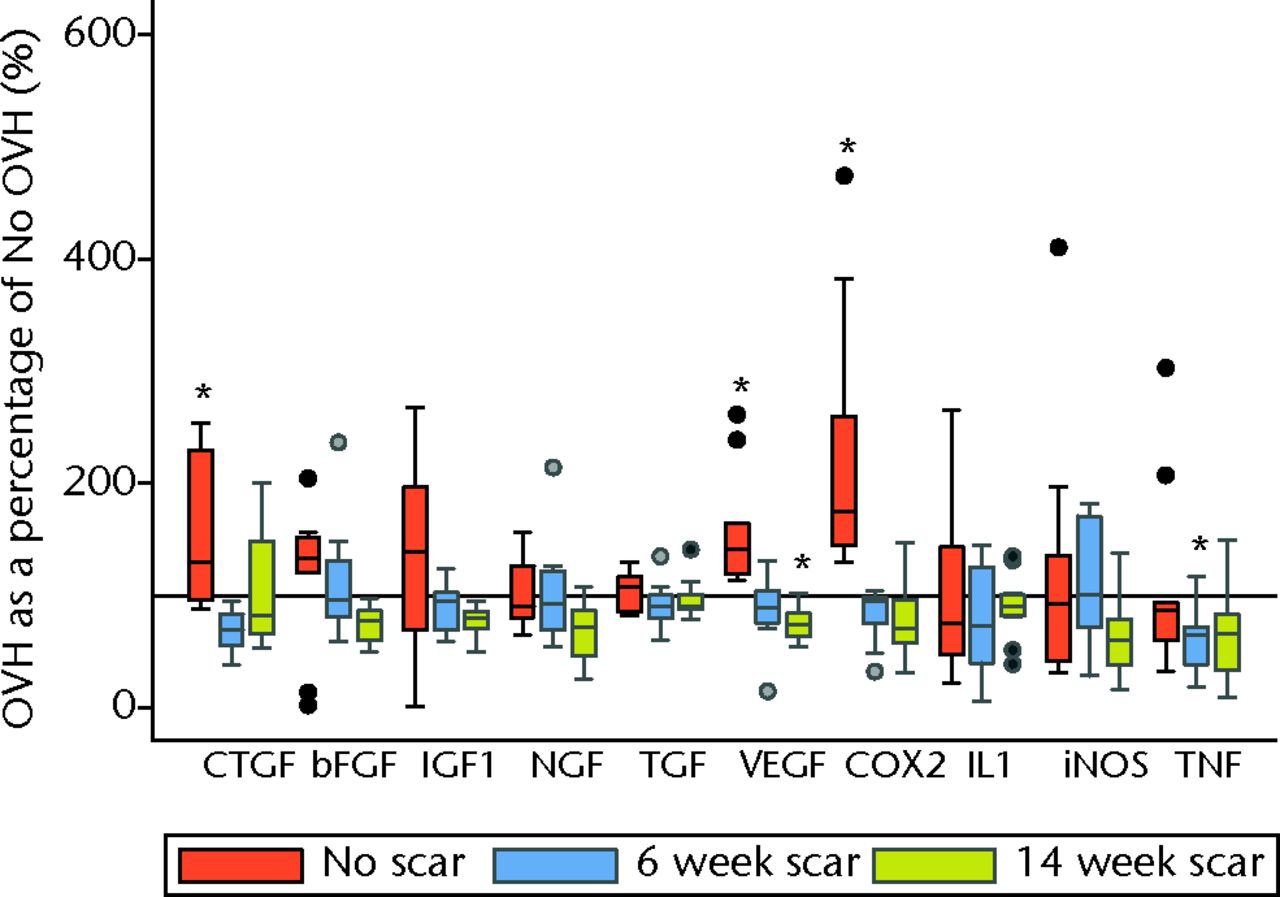
Figs. 2a - 2b
Graphs showing surgical menopause in normal and healing ligaments for a) collagens, proteoglycans, proteinases, hormone receptors and b) growth factors and inflammatory mediators. (* OVH different from No OVH; p ≤ 0.05. Horizontal reference line is 100% OVH as a percentage of No OVH; bFGF, basic fibroblast growth factor; Big, biglycan; CathK, cathepsin K proteinase; COL, collagen; COX2, cyclooxygenase 2; CTGF, connective tissue growth factor; Dec, decorin; ER, oestrogen receptor; IGF1, insulin-like growth factor type 1; IL1, interleukin 1 beta; iNOS, inducible nitric oxide synthase; MMP, matrix metalloproteinase; NGF, nerve growth factor; OVH, ovariohysterectomy; PR, progesterone receptor; TGF, transforming growth factor; TNF, tumour necrosis factor; VEGF, vascular endothelial growth factor).
Ligament healing without surgical menopause revealed 11 significant differences comparing No OVH–6 week scar with No OVH–No scar, and 11 significant differences comparing No OVH–14 week scar with No OVH–No scar (p ≤ 0.05, Table I). For both 6 and 14 week scars, upregulation of mRNA levels for collagen I, collagen III, collagen V, biglycan, MMP1, MMP13 and IGF1, and downregulation of mRNA levels of decorin and bFGF were observed with injury. CathK and TNFα were upregulated in six week scars only, whereas oestrogen and progesterone receptors were upregulated in 14 week scars only.
Table I
Ligament healing without surgical menopause
Molecules | No OVH–6 week scar/ No OVH–No scar (%) | No OVH–14 week scar/ No OVH–No scar (%) | |
---|---|---|---|
Collagens | |||
Collagen I | 639 (68)* | 484 (48)* | |
Collagen III | 194 (25)* | 148 (12)* | |
Collagen V | 267 (23)* | 154 (11)* | |
Proteoglycans | |||
Biglycan | 352 (52)* | 334 (23)* | |
Decorin | 31 (5)* | 53 (4)* | |
Proteinases | |||
MMP1 | 199 (52)* | 193 (32)* | |
MMP3 | 81 (14) | 97 (14) | |
MMP13 | 231 (30)* | 186 (13)* | |
CathK | 155 (29)* | 98 (10) | |
Hormone receptors | |||
Oestrogen receptor | 95 (14) | 141 (11)* | |
Progesterone receptor | 110 (16) | 149 (10)* | |
Growth factors | |||
CTGF | 69 (13) | 68 (9) | |
bFGF | 26 (3)* | 66 (8)* | |
IGF1 | 243 (29)* | 209 (14)* | |
NGF | 94 (9) | 108 (7) | |
TGFβ | 99 (10) | 88 (4) | |
VEGF | 102 (11) | 109 (10) | |
Inflammatory mediators | |||
COX2 | 110 (32) | 148 (28) | |
IL1β | 147 (34) | 127 (21) | |
iNOS | 134 (22) | 161 (22) | |
TNFα | 190 (28)* | 151 (25) | |
-
* (p ≤ 0.05) Data are shown as a percentage of No OVH–No scar (standard error of the mean). bFGF, basic fibroblast growth factor; CathK, cathepsin K proteinase; COX2, cyclooxygenase 2; CTGF, connective tissue growth factor; IGF1, insulin-like growth factor type 1; IL1β, interleukin 1 beta; iNOS, inducible nitric oxide synthase; MMP, matrix metalloproteinase; NGF, nerve growth factor; TGFβ, transforming growth factor beta; TNFα, tumour necrosis factor alpha; VEGF, vascular endothelial growth factor.
Ligament healing with surgical menopause revealed 12 significant differences comparing OVH–6 week scar with OVH–No scar, and ten significant differences comparing OVH–14 week scar with OVH–No scar (p ≤ 0.05, Table II). The changes that were common to both OVH–6 week scar and OVH–14 week scar were downregulation of mRNA levels for decorin, MMP3, oestrogen receptor, CTGF, bFGF, VEGF and COX2. For OVH–6 week scar only, upregulation of mRNA levels for collagen I, collagen V, MMP1, MMP13 and IGF1 were observed. For OVH–14 week scar only, downregulation of mRNA levels for collagen III, biglycan and progesterone receptor were observed.
Table II
Ligament healing with surgical menopause
Molecules | OVH–6 week scar/ OVH–No scar (%) | OVH–14 week scar/ OVH–No scar (%) |
---|---|---|
Collagens | ||
Collagen I | 247 (29)* | 155 (12) |
Collagen III | 98 (9) | 62 (3)* |
Collagen V | 143 (12)* | 119 (9) |
Proteoglycans | ||
Biglycan | 101 (17) | 52 (4)* |
Decorin | 27 (1)* | 49 (5)* |
Proteinases | ||
MMP1 | 203 (28)* | 178 (23) |
MMP3 | 51 (7)* | 56 (6)* |
MMP13 | 184 (17)* | 113 (6) |
CathK | 128 (6) | 150 (12) |
Hormone receptors | ||
Oestrogen receptor | 59 (8)* | 60 (6)* |
Progesterone receptor | 89 (8) | 63 (5)* |
Growth factors | ||
CTGF | 30 (2)* | 45 (6)* |
bFGF | 25 (3)* | 42 (3)* |
IGF1 | 157 (10)* | 116 (6) |
NGF | 93 (12) | 71 (8) |
TGFβ | 86 (5) | 81 (4) |
VEGF | 55 (6)* | 51 (3)* |
Inflammatory mediators | ||
COX2 | 40 (3)* | 50 (6)* |
IL1β | 112 (19) | 111 (10) |
iNOS | 122 (18) | 87 (14) |
TNFα | 112 (16) | 93 (16) |
-
* (p ≤ 0.05) Data are shown as a percentage of OVH–No scar (standard error of the mean). bFGF, basic fibroblast growth factor; CathK, cathepsin K proteinase; COX2, cyclooxygenase 2; CTGF, connective tissue growth factor; IGF1, insulin-like growth factor type 1; IL1β, interleukin 1 beta; iNOS, inducible nitric oxide synthase; MMP, matrix metalloproteinase; NGF, nerve growth factor; OVH, ovariohysterectomy; TGFβ, transforming growth factor beta; TNFα, tumor necrosis factor alpha; VEGF, vascular endothelial growth factor
Mechanical
Surgical menopause in normal MCLs (OVH–No scar versus No OVH–No scar) revealed no differences in cross-sectional area, ligament laxity, total creep strain, or failure stress (Figs 3 to 6). Likewise, surgical menopause in early healing MCLs (OVH–6 week scar versus No OVH–6 week scar, and OVH–14 week scar versus No OVH–14 week scar) revealed no differences in cross-sectional area, ligament laxity, total creep strain, or failure stress (Figs 3 to 6).
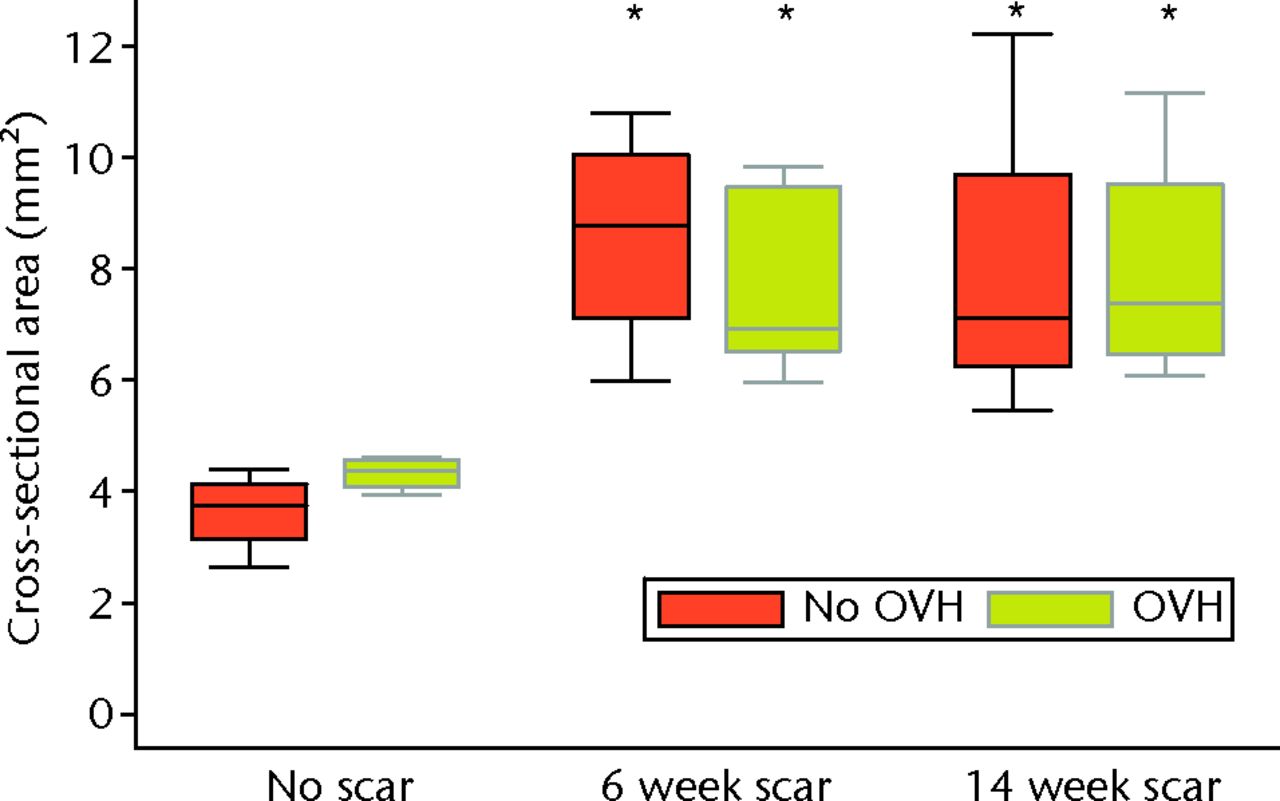
Fig. 3
Graph showing cross-sectional area of normal and healing ligaments with or without surgical menopause (* different from No scar with same condition of No OVH or OVH; p ≤ 0.05; OVH, ovariohysterectomy).
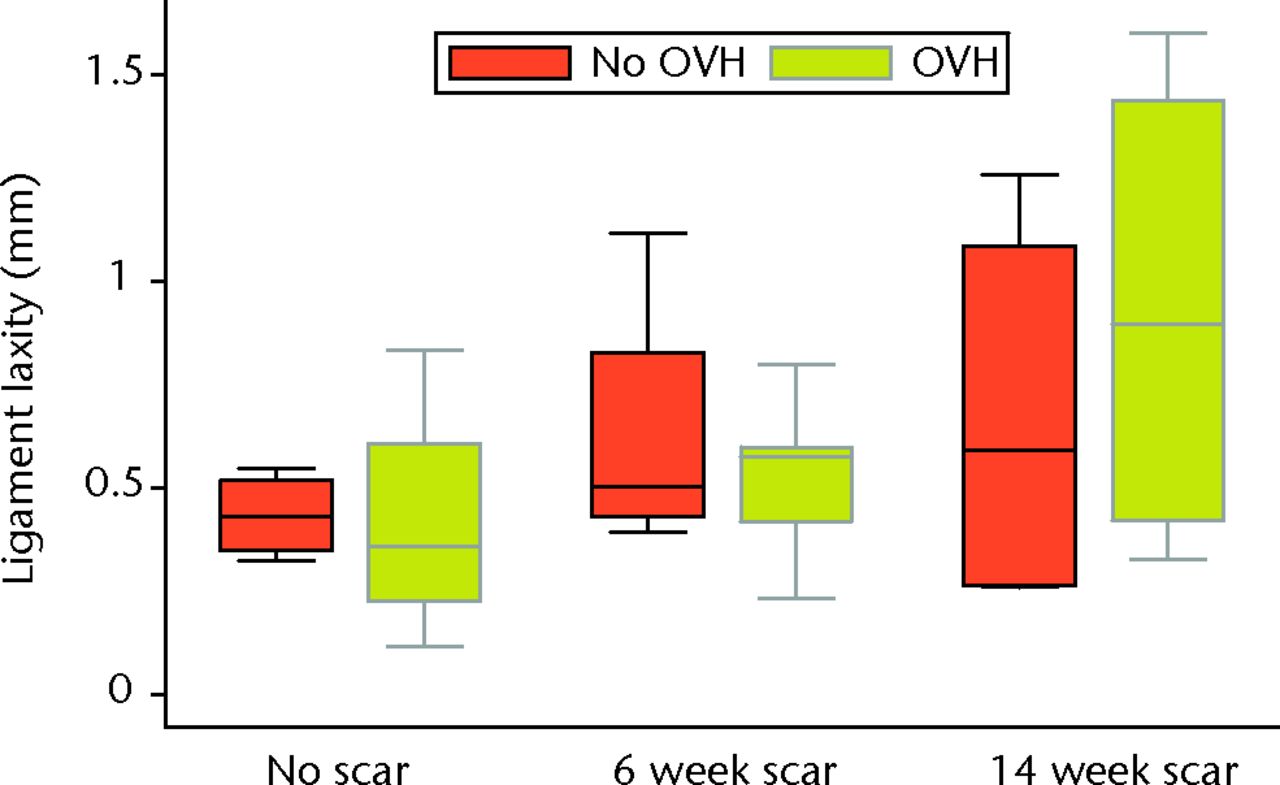
Fig. 4
Graph showing ligament laxity of normal and healing ligaments with or without surgical menopause (OVH, ovariohysterectomy).
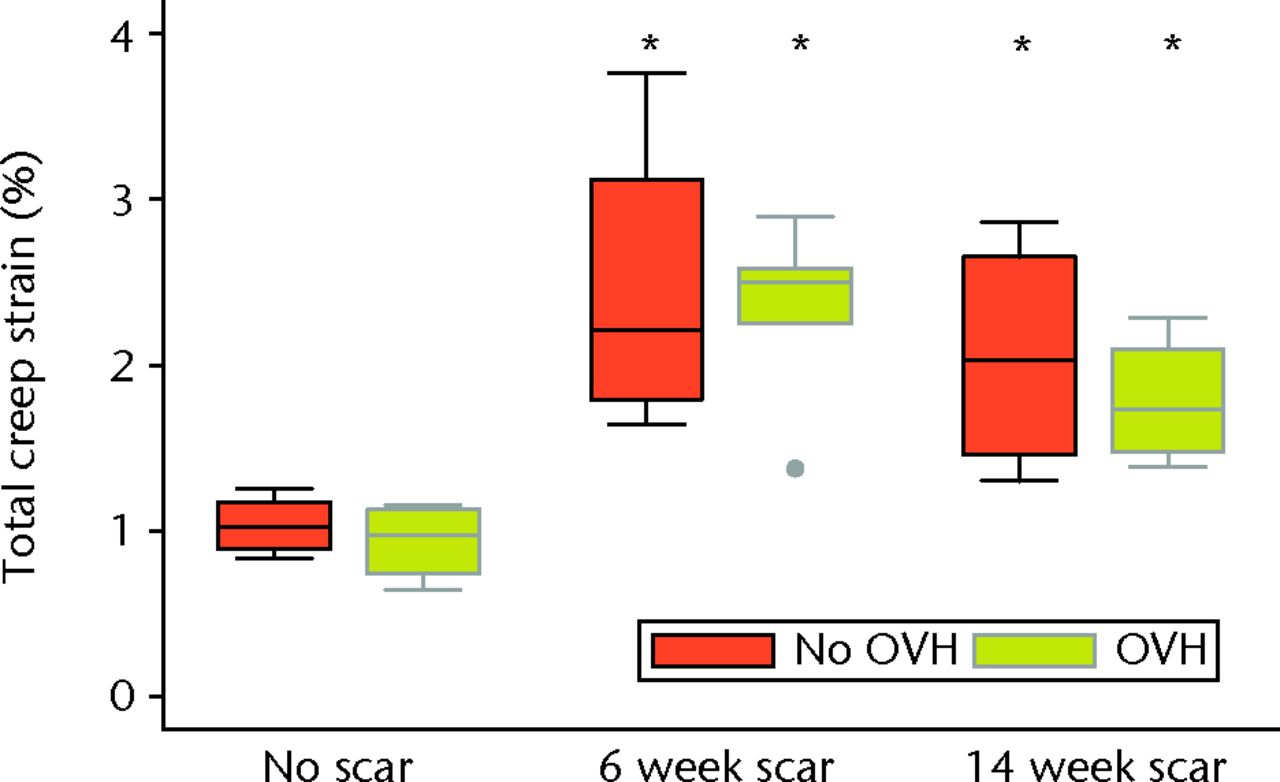
Fig. 5
Graph showing total creep strain of normal and healing ligaments with or without surgical menopause (* different from No scar with same condition of No OVH or OVH; p ≤ 0.05; OVH, ovariohysterectomy).
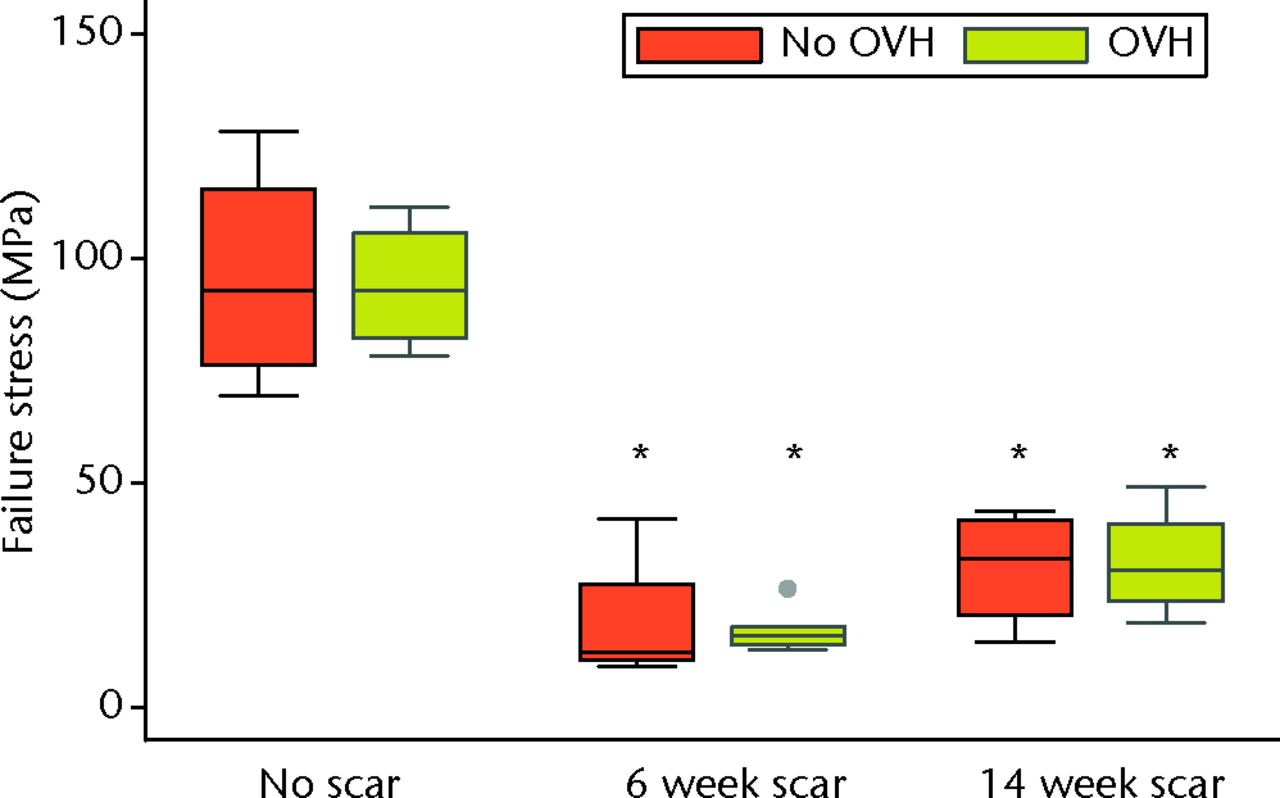
Fig. 6
Graph showing failure stress of normal and healing ligaments with or without surgical menopause (* different from No scar with same condition of No OVH or OVH; p ≤ 0.05; OVH, ovariohysterectomy).
Without surgical menopause, ligament healing resulted in an increased cross-sectional area comparing No OVH–6 week scar and No OVH–14 week scar with No OVH–No scar (p ≤ 0.05, Fig. 3). No differences were detected for ligament laxity (Fig. 3). No differences were detected for ligament laxity (Fig. 4). No OVH–6 week scar and No OVH–14 week scar had increased total creep strain compared with No OVH–No scar (p ≤ 0.05, Fig. 5). No OVH–6 week scar and No OVH–14 week scar had decreased failure stress compared with No OVH–No scar (p ≤ 0.05, Fig. 6).
As with healing without surgical menopause, ligaments healing with surgical menopause showed increased cross-sectional area and total creep strain when comparing OVH–6 week scar and OVH–14 week scar with OVH–No scar (p ≤ 0.05; Figs 3 and 5). No differences were detected for ligament laxity (Fig. 4). No differences were detected for ligament laxity (Fig. 4). Like healing without surgical menopause, ligaments healing with surgical menopause had decreased failure stress when comparing OVH–6 week scar and OVH–14 week scar with OVH–No scar (p ≤ 0.05, Fig. 6).
Discussion
Early ligament healing after surgical menopause resulted in increased total creep strain and decreased failure stress, which was similar to the findings for early ligament healing without surgical menopause. Surgical menopause did not produce changes in total creep strain or failure stress in either normal or early healing MCLs. However, normal MCLs with surgical menopause exhibited changes in mRNA levels for molecules from all categories evaluated including collagens, proteoglycans, proteinases, hormone receptors, growth factors, and inflammatory mediators. Interestingly, early healing MCLs with surgical menopause had fewer changes, with collagens, proteinases and inflammatory mediators showing a difference at six weeks of healing, and proteoglycans, growth factors and hormone receptors showing a difference at 14 weeks of healing.
Surgical menopause did not alter healing ligament mechanical properties. The low-load and high-load mechanical properties were not different when comparing ligament injuries that healed with or without surgical menopause. The mechanical properties of healing ligaments were inferior to normal ligaments, regardless of whether surgical menopause was induced or not. Consistent with previous findings, increased total creep strain16,24 and decreased failure stress17,25 were found when comparing healing MCLs to normal MCLs. Decreased failure stress of healing ligaments was detected, even though one OVH–No scar MCL triggered the load cell safety limit before complete failure and the maximum recorded force was used as a conservative estimate (Fig. 6). Most ligaments failed in the midsubstance, with four healing ligament failures at or near the tibial insertion, which is similar to previous findings.17 Surgical menopause did not affect the failure properties of MCLs in these rabbits that underwent ovariohysterectomy, which was consistent with findings for MCLs from rabbits that underwent ovariectomy26 as well as MCLs and ACLs from sheep that underwent ovariectomy,27 ACLs from monkeys that underwent ovariectomy,28 and ACLs from rats at different stages of the oestrous cycle.7 Furthermore, surgical menopause did not further impair mechanical properties of healing ligaments compared with ligaments that healed without surgical menopause. This lack of mechanical change occurred despite significant molecular changes. Interestingly, significant molecular changes without corresponding mechanical changes were observed when pregnancy occurred during ligament healing in this same model.29 Ligament injury and subsequent healing had a greater influence on mechanical behaviour than hormonal changes.
The findings presented in this study raise the question of why surgical menopause does not impair healing of an injured knee ligament, but does appear to modify skin wound healing.4 Firstly, the rabbits used were otherwise young (one year old) and healthy. Therefore, healing of ligaments may be influenced more by factors other than systemic sex hormones (e.g. ageing). Secondly, the mechanical environment in the ligament healing process may be very different from that of skin and the impact of such mechanical loading may override biological factors. Thirdly, and related to the second point, is the observation that OVH led to many significant alterations in cell metabolism (as evidenced by mRNA levels for relevant molecules) at six and 14 weeks post-injury, but these changes did not translate into overtly altered recovery of mechanical integrity. However, future studies should investigate whether these mRNA changes were reflected in protein alterations. In spite of this limitation, it would appear that mechanical considerations may trump biology in this timeframe.
Other study limitations relate to the preclinical model. Rabbits have oestrous cycles, rather than human menstrual cycles, and are induced ovulators, having basal oestrogen levels when not induced. This animal model allowed for the evaluation of ligament healing at different intervals following surgical menopause. The well-documented MCL gap injury model was assessed at six week and 14 week healing intervals, which generally relate to cell proliferation with matrix production and matrix remodeling stages, respectively.1 Although molecular and mechanical assessments were undertaken in the current study, morphological and biochemical assessments could have been performed to investigate the effect of surgical menopause, as with the effect of ligament healing (reviewed in Thornton et al16). Serum oestrogen levels were not measured for rabbits that underwent ovariohysterectomy in the current study; however, previous studies have demonstrated decreased serum oestrogen levels in rabbits that underwent ovariectomy at time points varying from two to 14 weeks following surgical menopause.11-14 If the data from the current study were used as pilot data for a future study on the effect of surgical menopause on healing ligaments, the minimum sample size required for a power of 80% would be 73 for comparisons of total creep strain and failure stress. A study with this sample size is not likely to be considered practical or ethical.
In conclusion, healing ligaments had inferior mechanical properties compared with uninjured ligaments, whether surgical menopause was induced or not. Surgical menopause did not affect the mechanical properties of normal or early healing MCLs in a rabbit model. The results in this preclinical model suggest that menopause may result in no further impairment to the ligament healing process.
Acknowledgements: The authors gratefully acknowledge the technical assistance of S. Bailey, N. Chamberland, K. Fitzpatrick, X. Shao and H. Tsao.
1 Frank CB , HartDA, ShriveNG. Molecular biology and biomechanics of normal and healing ligaments-a review. Osteoarthritis Cartilage1999;7:130–140. Google Scholar
2 Frank C , AmielD, AkesonWH. Healing of the medial collateral ligament of the knee. A morphological and biochemical assessment in rabbits. Acta Orthop Scand1983;54:917–923.CrossrefPubMed Google Scholar
3 Ashcroft GS , DodsworthJ, van BoxtelE, et al.Estrogen accelerates cutaneous wound healing associated with an increase in TGF-beta1 levels. Nat Med1997;3:1209–1215.CrossrefPubMed Google Scholar
4 Gilliver SC , AshworthJJ, AshcroftGS. The hormonal regulation of cutaneous wound healing. Clin Dermatol2007;25:56–62.CrossrefPubMed Google Scholar
5 Sciore P , FrankCB, HartDA. Identification of sex hormone receptors in human and rabbit ligaments of the knee by reverse transcription-polymerase chain reaction: evidence that receptors are present in tissue from both male and female subjects. J Orthop Res1998;16:604–610.CrossrefPubMed Google Scholar
6 Liu SH , al-ShaikhR, PanossianV, et al.Primary immunolocalization of estrogen and progesterone target cells in the human anterior cruciate ligament. J Orthop Res1996;14:526–533.CrossrefPubMed Google Scholar
7 Rau MD , RenoufD, BenfieldD, et al.Examination of the failure properties of the anterior cruciate ligament during the estrous cycle. Knee2005;12:37–40.CrossrefPubMed Google Scholar
8 Hart DA , AchariY. Alterations to cell metabolism in connective tissues of the knee after ovariohysterectomy in a rabbit model: Are there implications for the post-menopausal athlete?Br J Sports Med2010;44:867–871. Google Scholar
9 Kinney RC , SchwartzZ, WeekK, LotzMK, BoyanBD. Human articular chondrocytes exhibit sexual dimorphism in their responses to 17β-estradiol. Osteoarthritis Cartilage2005;13:330–337. Google Scholar
10 Achari Y , LuT, HartDA. Polymorphisms in the promoter regions for human MMP-1 and MMP-13 lead to differential responses to the alpha and beta isoforms of estrogen receptor and their ligand in vitro. Biochim Biophys Acta2008;1782:391–400.CrossrefPubMed Google Scholar
11 Aikawa K , SuginoT, MatsumotoS, et al.The effect of ovariectomy and estradiol on rabbit bladder smooth muscle contraction and morphology. J Urol2003;170:634–637.CrossrefPubMed Google Scholar
12 Komatsuda T , SugitaT, SanoH, et al.Does estrogen alter the mechanical properties of the anterior cruciate ligament? An experimental study in rabbits. Acta Orthop2006;77:973–980.CrossrefPubMed Google Scholar
13 Yang FL , HuKQ, WangX, et al.Combination of raloxifene, aspirin and estrogen as novel paradigm of hormone replacement therapy in rabbit model of menopause. Acta Pharmacol Sin2011;32:1031–1037.CrossrefPubMed Google Scholar
14 Abramov Y , HirschE, IlievskiV, GoldbergRP, SandPK. Transforming growth factor beta1 gene expression during vaginal wound healing in a rabbit menopause model. BJOG2013;120:251–256.CrossrefPubMed Google Scholar
15 Achari Y , RenoCR, TsaoH, MorckDW, HartDA. Influence of timing (pre-puberty or skeletal maturity) of ovariohysterectomy on mRNA levels in corneal tissues of female rabbits. Mol Vis2008;14:443–455.PubMed Google Scholar
16 Thornton GM , LeaskGP, ShriveNG, FrankCB. Early medial collateral ligament scars have inferior creep behaviour. J Orthop Res2000;18:238–246.CrossrefPubMed Google Scholar
17 Chimich D , FrankC, ShriveN, DougallH, BrayR. The effects of initial end contact on medial collateral ligament healing: a morphological and biomechanical study in a rabbit model. J Orthop Res1991;9:37–47.CrossrefPubMed Google Scholar
18 Reno C , MarchukL, ScioreP, FrankCB, HartDA. Rapid isolation of total RNA from small samples of hypocellular, dense connective tissues. Biotechniques1997;22:1082–1086.CrossrefPubMed Google Scholar
19 Reno C , BoykiwR, MartinezML, HartDA. Temporal alterations in mRNA levels for proteinases and inhibitors and their potential regulators in the healing medial collateral ligament. Biochem Biophys Res Commun1998;252:757–763.CrossrefPubMed Google Scholar
20 Hellio Le Graverand MP , EggererJ, ScioreP, et al.Matrix metalloproteinase-13 expression in rabbit knee joint connective tissues: influence of maturation and response to injury. Matrix Biol2000;19:431–441.CrossrefPubMed Google Scholar
21 Achari Y , RenoCR, MorckDW, HartDA. Influence of bilateral medial collateral ligament injury on mRNA expression in distal corneal tissues of control and ovariohysterectomized rabbits. Cornea2010;29:418–431.CrossrefPubMed Google Scholar
22 Thornton GM , BaileySJ. Repetitive loading damages healing ligaments more than sustained loading demonstrated by reduction in modulus and residual strength. J Biomech2012;45:2589–2594.CrossrefPubMed Google Scholar
23 Shrive NG , LamTC, DamsonE, FrankCB. A new method of measuring the cross-sectional area of connective tissue structures. J Biomech Eng1988;110:104–109.CrossrefPubMed Google Scholar
24 Thornton GM , ShriveNG, FrankCB. Healing ligaments have decreased cyclic modulus compared to normal ligaments and immobilization further compromises healing ligament response to cyclic loading. J Orthop Res2003;21:716–722.CrossrefPubMed Google Scholar
25 Thornton GM , JohnsonJC, MaserRV, et al.Strength of medial structures of the knee joint are decreased by isolated injury to the medial collateral ligament and subsequent joint immobilization. J Orthop Res2005;23:1191–1198.CrossrefPubMed Google Scholar
26 Rasanen T , MessnerK. Estrogen-dependent tensile properties of the rabbit knee medial collateral ligament. Scan J Med Sci Sports2000;10:20–27.CrossrefPubMed Google Scholar
27 Strickland SM , BelknapTW, TurnerSA, WrightTM, HannafinJA. Lack of hormonal influences on mechanical properties of sheep knee ligaments. Am J Sports Med2003;31:210–215.CrossrefPubMed Google Scholar
28 Wentorf FA , SudohK, MosesC, ArendtEA, CarlsonCS. The effects of estrogen on material and mechanical properties of the intra- and extra-articular knee structures. Am J Sports Med2006;34:1948–1952.CrossrefPubMed Google Scholar
29 Hart DA , RenoC, FrankCB, ShriveNG. Pregnancy affects cellular activity, but not tissue mechanical properties, in the healing rabbit medial collateral ligament. J Orthop Res2000;18:462–471. Google Scholar
Funding statement:
This work was supported by the Natural Sciences and Engineering Research Council of Canada (GMT: 261630), Canadian Institutes of Health Research Institute of Musculoskeletal Health and Arthritis (GMT: 94006), Canadian Institutes of Health Research Institute for Gender and Health (DAH and GMT: 63210), and Alberta Innovates Health Solutions Osteoarthritis Team (DAH: 200700596).
Author contributions:
G. M. Thornton: Design of study; Acquisition, analysis and interpretation of data; Writing and revision of manuscript
C. R. Reno: Acquisition and analysis of data; Revision of manuscript
Y. Achari: Design of study; Analysis of data; Revision of manuscript
D. W. Morck: Acquisition of data, Revision of manuscript
D. A. Hart: Design of study, Interpretation of data, Revision of manuscript
ICMJE Conflict of Interest:
None declared
©2015 The British Editorial Society of Bone & Joint Surgery. This is an open-access article distributed under the terms of the Creative Commons Attributions licence, which permits unrestricted use, distribution, and reproduction in any medium, but not for commercial gain, provided the original author and source are credited.