Abstract
Objectives
Third-body wear is believed to be one trigger for adverse results with metal-on-metal (MOM) bearings. Impingement and subluxation may release metal particles from MOM replacements. We therefore challenged MOM bearings with relevant debris types of cobalt–chrome alloy (CoCr), titanium alloy (Ti6Al4V) and polymethylmethacrylate bone cement (PMMA).
Methods
Cement flakes (PMMA), CoCr and Ti6Al4V particles (size range 5 µm to 400 µm) were run in a MOM wear simulation. Debris allotments (5 mg) were inserted at ten intervals during the five million cycle (5 Mc) test.
Results
In a clean test phase (0 Mc to 0.8 Mc), lubricants retained their yellow colour. Addition of metal particles at 0.8 Mc turned lubricants black within the first hour of the test and remained so for the duration, while PMMA particles did not change the colour of the lubricant. Rates of wear with PMMA, CoCr and Ti6Al4V debris averaged 0.3 mm3/Mc, 4.1Â mm3/Mc and 6.4 mm3/Mc, respectively.
Conclusions
Metal particles turned simulator lubricants black with rates of wear of MOM bearings an order of magnitude higher than with control PMMA particles. This appeared to model the findings of black, periarticular joint tissues and high CoCr wear in failed MOM replacements. The amount of wear debris produced during a 500 000-cycle interval of gait was 30 to 50 times greater than the weight of triggering particle allotment, indicating that MOM bearings were extremely sensitive to third-body wear.
Cite this article: Bone Joint Res 2015;4:29–37.
Article focus
In a prior clinical study of McKee–Farrar cases (cemented, large diameter total hip arthroplasty (THA)), Howie et al1 proposed the following hip-impingement scenario for metal-on-metal (MOM) bearings used in THA, the rigid cobalt–chrome (CoCr) cup rim impacts against the CoCr head, producing a two-body wear mechanism. Large CoCr particles are released, and the circulating metal particles produce an aggressive third-body wear of CoCr surfaces. However, there appears to have been no follow-up of this novel hypothesis.
In this first simulator study of its kind, we investigated the wear response of MOM bearings to CoCr and titanium alloy (Ti6Al4V) particles introduced as clinically-relevant metal debris. Custom flakes of polymerised bone cement (PMMA) were used as control debris.
We hypothesised that particles of bone cement would have minimal effect on MOM wear, metal particles (CoCr, Ti6Al4V) would damage CoCr surfaces, increasing MOM wear by an order of magnitude and Ti6Al4V particles would smear onto CoCr surfaces, disrupting the protein lubrication and creating more severe wear.
Key messages
It is proposed that a major risk with MOM bearings is the release of metal debris that circulates the joint and provokes aggressive third-body wear. Depending on alloys used for femoral stem and acetabular shell designs, such metal debris may include CoCr, Ti6Al4V and other particles.
Our MOM simulator study showed that CoCr and Ti6Al4V particles turned test lubricants black within the first hour and resulting MOM rates of wear were elevated an order of magnitude above controls. These laboratory data appeared to mimic the high wear measured in retrieved MOM bearings and observations of black periarticular tissues.
This investigation with ten debris insertions spaced over a duration of five million cycles demonstrated consistent trends of wear (linear-regression coefficients R > 0.98) that indicated the methodology was applicable for future scientific study.
Strengths and limitations
This simulation study used what we would now consider clinically relevant particles of PMMA, CoCr and Ti6Al4V.
The methodology was chosen to represent the production of debris during one episode of hip-impingement and the consequences of abrasive wear perpetuated by the damaged CoCr surfaces during the subsequent 500 000 cycles of gait.
There are many issues facing simulation of debris formation in vivo. Some patients may sublux or impinge the hip joint at every step, while others may do so occasionally or not at all. The nature of debris production in vivo is open to speculation. In addition, metal debris may circulate the hip joint, be transported to distant tissue sites, or corrode and be eliminated. The decomposition lifetimes are unknown for metal debris. It is also unknown whether our 0.5 mg debris allotments or the number of particulates had clinical relevance.
The methodological issues are complex but this debris study may aid in the development of adverse test methods that have clinical relevance.
Introduction
Metal-on-metal (MOM) bearings used for total hip arthroplasty (THA) in the past decade have produced many cases of failure, generally grouped under the term ‘adverse reactions to wear debris' (ARMD).2,3 The majority of reports focused on cup design and implant malpositioning, with ‘edge loading’ appearing to be an important risk.4-7 However, a trigger mechanism largely overlooked is that of two-body and three-body wear.1,8-11
In the history of THA, the nemesis for metal-on-polyethylene (MPE) bearing combinations was hip impingement, debris production and third-body abrasion.12-14 Particles reported to be circulating in the joint included bone cement (polymethylmethacrylate (PMMA)), various metals (stainless steel, cobalt–chrome (CoCr), pure titanium (Ti) alloy (Ti6Al4V)) and ceramics (hydroxyapatite (HA), alumina). Various pin-on-disk (POD) wear studies have been performed to understand the abrasive wear of polyethylene surfaces15-17 while hip simulator studies have used abrasive slurries of PMMA debris.18-21 However, with respect to wear studies in MOM bearings, there appear to be only two reports.8,22 Lu et al8 investigated the effect of Ti particles and noted that wear was elevated from 0.036 mm3/Mc in MOM controls to 1.8 mm3/Mc and 14.7 mm3/Mc in two femoral heads (Fig. 1), the latter values representing an eightfold disparity. A MOM simulator study (n = 3, 36 mm) by Liao et al22 used HA particles. This study showed stable trends of wear reaching approximately 0.2 mm3/Mc over five million cycles, but with an 11 fold disparity between the high and low rates of wear.
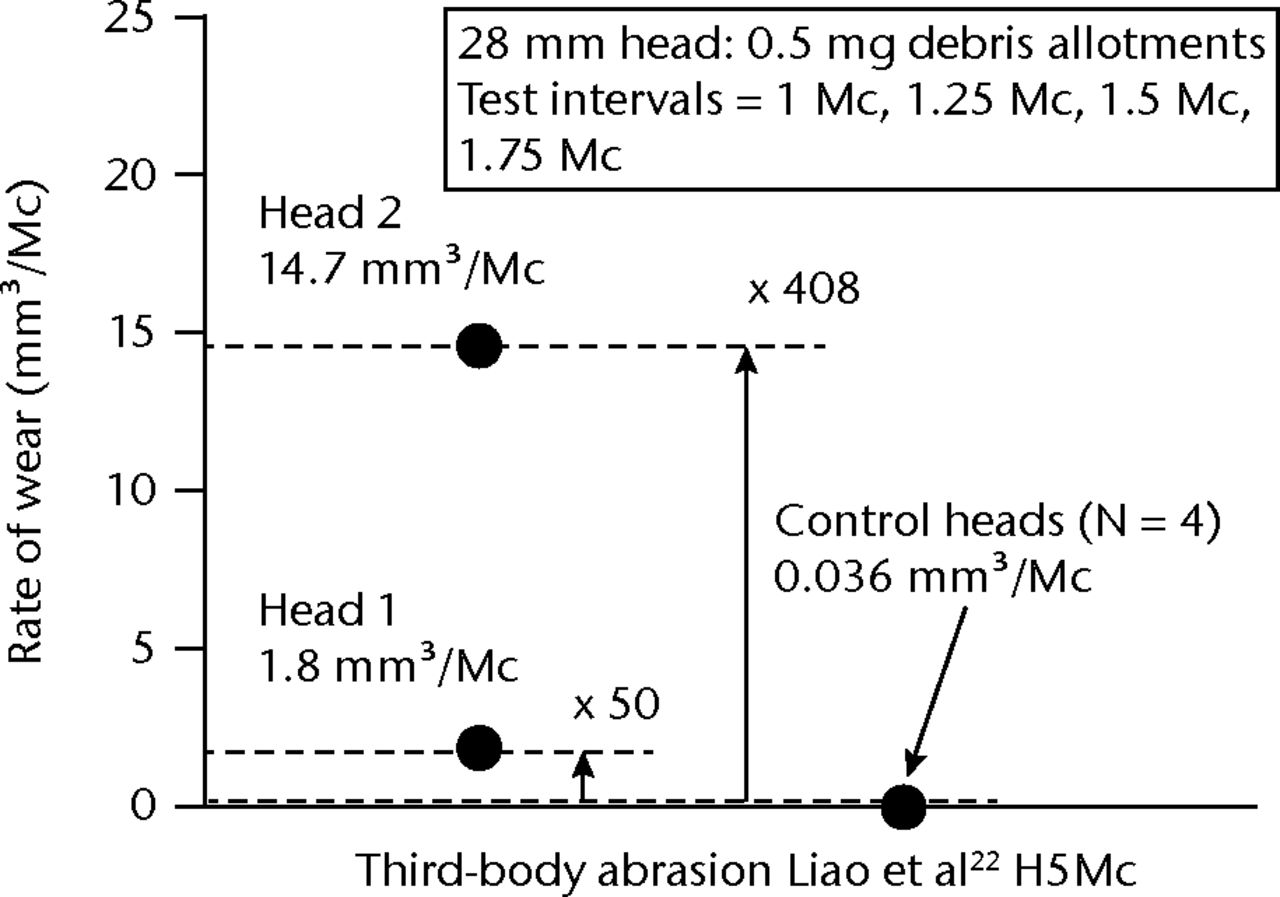
Fig. 1
Graph showing metal-on-metal wear in two femoral heads run in a simulator study22 with titanium particles.
Recent retrieval studies of modular MOM bearings have provided unequivocal evidence of two- and three-body wear damage.9,11,23-25 A prior retrieval study of cemented McKee–Farrar THA (cemented non-modular stem and cup design) proposed three abrasive-wear scenarios, the rigid CoCr cup impinges on the femoral head creating surface damage, the cup impingement releases CoCr particles and circulating clouds of CoCr particulates produce an aggressive third-body wear.1 Simulator studies of effects of metal particles abrading MOM appear scant.8 Therefore, our goal was to run an in vitro wear study of MOM bearings challenged by metal particles. Our pilot study revealed that PMMA particles had minimal effect on CoCr surfaces, whereas both CoCr and Ti6Al4V particles abraded the bearings within the first ten seconds of the test.26 Ti6Al4V was also found smeared onto CoCr surfaces. Therefore, our hypotheses for this study were that PMMA particles would not increase MOM wear, CoCr and Ti6Al4V particles would increase MOM wear by an order of magnitude, and Ti6Al4V debris would be found as a contaminating layer on CoCr bearings.
Materials and Methods
Debris models
The simulator model used cups mounted ‘inverted’ (under the head),8,22 debris was inserted at the beginning of each 500 000-cycle test interval, and ten debris insertions were spaced over the duration of the 5 Mc test (Table I).22 Determining a clinically-appropriate abrasive procedure for simulating total joint arthroplasties in vivo is fraught with uncertainty. For example, Lu et al8 inserted 0.5 mg of Ti-metal debris, Liao et al22 inserted 280 mg of HA powder and Wang and Essner19 used a slurry containing 450 mg to 4500 mg of crushed bone cement. Without a consensus from the literature, we used our standard 5 mg debris allotment.26
Table I
Summary of hip simulator studies using abrasion methods
Study | Bearings | Diameter (mm) | Cup mounting | Debris method (mg/ml) | Particle type | Particle size (µm) | No. particles | Test interval | No. sample replicates | |||||||||
---|---|---|---|---|---|---|---|---|---|---|---|---|---|---|---|---|---|---|
Lu 20008 | MOM | 28 | Inverted | Insert (0.5) | Ti | NS | 150 | 0.25 Mc | 2 | |||||||||
Liao 201022 | MOM | 36 | Inverted | Insert (280) | HA | < (100) | NS | 0.5 Mc | 3 | |||||||||
Parikh 201321 | “DHOxZr” | 38 | Anatomic 35o | Slurry (10) | P | Commercial bone cement powder (Versabond, Smith & Nephew, Memphis, Tennessee) | NA | 0.5 Mc and 1 Mc | NS | |||||||||
Halim 201426 | MOM | 38 | Inverted and anatomic 45 o | Insert (5) | P, C, T6 | Commercial bone cement powder (60 to 340) (Cobalt, Biomet, Warsaw, Indiana) | 230 C, 340 T6, 1300 P | 10 cycles | 2 each set | |||||||||
-
Insert, debris inserted between bearing surfaces; Slurry, debris mixed into serum lubricant; C, CoCr particle; DHOxZr, zirconia ceramic surface with diffusion hardened sub-surface zone; HA, hydroxyapatite particle; 1 Mc, 1 million simulator gait cycles; MOM, metal-on-metal bearing; NA, not applicable; NS, not specified; P, Proprietary bone–cement powder; Ti, titanium particle; T6, titanium alloy particle (Ti6Al4V)
The protein concentration in the diluted serum lubricant (Hyclone Ogden, Utah) was 17 mg/ml and each test chamber held approximately 400 ml volume.27,28 As in our scratch-profiling study,26 we used commercially available CoCr beads and irregularly-shaped Ti6Al4V chips that represented considerable morphological differences (Fig. 2). The range of particle size reached a peak at around 420 µm and this represented the scale of damage seen on retrieved MOM bearings.9,25 We used a fine metal file to scrape off particles of bone cement from a retrieved total knee arthroplasty. The PMMA allotments were checked by SEM and EDS methods for contaminating metal particles. SEM imaging (MA 15, Zeiss, Thornwood, New York) also characterised the morphology of the particles (Fig. 2) also characterised the morphology of the particles (Fig. 2) and the numbers of particles per allotment were estimated at 230, 340, and 1300 for PMMA, CoCr and Ti6Al4V, respectively.26
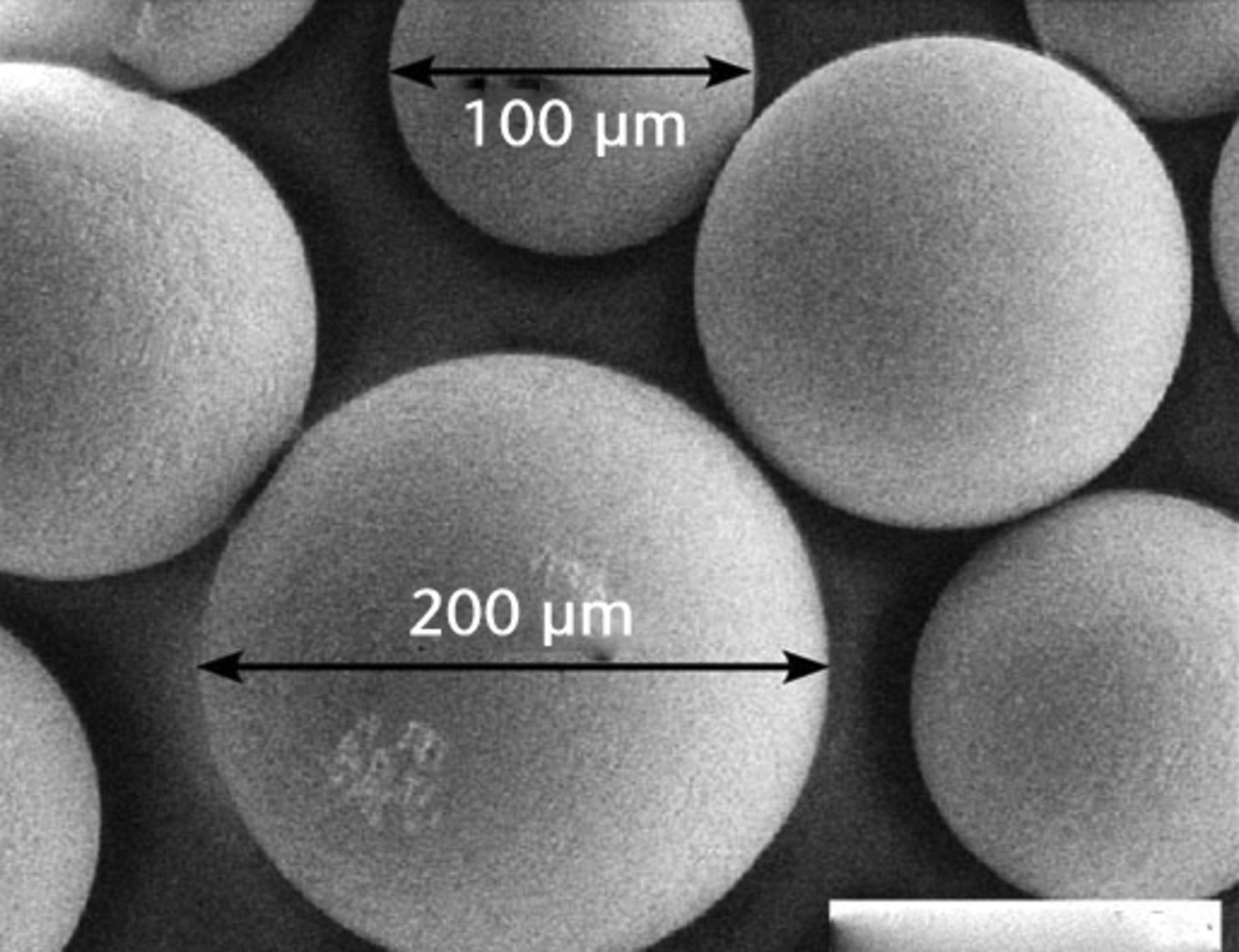
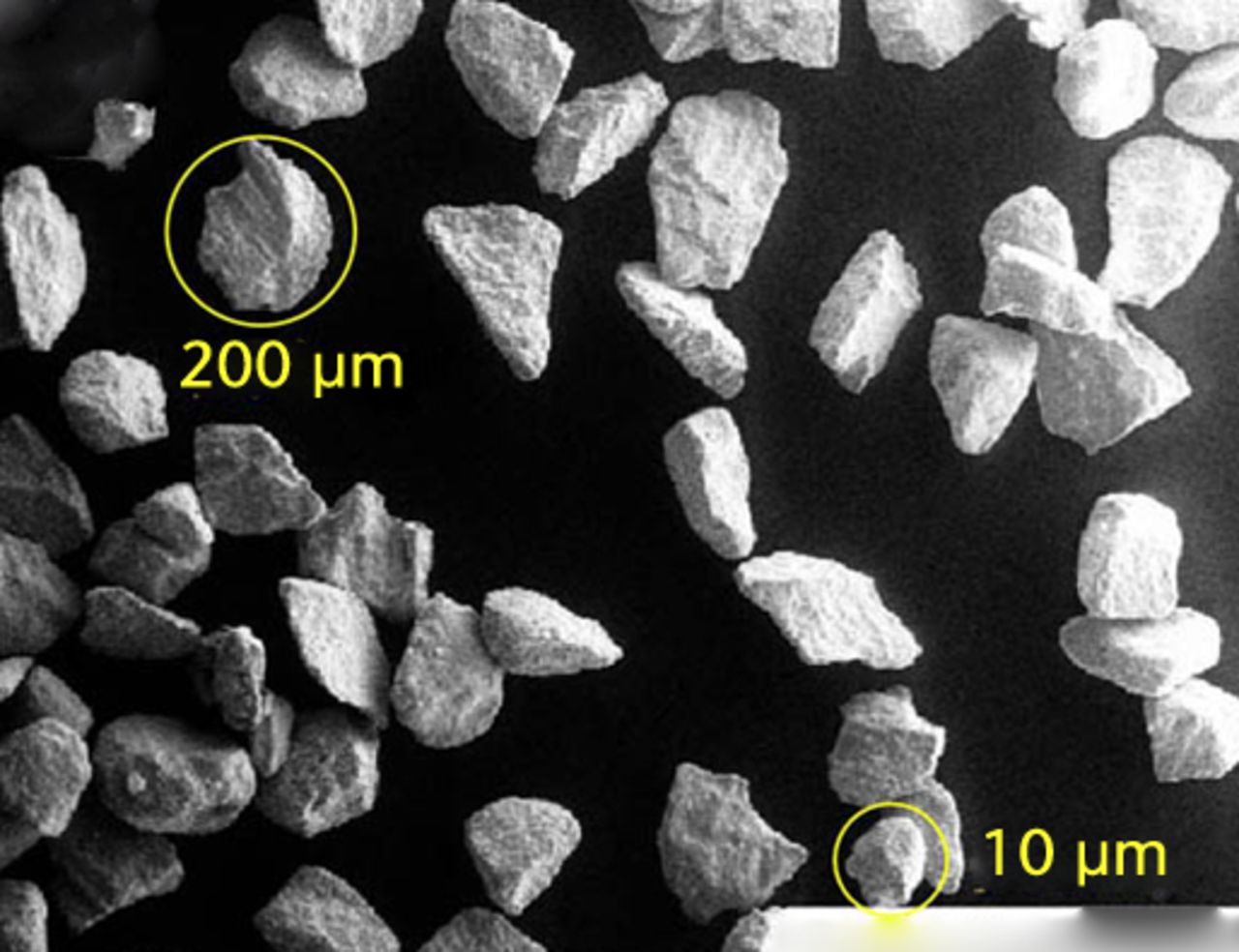

Figs. 2a - 2c
SEM imaging showed particulate morphologies including a) cobalt–chrome beads (mag. × 1000), b) titanium alloy particles (mag. × 100) and c) flakes of polymerised cement (mag. × 100).
MOM simulator wear tests
A total of nine 38 mm MOM were used with three bearings allocated to each debris treatment (wrought, high-carbon CoCr alloy: DJO Surgical, Austin, Texas). A 12-station hip simulator (Shore Western Manufacturing, Monrovia, California) was run under standard guidelines.27,28 The cups were mounted below the femoral heads as is typical for debris-insertion studies (Tables II and III). All chambers were run with a ‘clean’ lubricant to 0.8 Mc to complete the run-in wear phase. From 0.8 Mc onwards, 5 mg particle allotments26 were added at the beginning of each test interval (n = 10 intervals of 0.5 Mc duration).22 Particles were placed in each cup, loaded with the femoral head, and lubricant added to fill the chambers. Wear analysis was by weight loss and data were analysed by linear regression, with statistical analyses performed using one-way ANOVA and Dunn’s multiple comparisons. Volumetric rates of wear were calculated using specific gravity 8.26 for a CoCr alloy.27
Table II
Metal-on-metal (MOM) rates of wear assessed over 0.8 Mc to 5 Mc duration (weight loss in mg/Mc)
MOM challenges | Minimum R-value | Weight loss range min/max (mg/Mc) | Max/min wear ratios | Average weight loss (mg/Mc) | Average wear ratio |
---|---|---|---|---|---|
PMMA | 0.913 | Â Â 2.1/3.8 | 1.8 | Â Â 3 | Â Â 1 |
CoCr | 0.925 | 25.7/48.6 | 1.9 | 33.6 | 11.2 |
Ti6Al4V | 0.986 | 47.4/60.8 | 1.3 | 53.1 | 17.7 |
-
MoM, metal-on-metal; Min, minimum; Max, maximum; PMMA, polymethylmethacrylate; CoCr, cobalt–chrome; Ti6Al4V, titanium alloy
Table III
Metal-on-metal (MOM) rates of wear assessed over 0.8 Mc to 5Â Mc duration with volumetric rates of wear in mm3/Mc (shown weight loss as mm3/Mc)
MOM challenges | Volume range min/max (mm3/Mc) | Average rate of wear (mm3/Mc) |
---|---|---|
PMMA | 0.25/0.45 | 0.36 |
CoCr | 3.12/5.88 | 4.07 |
Ti6Al4V | 5.74/7.37 | 6.43 |
-
PMMA, polymethylmethacrylate bone cement; CoCr, cobalt–chrome alloy; Ti6Al4V, titanium alloy; Min, minimum; Max, maximum
Surface roughness of femoral heads
Femoral heads were examined at 2.5 Mc, 3.5 Mc and 5 Mc duration and wear zones marked and photographed pre-analysis. Femoral-head roughness and scratch profiles were measured by white light interferometry (NewView 600, Zygo Corporation, Middlefield, Connecticut). A total of 12 replicated fields of view were taken per wear scar. The large scratches evident in tests with metal debris were individually profiled on one head selected per debris group (n = 12 measurements). Scratch widths, lip heights and valley depths were compared.26 SEM imaging at 5 Mc duration further characterised the scratch topography, with EDS imaging used to identify surface contaminants (X-flash detector 4010, Bruker AXS, Madison, Wisconsin). Roughness data were assessed using two methods. The main wear zone roughness (MWZ-Ra) was assessed with exclusion of areas containing large scratches i.e. > 20 µm wide). This provided an indication of the wear-polishing effect in the MWZ-Ra method. The total inclusion method (TWZ-Ra) measured roughness that was typical of areas featuring large scratches (common after metal-debris challenge). TWZ-Ra data was also used in support of the profiling method of characterising scratch topography.
Results
MOM simulator wear tests
Run-in and steady state wear phases were completed satisfactorily to 0.8 Mc duration with ‘clean’ lubricants, all of which retained their golden yellow colour. Following the PMMA challenge (0.8 Mc to 5 Mc), the wear trends were satisfactorily linear (Table II: regression coefficients > 0.9). The resulting MOM rates of wear were < 0.45 mm3/Mc (Table III) and there was no lubricant colour change at any test interval up to 5 Mc duration (Fig. 3) and there was no lubricant colour change at any test interval up to 5 Mc duration (Fig. 3). In contrast with the CoCr challenge, the lubricants turned black within the first hour and remained black for the duration of each test interval. MOM rates of wear ranged from 3.1 mm3/Mc up to 5.9 mm3/Mc (Fig. 4). The Ti6Al4V challenge also turned lubricants black with rates of wear that ranged from 5.7 mm3/Mcto 7.4 mm3/Mc (Table III). MOM wear ratios for the CoCr and Ti6Al4V challenges versus PMMA controls were 11.2:1 and 17.7:1 (Table II). Comparing wear in metal groups with the cement group revealed statistically significant differences (p < 0.01). The Ti6A14V and CoCr groups showed only minor differences (p = 0.069). The CoCr challenge produced a MOM weight loss that was 31Â times greater than the weight of the debris allotment, and a 50 times greater weight loss in the Ti6Al4V challenge (Table IV).

Fig. 3
Image showing that serum lubricants turned black with metal debris (cobalt–chrome, 1 to 3; titanium alloy, 4 to 6) whereas the chambers used with polymethylmethacrylate debris (7 to 9) always retained the typical yellow colour (duration of test 3.5 Mc to 4.5 Mc)
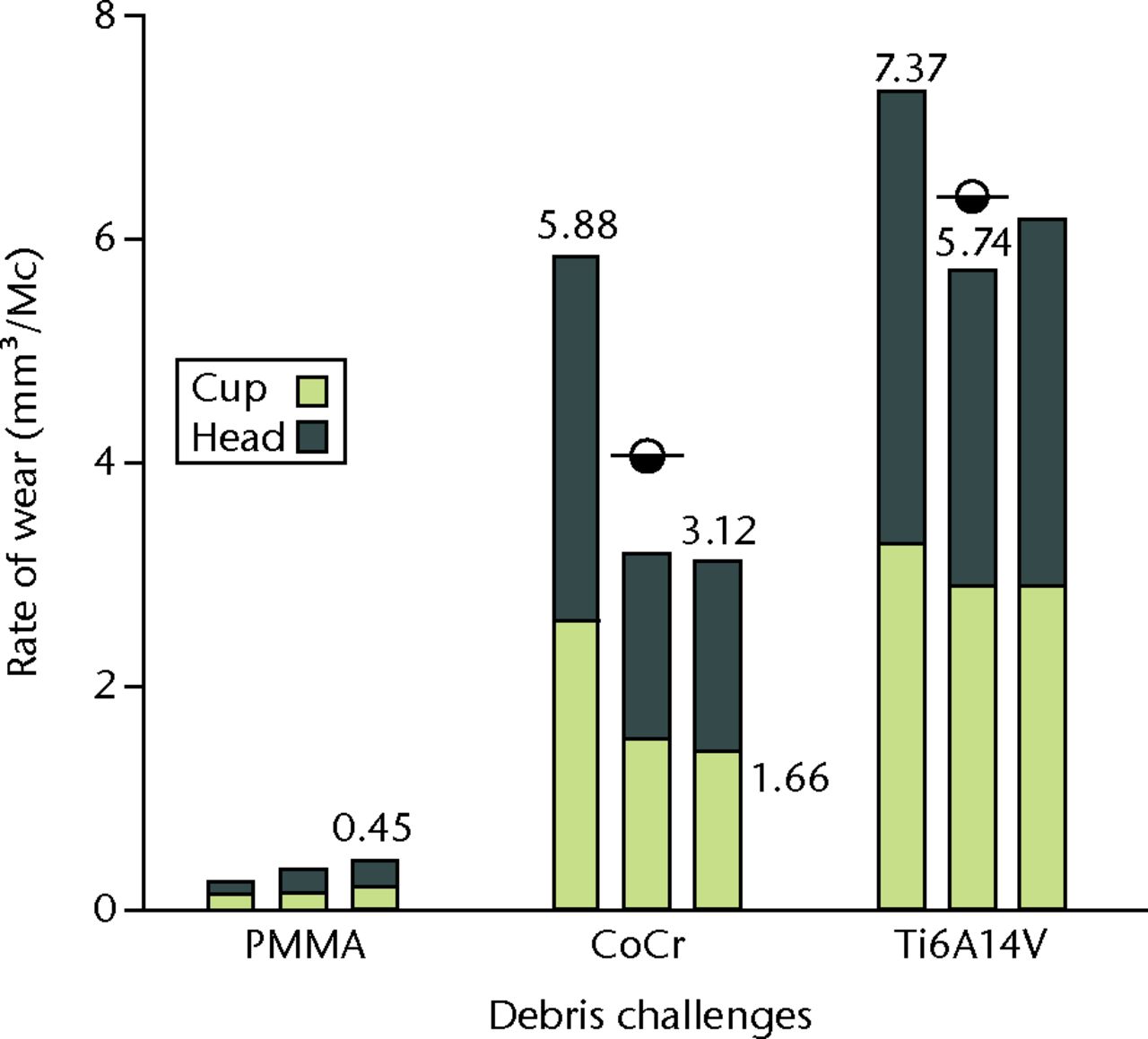
Fig. 4
Graph showing that rates of wear were approximately equal for heads and cups in each metal-on-metal pair (linear regression over 0.8 Mc to 5Mc; PMMA, polymethylmethacrylate; CoCr, cobalt–chrome; Ti6Al4V, titanium alloy).
Table IV
Metal-on-metal (MOM) wear produced over the 0.5 Mc test interval relative to the 0.5 mg allotments of debris
Average trends per 0.5 Mc interval | PMMA debris | CoCr debris | Ti6Al4V debris |
---|---|---|---|
MOM weight-loss (mg) | 1.48 | 16.81 | 26.57 |
Corrected (PMMA controls) | 15.33 | 25.09 | |
Debris allotment (mg) | Â Â 0.5 | Â Â 0.5 | |
Ratio MOM/debris | 31 | 50 | |
-
PMMA, polymethylmethacrylate bone cement; CoCr, cobalt–chrome alloy; Ti6Al4V, titanium alloy
Surface roughness of femoral heads
In each of the three test groups, the MWZ-index showed no statistically significant differences with respect to the three heads in each group, between-debris groups or overall test duration (Fig. 5). In addition, the PMMA debris challenge provided almost identical MWZ-Ra and TWZ-Ra assessments due to absence of large scratches. The metal debris challenges provided a very different result. Using the total-inclusion method (TWZ-Ra) that measured roughness typical of areas with large scratches, the CoCr particulates showed roughness elevated almost sevenfold; with Ti6Al4V particulates there was an almost tenfold elevation (Table V). SEM and EDS imaging identified islands of metal contamination containing elemental signatures of T, Al and V. Profiling of wear topography at 5 Mc duration revealed that the dramatic changes were caused by scratches 37µm to 116 µm wide, evident on all bearings challenged by metal particulates (Fig. 6, Table VI).
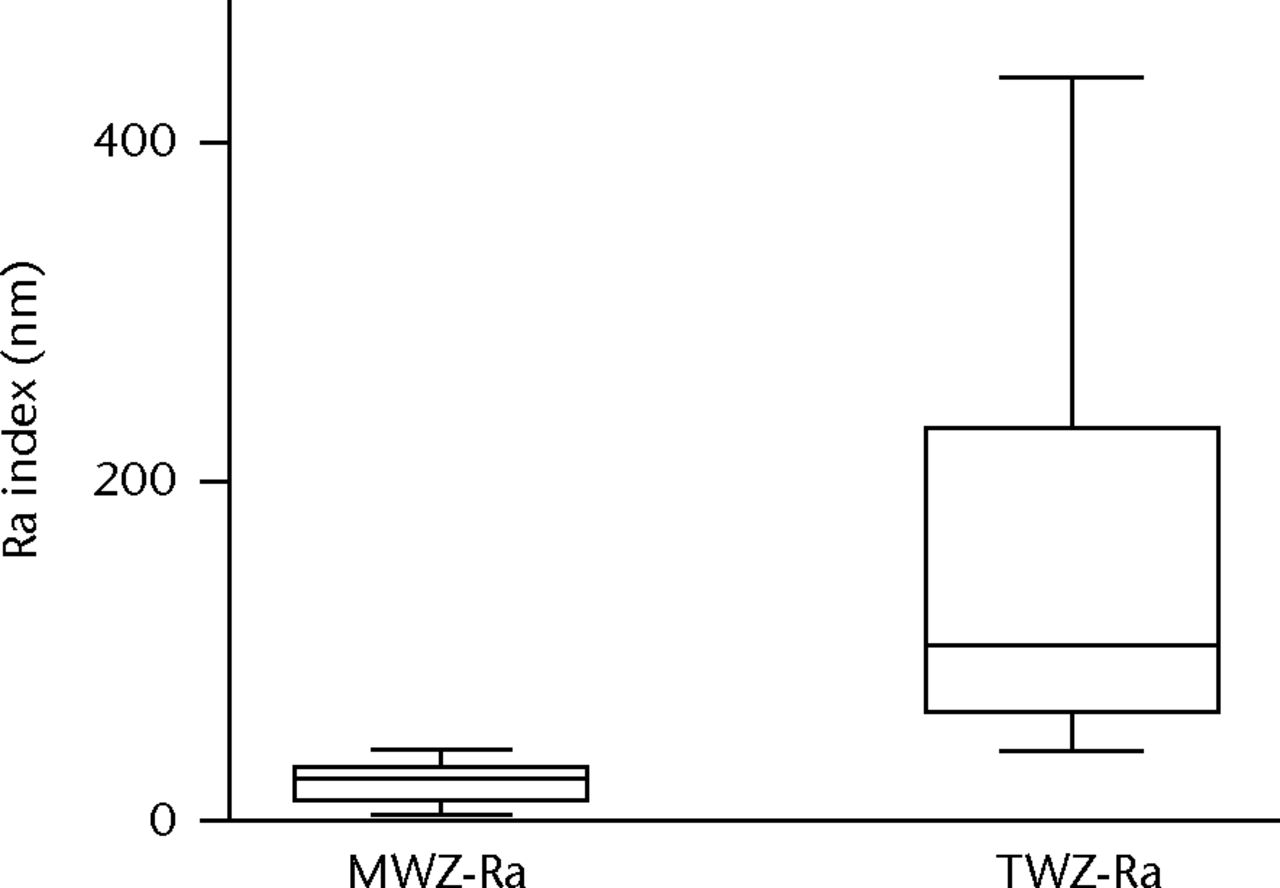
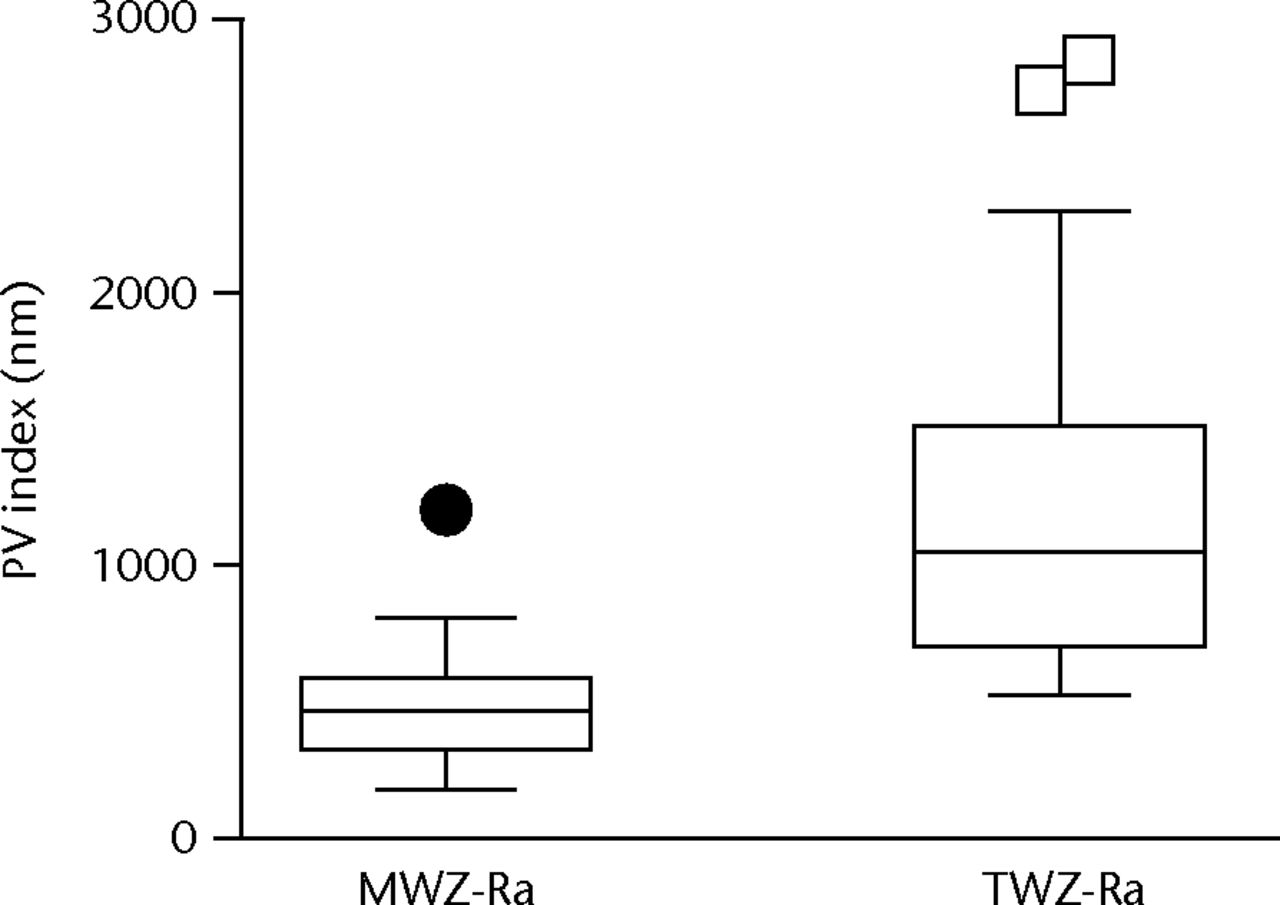
Figs. 5a - 5b
Graphs showing roughness index measured by the main wear zone roughness (MWZ-Ra) and total inclusion roughness (TWZ-Ra). Methods in cobalt–chrome (CoCr) particulate challenge (at 5 Mc) were statistically significantly different (p < 0.0001) with regard to a) roughness (Ra) and b) peak to valley depth (PV).
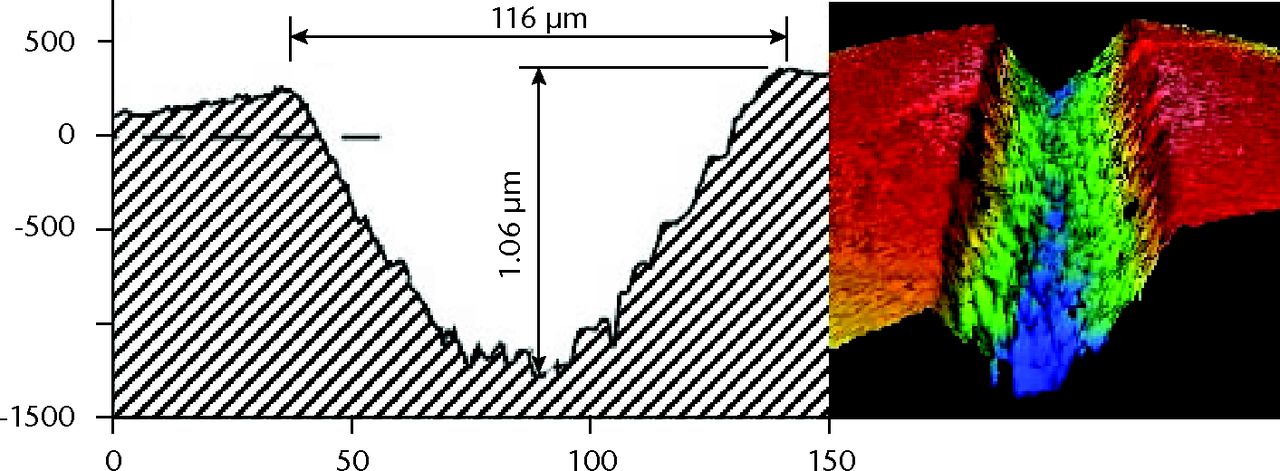
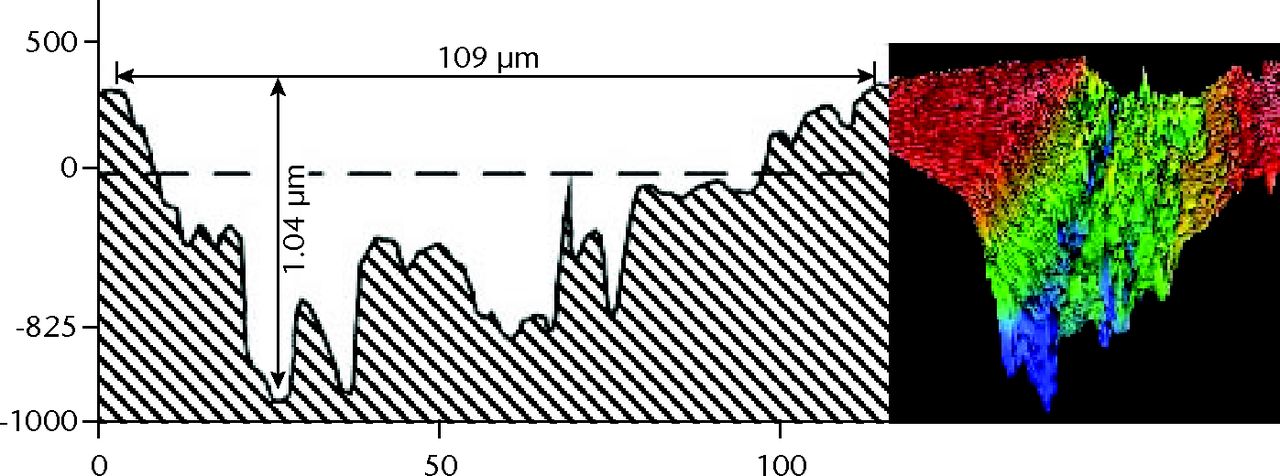
Figs. 6a - 6b
Graphs showing scratch profiles that were compared by interferometry for heads challenged by a) cobalt–chrome and b) titanium alloy debris.
Table V
Comparison of the main wear zone roughness (MWZ-Ra) and total inclusion roughness (TWZ-Ra) methods in three debris groups (roughness (Ra) and peak to valley depth (PV) indices, n = 36)
Methods | PMMA Ra (nm) | CoCr Ra (nm) | Ti6AI4V Ra (nm) | PMMA PV (nm) | CoCr PV (nm) | Ti6AI4V PV (nm) |
---|---|---|---|---|---|---|
MWZ-Ra | 11 | Â Â 21 | Â Â 16 | 389 | Â Â 430 | Â Â 450 |
TWZ-Ra | 13 | 145 | 159 | 329 | 1219 | 1401 |
Ratio | Â Â 1.2 | Â Â Â Â 6.9 | Â Â Â Â 9.9 | Â Â Â Â 0.8 | Â Â Â Â Â Â 2.8 | Â Â Â Â Â Â 3.1 |
-
PMMA, polymethylmethacrylate bone cement; CoCr, cobalt–chrome; Ti6Al4V, titanium alloy
Table VI
Scratch morphology profiled (n = 12) on one femoral head per debris group
Debris test | Scratch width | Valley depth | Lip height | Lip: valley ratio | Scratch depth | Aspect ratio |
---|---|---|---|---|---|---|
(W µm) | (V µm) | (P µm) | (PV µm) | (PV/W) | ||
PMMA | 3.9 | 0.055 | 0.021 | 0.38 | 0.08 | 0.019 |
(2.5 to 5.5) | (0.013 to 0.101) | (0.008 to 0.037) | ||||
CoCr | 78 | 0.848 | 0.211 | 0.25 | 1.06 | 0.014 |
(62 to 116) | (0.336 to 1.4) | (0.042 to 0.397) | ||||
Ti | 70 | 0.906 | 0.184 | 0.20 | 1.09 | 0.016 |
(37 to 115) | (0.267 to 1.8) | (0.065 to 0.535) | ||||
-
PMMA, polymethylmethacrylate bone cement; CoCr, cobalt–chrome alloy; Ti, titanium alloy; PV peak to valley depth
Discussion
This appears to be the first five million-cycle simulator study comparing the effects of abrasion of PMMA, CoCr and Ti6A4V contamination in MOM bearings. We propose that debris insertion at the beginning of each test interval represented one impingement episode,1 which was followed with 500 000 gait cycles for the MOM wear assessment. PMMA particulates have been used for some time as simulator test slurries.19,20,29 and were included here as controls. As anticipated, MOM rates of wear remained low. PMMA particulates and the bearings revealed minimal roughness changes, thus supporting our first hypothesis. In contrast, insertion of metal debris into MOM bearings had an adverse effect over each 500Â 000-cycle test interval. Metal particulates turned all lubricants black within the first hour of the test and produced rates of wear that were an order of magnitude higher than the controls. This supported the second hypothesis, indicating that wear in MOM bearings were very sensitive to the presence of metal debris.
There are many limitations in simulating abrasive wear mechanisms in vivo. It is impossible to predict the release of abrasive particulates in a patient’s hip joint.10,12,30 AÂ patient can accumulate 500 000 cycles of gait within a few months of daily activities, but the number of impingement events is not known. Some patients may sublux or impinge their hip joint at every step, while others may do this occasionally or not at all. We can only speculate broadly on debris production in vivo. In addition the size, shape and decomposition-processes of metal particles in vivo are virtually unknown. The contrast here is that studies of both MOM retrievals31 and simulator samples32 have typically described CoCr particles that were predominantly < 50 nm median size. Our debris profiling study26 showed that even the 100 µm to 400 µm sized metal particles were pulverised within ten seconds of the start of the test in the hip simulator study. The decomposition of one 100 µm sized CoCr fragment to the 0.05 µm median size would produce 8000 million particles. The life history of such particles (100 µm to 50 nm in size) in regard to decomposition under compressive and shear stresses, with resulting corrosion–dissolution into ionic form, appear to be additional ‘unknowns’.
Particle size was addressed by our retrieval studies that showed surface scratches of between 40 µm and 100 µm wide.9,23,25 These observations indicated that the abrading particles had to be at least of similar size (up to 100Â µm wide).26 However, there is no consensus in the literature and, therefore, future studies may wish to investigate different sizes of particles. In the patient, the debris may readily escape from the joint, whereas in the simulator, all debris was retained in the test chambers for 500Â 000 test cycles. It is also unknown whether CoCr beads or Ti6Al4V chips would be representative of debris produced in vivo26 or whether the introduction of 200 to 300 metal particles to MOM bearings at one time had clinical relevance. Could this test that turned lubricants black be considered unduly severe? It is noted that a common finding in contemporary MOM revisions has been black-coloured, peri-implant tissues, with MOM rates of wear reaching up to 70 mm3 per year.2,4,33 Thus, simulator rates of wear of 3 mm3 to 7 mm3 per million cycles in this study may be a fair representation of a mild to moderate clinical risk. In this regard, simulator studies with clean lubricants have produced rates of wear typically ranging from 0.5 mm3 to 0.8 mm3 per million cycles.34 The fact that blackened lubricants were conspicuous in this study with MOM rates of wear as low as 3.1 mm3 per million cycles, indicated that the margin of safety may be very small.
The PMMA challenge produced low MOM rates of wear and fine scratches that likely represented ‘self-polishing’ by surface carbides. It was particularly interesting that PMMA particles produced rates of wear similar to that of the simulator study using ceramic particles (MOM HA-challenge = 0.2 mm3/Mc).22 HA as a ceramic particle is reputed to have extreme hardness22 and conventional teaching suggests that the greater the hardness, the greater the damage.16,17 However, many factors may be involved, including the number of particles, debris circulation (ingress/escape ratios),12 and rate of decomposition, to name a few.
The Ti6Al4V particle challenge produced the highest MOM rates of wear and consistently turned lubricants black, thus supporting ourthird hypothesis. The earlier Ti-metal debris study8 produced a max-to-min wear ratio of × 8.2 (Fig. 1). Analysis of rates of wear in our Ti6Al4V debris study showed a max-to-min wear ratio of × 1.3 with particularly high linear-regression coefficients (Table VI: R > 0.98). This third-body abrasion study therefore presented a stable trend of wear over the standard five million-cycle test duration, and may offer a suitable model for future scientific studies.
It is to be noted that smearing Ti6Al4V across bearing surfaces has been a common finding in both MPE and ceramic-on-ceramic retrievals with case histories of impingement and dislocation.2,34-38 In this MOM simulator study, islands of Ti6Al4V found contaminating the MOM surfaces were identical to those demonstrated in our ten-cycle scratch-profile study.26 While it is unknown how tenacious these titanium coatings are in vivo,35,36 they were clearly present on 38 mm MOM bearings after 500 000 gait cycles, thus confirming our third hypothesis.
It was notable that the surface roughness following the CoCr and Ti6Al4V debris challenges appeared similar. However, these data were taken 500 000 cycles after debris insertion. We could have investigated a more transient wear effect, e.g. using 1000 or 10 000 cycle intervals, but chose to remain consistent to the original test protocol. The dual ability of Ti6Al4V particles to both scratch and smear on CoCr surfaces appeared to be the most aggressive mechanism of wear. The Ti6Al4V challenge elevated the average roughness indexes (TWZ-Ra) almost tenfold, a most significant change. This may explain the diversity in the prior simulator study that used the softer titanium (pure) metal as debris (Fig. 1) almost tenfold, a most significant change. This may explain the diversity in the prior simulator study that used the softer titanium (pure) metal as debris (Fig. 1) metal as debris (Fig. 1).
In the MOM retrieval study by Howie et al,1 it was proposedthata cascade of three events was necessary to trigger adverse wear, i.e. hip impingement or subluxation events that produced metal debris, ingress of metal debris between CoCr bearings, entrapment and displacement of the metal debris with the hip loading and movement producing a third-body abrasive wear. This was a study of cemented McKee–Farrar THA that featured non-modular stem and cup designs.1 It is to be noted that there are alternative sources of metallic particles in contemporary THA, including fretting with porous coatings adjacent to bone, fretting between fixation screws and metal implants, and mechanically-assisted corrosion at taper junctions.The additional metal debris would also be able to circulate the hip joint during subsequent activities of daily living. Such a patient-specific, abrasive wear mechanism would help explain why apparently identical McKee–Farrar procedures could perform well for > Â 20Â years in some cases39-42 and yet fail at < eight years in others, due to ARMD.1,43,44
This is the first MOM simulator study to investigate abrasive wear conditions created by metal particles and using bone cement as the control particles. The governing hypothesis was that the metal rim of an acetabular cup would damage the femoral neck during an impingement episode, thereby releasing large fragments of metal. Our simulator model assumed one THA impingement event followed by 500 000 cycles of gait, this being repeated ten times over five million cycles of study. The insertion of bone cement particles did not elevate MOM rates of wear above normal. In contrast, the insertion of CoCr and Ti6Al4V particles elevated rates of wear 11 and 17 times higher, respectively. These data indicated that MOM bearings were particularly sensitive to abrasive wear by metal particles. This may represent a clinically-relevant test mode as impingement is known to be the nemesis of THA devices.
1 Howie DW , McCaldenRW, NawanaNS, et al.The long-term wear of retrieved McKee-Farrar metal-on-metal total hip prostheses. J Arthroplasty2005;20:350–357.CrossrefPubMed Google Scholar
2 Langton DJ , JamesonSS, JoyceTJ, et al.Early failure of metal-on-metal bearings in hip resurfacing and large-diameter total hip replacement: A consequence of excess wear. J Bone Joint Surg [Br]2010;92-B:38–46.CrossrefPubMed Google Scholar
3 Langton DJ , JoyceTJ, JamesonSS, et al.Adverse reaction to metal debris following hip resurfacing: the influence of component type, orientation and volumetric wear. J Bone Joint Surg [Br]2011;93-B:164–171.CrossrefPubMed Google Scholar
4 Morlock MM , BishopN, ZustinJ, et al.Modes of implant failure after hip resurfacing: morphological and wear analysis of 267 retrieval specimens. J Bone Joint Surg [Am]2008;90-A(Suppl 3):89–95.CrossrefPubMed Google Scholar
5 Kwon YM , MellonSJ, MonkP, MurrayDW, GillHS. In vivo evaluation of edge-loading in metal-on-metal hip resurfacing patients with pseudotumours. Bone Joint Res2012;1:42–49.CrossrefPubMed Google Scholar
6 Hart AJ , SabahS, HenckelJ, et al.The painful metal-on-metal hip resurfacing. J Bone Joint Surg [Br]2009;91-B:738–744.CrossrefPubMed Google Scholar
7 Underwood RJ , ZografosA, SaylesRS, HartA, CannP. Edge loading in metal-on-metal hips: low clearance is a new risk factor. Proc Inst Mech Eng H2012;226:217–226.CrossrefPubMed Google Scholar
8 Lu B, Marti A, McKellop H. Wear of a second-generation metal-on-metal hip replacement effect of third-body abrasive particles. Trans Sixth World Biomat Congr Kamuela, Hawaii, 2000:183. Google Scholar
9 Clarke IC, Donaldson TK, Burgett MD, et al. Normal and Adverse Wear Patterns Created In-vivo on MOM Surfaces - a retrieval study representing four vendors. ASTM International 2013. DOI: 10.1520/STP156020120049. Google Scholar
10 Clarke IC , LazennecJY, BrussonA, et al.Impingement and 3rd-body Wear Mechanisms with 28mm MOM - A trigger mechanism for adverse wear in CoCr bearings. Clin Orthop Relat Res2014;472:497–508. Google Scholar
11 McHugh D, Currier J, Kennedy F, Collier J, Van Citters D. Plastic Deformation from Edge Loading is common on Retrieved Metal-on-Metal Hips and Can Be Predicted With Finite Element Analysis. ASTM International 2013:DOI:10.1520/STP156020120046. Google Scholar
12 Heiner AD , LundbergHJ, BaerTE, et al.Effects of episodic subluxation events on third body ingress and embedment in the THA bearing surface. J Biomech2008;41:2090–2096.CrossrefPubMed Google Scholar
13 Kligman M , FurmanBD, PadgettDE, WrightTM. Impingement contributes to backside wear and screw-metallic shell fretting in modular acetabular cups. J Arthroplasty2007;22:258–264.CrossrefPubMed Google Scholar
14 Birman MV , NoblePC, CondittMA, LiS, MathisKB. Cracking and impingement in ultra-high-molecular-weight polyethylene acetabular liners. J Arthroplasty2005;20(Suppl3):87–92.CrossrefPubMed Google Scholar
15 Caravia L , DowsonD, FisherJ, JobbinsB. The influence of bone and bone cement debris on counterface roughness in sliding wear tests of ultra-high molecular weight polyethylene on stainless steel. Proc Inst Mech Eng H1990;204:65–70.CrossrefPubMed Google Scholar
16 Davidson JA , PoggieRA, MishraAK. Abrasive wear of ceramic, metal, and UHMWPE bearing surfaces from third-body bone, PMMA bone cement, and titanium debris. Biomed Mater Eng1994;4:213–229.PubMed Google Scholar
17 Que L , TopoleskiLD. Third-body wear of cobalt-chromium-molybdenum implant alloys initiated by bone and poly(methyl methacrylate) particles. J Biomed Mater Res2000;50:322–330.CrossrefPubMed Google Scholar
18 Bragdon CR , JastyM, MuratogluOK, O’ConnorDO, HarrisWH. Third-body wear of highly cross-linked polyethylene in a hip simulator. J Arthroplasty2003;18:553–561.CrossrefPubMed Google Scholar
19 Wang A , EssnerA. Three-body wear of UHMWPE acetabular cups by PMMA particles against CoCr, alumina and zirconia heads in a hip joint simulator. Wear2001;250:212–216. Google Scholar
20 Kubo K , ClarkeIC, WilliamsPA, et al.Aggressive 3rd-body wear challenge to highly crosslinked polyethylene: A hip simulator model. J Bone Joint Surg [Br]2010;92-B(SupplI):103. Google Scholar
21 Parikh A, Hill P, Pawar V, Sprague J. Hip simulator wear testing of modular diffusion hardened oxidized zirconium couples. [abstract] Orthopedic Research Society, 2012. Google Scholar
22 Liao Y-S, Swope S, Whitaker D, et al. Effects of 3rd body Hydroxyapatite (HA) Particles on the Wear Performance of 36 mm Ceramic-on-Metal (COM) and Metal-on-Metal (MOM) Hip Joint components in a Wear Simulation Study. [abstract] ORS 56th Annual meeting 2010:2325. Google Scholar
23 Bowsher JG , DonaldsonTK, WilliamsPA, ClarkeIC. Surface damage after multiple dislocations of a 38-mm-diameter, metal-on-metal hip prosthesis. J Arthroplasty2008;23:1090–1096.CrossrefPubMed Google Scholar
24 Kubo K , ClarkeIC, LazennecJY, et al.Wear Mapping analysis with retrieval 28mm CoCr-CoCr Hip Bearings – 11 years Experience. J Bone Joint Surg [Br]2010;92-B(Suppl):146. Google Scholar
25 Clarke IC , LazennecJY, BrussonA, et al.Risk of impingement and third-body abrasion with 28-mm metal-on-metal bearings. Clin Orthop Relat Res2014;472:497–508.CrossrefPubMed Google Scholar
26 Halim T , BurgettM, DonaldsonTK, et al.Profiling the third-body wear damage produced in CoCr surfaces by bone cement, CoCr, and Ti6Al4V debris: a 10-cycle metal-on-metal simulator test. Proc Inst Mech Eng H2014;228:703–713.CrossrefPubMed Google Scholar
27 Bowsher JG , ClarkeIC, WilliamsPA, DonaldsonTK. What is a “normal” wear pattern for metal-on-metal hip bearings?J Biomed Mater Res B Appl Biomater2009;91:297–308. Google Scholar
28 No authors listed. Implants for surgery - wear of total hip-joint prostheses - Part 3: Loading and displacement parameters for orbital bearing type wear testing machines and corresponding environmental conditions for test. ISO/CD. http://www.iso.org/iso/home.htm (date last accessed 3 February 2015). Google Scholar
29 Bragdon CR , JastyM, MuratogluOK, HarrisWH. Third-body wear testing of a highly cross-linked acetabular liner: the effect of large femoral head size in the presence of particulate poly(methyl-methacrylate) debris. J Arthroplasty2005;20:379–385.CrossrefPubMed Google Scholar
30 Onda K , NagoyaS, KayaM, YamashitaT. Cup-neck impingement due to the malposition of the implant as a possible mechanism for metallosis in metal-on-metal total hip arthroplasty. Orthopedics2008;31:396.CrossrefPubMed Google Scholar
31 Doorn PF , CampbellPA, WorrallJ, et al.Metal wear particle characterization from metal on metal total hip replacements: transmission electron microscopy study of periprosthetic tissues and isolated particles. J Biomed Mater Res1998;42:103–111.CrossrefPubMed Google Scholar
32 Leslie I , WilliamsS, BrownC, et al.Effect of bearing size on the long-term wear, wear debris, and ion levels of large diameter metal-on-metal hip replacements-An in vitro study. J Biomed Mater Res B Appl Biomater2008;87:163–172.CrossrefPubMed Google Scholar
33 Witzleb WC , HanischU, ZieglerJ, GuentherKP. In vivo wear rate of the Birmingham Hip Resurfacing arthroplasty. A review of 10 retrieved components. J Arthroplasty2009;24:951–956.CrossrefPubMed Google Scholar
34 Clarke IC , DonaldsonT, BowsherJG, NasserS, TakahashiT. Current concepts of metal-on-metal hip resurfacing. Orthop Clin North Am2005;36:143–162.CrossrefPubMed Google Scholar
35 Eberhardt AW , McKeeRT, CucklerJM, et al.Surface roughness of CoCr and ZrO2 femoral heads with metal transfer: A retrieval and wear simulator study. Int J Biomater2009;2009:185456. Google Scholar
36 Müller FA , HagymásiM, GreilP, ZeilerG, SchuhA. Transfer of metallic debris after dislocation of ceramic femoral heads in hip prostheses. Arch Orthop Trauma Surg2006;126:174–180.CrossrefPubMed Google Scholar
37 Bal BS , RahamanMN, AletoT, et al.The significance of metal staining on alumina femoral heads in total hip arthroplasty. J Arthroplasty2007;22:14–19.CrossrefPubMed Google Scholar
38 Clarke IC , GreenDD, WilliamsPA, et al.Hip-simulator wear studies of an alumina-matrix composite (AMC) ceramic compared to retrieval studies of AMC balls with 1-7 years follow-up. Wear2009;267:702–709. Google Scholar
39 McKellep H , ParkSH, ChiesaR, et al.In vivo wear of three types of metal on metal hip prostheses during two decades of use. Clin Orthop Relat Res1996;329(Suppl):S128–140.CrossrefPubMed Google Scholar
40 Campbell P , UrbanRM, CatelasI, SkiporAK, SchmalzriedTP. Autopsy analysis thirty years after metal-on-metal total hip replacement. A case report. J Bone Joint Surg [Am]2003;85-A:2218–2222.CrossrefPubMed Google Scholar
41 Schmalzried TP , PetersPC, MaurerBT, BragdonCR, HarrisWH. Long-duration metal-on-metal total hip arthroplasties with low wear of the articulating surfaces. J Arthroplasty1996;11:322–331.CrossrefPubMed Google Scholar
42 Schmalzried TP , SzuszczewiczES, AkizukiKH, PetersenTD, AmstutzHC. Factors correlating with long term survival of McKee-Farrar total hip prostheses. Clin Orthop Relat Res1996;329(Suppl):S48–S59.CrossrefPubMed Google Scholar
43 Langton DJ , JamesonSS, JoyceTJ, et al.Accelerating failure rate of the ASR total hip replacement. J Bone Joint Surg [Br]2011;93-B:1011–1016.CrossrefPubMed Google Scholar
44 Jones DA , LucasHK, O'DriscollM, PriceCHG, WibberleyB. Cobalt Toxicity after McKee Hip Arthroplasty. J Bone Joint Surg [Br]1975;57-B:289–296.PubMed Google Scholar
Funding statement:
This MOM retrieval study was supported by an FDA contract ‘Development and Validation of Improved Premarket Testing methods (simulator studies) for Metal-on-Metal (MOM) Hip Replacements’ (FD-1090372). Thanks are due to the DJO Group (Austin, Texas) for donation of the MOM implants used in this study. Funds have also been received by the FDA from Critical Path Funding to assist with this study. I. C. Clarke has received funding from Stryker, WMT and Biomet Japan, none of which is related to this article.
Author contributions:
T. Halim: Simulator study, Micro-analyses, Data consolidation
I. C. Clarke: Design of study, Overview of analyses, Data consolidation, Manuscript production, Reviews
M. D. Burgett-Moreno: Simulator study, Micro-analyses, Data consolidation, Manuscript review
T. K. Donaldson: Consultant Orthopedic Surgeon, Clinical perspective, Paper reviews
C. Savisaar: Design of study, Overview of analyses, Paper reviews
J. G. Bowsher: Design of study, Overview of analyses, Paper reviews
ICMJE Conflict of Interest:
This study was federally funded (FDA) for open-access distribution
©2015 The British Editorial Society of Bone & Joint Surgery. This is an open-access article distributed under the terms of the Creative Commons Attributions licence, which permits unrestricted use, distribution, and reproduction in any medium, but not for commercial gain, provided the original author and source are credited.