Abstract
Objectives
Rotator cuff tears are among the most common and debilitating upper extremity injuries. Chronic cuff tears result in atrophy and an infiltration of fat into the muscle, a condition commonly referred to as ‘fatty degeneration’. While stem cell therapies hold promise for the treatment of cuff tears, a suitable immunodeficient animal model that could be used to study human or other xenograft-based therapies for the treatment of rotator cuff injuries had not previously been identified.
Methods
A full-thickness, massive supraspinatus and infraspinatus tear was induced in adult T-cell deficient rats. We hypothesised that, compared with controls, 28 days after inducing a tear we would observe a decrease in muscle force production, an accumulation of type IIB fibres, and an upregulation in the expression of genes involved with muscle atrophy, fibrosis and inflammation.
Results
Chronic cuff tears in nude rats resulted in a 30% to 40% decrease in muscle mass, a 23% reduction in production of muscle force, and an induction of genes that regulate atrophy, fibrosis, lipid accumulation, inflammation and macrophage recruitment. Marked large lipid droplet accumulation was also present.
Conclusions
The extent of degenerative changes in nude rats was similar to what was observed in T-cell competent rats. T cells may not play an important role in regulating muscle degeneration following chronic muscle unloading. The general similarities between nude and T-cell competent rats suggest the nude rat is likely an appropriate preclinical model for the study of xenografts that have the potential to enhance the treatment of chronically torn rotator cuff muscles.
Cite this article: Bone Joint Res 2014;3:262–72.
Article focus
To assess the role of T lymphocytes in the development of fatty degeneration following rotator cuff tear
To evaluate the viability of an immunodeficient rodent model that could be used to study xenograft-based therapies for the treatment of rotator cuff tears
Key messages
T lymphocytes are dispensable for the onset of lipid infiltration and muscle atrophy following rotator cuff tear
The NIH nude rat is an attractive model for the study of xenograft-based therapies for enhancing the treatment of rotator cuff tears
Strengths and limitations
Strength: Detailed analysis of changes in muscle contractility, muscle fibre architecture, lipid infiltration, and macrophage infiltration in a rodent model of rotator cuff tear.
Limitation: We only measured at one time point of one month and did not evaluate changes at other time points
Introduction
Rotator cuff tears are among the most frequent and debilitating upper extremity injuries, with over 250 000 surgical repairs performed each year in the United States.1 While there have been notable improvements in surgical repair and rehabilitation techniques, many patients continue to have symptoms after repair, and re-tear rates for surgical repair of full-thickness tears remain unacceptably high.2 Tears of the supraspinatus are most common, followed by the infraspinatus tendon, and less involved are the subscapularis and teres minor tendons.3 A set of common pathological changes occur in torn rotator cuff muscles including atrophy of muscle fibres, fibrosis, and an accumulation of lipid within and around muscle fibres.3 These changes are commonly referred to as ‘fatty degeneration’ and despite successful surgical repair of the tear, fatty degeneration often does not improve after repair and correlates with poor functional outcomes.4 The cellular and molecular aetiology of fatty degeneration is not fully understood, and gaining greater insight into the physiological processes that regulate muscle fibre atrophy, fibrosis and lipid accumulation will likely improve the treatment of patients with chronic rotator cuff tears.
The immune system plays an important role in the regeneration of muscle after injury.5 Macrophages, which arise from monocytes produced in bone marrow, are the most well studied members of the leukocyte family that participate in muscle regeneration.5 There are two general classes of macrophages that participate in muscle regeneration. After muscle injury, pro-inflammatory M1 macrophages rapidly enter muscle tissue and participate in phagocytosis of muscle debris, and also secrete cytokines and produce nitric oxide, which promote muscle degeneration.6 Anti-inflammatory M2 macrophages have a slower entry into injured muscle, and secrete anti-inflammatory cytokines that attenuate M1 macrophages and can promote muscle fibre regeneration.6 T lymphocytes, which arise from haematopoietic stem cells in the bone marrow and mature in the thymus, are thought to play an important role in coordinating the inflammation and regeneration of muscle after injury, through direct interactions with muscle fibres, as well as indirectly through the regulation of M1 and M2 macrophages.7-9 T lymphocytes are also recruited into adipose tissue in obese individuals and contribute to obesity-related increases in local and systemic inflammation through direct interactions with adipocytes or via the recruitment of M1 macrophages.10 A similar response has been observed in atherosclerotic plaques, where T lymphocytes directly promote local inflammation and lipid accumulation through the secretion of pro-inflammatory cytokines and the activation of M1 macrophages.9 Additionally, diseases that result in T cell depletion, like HIV, may increase the susceptibility to developing a rotator cuff tear.11 While T lymphocytes have a clear role in promoting inflammation and lipid accumulation in several tissues, the role that these cells play in orchestrating the response of the rotator cuff to a tear was not known. To address the role of T lymphocytes in fatty degeneration of a rotator cuff, we used the NIH nude rat (NIH-Foxn1rnu). This outbred line of rats has a naturally occurring mutation in the Foxn1 gene, which results in the failure to form a mature thymus and subsequent lack of mature T lymphocytes, although monocytes and B cells are still present.12-14
There has been much interest in the use of stem cell therapy to augment the healing of torn rotator cuff muscles.15 Mesenchymal stem cells have the ability to differentiate into multiple cell types that may be helpful for the treatment of chronic rotator cuff tears, including skeletal muscle, tendon, cartilage and bone.16 While animal studies using autologous cells can be informative, evaluating human derived stem cells in animal models of injury and disease is also important in identifying safe and effective stem cell therapies.17 The lack of T lymphocytes allows nude rats to accept xenografts and to potentially serve as models to study cell-based therapies in specific injuries and disease states.14
To gain a greater understanding of the role that T lymphocytes play in fatty degeneration of a rotator cuff, and to evaluate the suitability of an immune deficient rodent model that could be used to study xenograft-based therapies for the treatment of rotator cuff injuries, we used a well established chronic experimental technique of a full-thickness rotator cuff tear18-20 in a group of adult NIH nude rats. We determined changes in muscle fibre contractility, type of muscle fibre, distribution and size, and the expression of messenger RNA (mRNA) and microRNAs (miRNAs) involved in muscle atrophy, inflammation, lipid synthesis and storage, extracellular matrix synthesis and fibrosis, and macrophage accumulation in nude rats that underwent a full-thickness supraspinatus and infraspinatus tear. We tested the hypothesis that 28 days following a rotator cuff tear in nude rats, there would be a reduction in muscle-specific fibre force production and an induction in the expression of mRNA and miRNA transcripts that regulate atrophy, autophagy, inflammation, lipid accumulation and fibrosis.
Materials and Methods
Animals
This study was approved by the University of Michigan Committee for the Use and Care of Animals. Four-month-old male athymic nude rats (NIH-Foxn1rnu) (n = 5) were obtained from Charles River Laboratories (Wilmington, Massachusetts). We selected this sample size based upon a power analysis of specific force data from a previous study.18 Rats were housed in specific pathogen-free conditions and provided food and water ad libidum. Animal handling and care was performed in accordance with the NIH Policy on Humane Care and Use of Laboratory Animals.
Surgeries
Full-thickness tears of the right supraspinatus and infraspinatus were performed as previously described,18,21 with the left supraspinatus and infraspinatus muscles serving as sham-operated, intact controls. We chose these muscles, rather than the subscapularis and teres minor, due to the higher frequency of injury in humans.3 The skin around the shoulder was thoroughly scrubbed with ChloraPrep (CareFusion), and a full-thickness tenectomy of the right supraspinatus and infraspinatus tendons was performed through a deltoid-splitting transacromial approach. This technique simulates a massive rotator cuff tear and prevents scarring and healing of detached tendons that can occur in rodent models.19 The left shoulder served as a sham-operated control in which a deltoid-splitting surgery was performed, but the rotator cuff tendons were left intact. A splash block of 0.5% bupivicaine with 1:100 000 epinephrine was administered. The deltoid was closed using an absorbable 5-0 chromic gut suture (Johnson and Johnson, New Brunswick, New Jersey) and the skin was closed using 5-0 nylon (Johnson and Johnson) and GLUture (Abbott Laboratories, Abbott Park, Illinois). Following surgery, a single dose of ampicillin (20 mg/kg, subcutaneous) and carprofen (5 mg/kg, subcutaneous) was administered. Buprenorphine (0.05 mg/kg) was administered for analgesia during the post-operative period. Free cage activity and weight-bearing were allowed, and rats were closely monitored for signs of distress or infection. A total of 28 days after surgery, rats were anaesthetised with sodium pentobarbital (50 mg/kg), and the supraspinatus and infraspinatus muscles were harvested. The distal ends of all muscles were free and no signs of lateral adhesion formation were present. Rats were then humanely euthanised with pentobarbital overdose and induction of pneumothorax. Supraspinatus muscles were weighed, finely minced, and immediately prepared for RNA isolation. Infraspinatus muscles were weighed, and separated at the mid-belly for histology and single fibre contractility.
Histology and immunohistochemistry
Distal segments of the infraspinatus were frozen in Tissue-Tek (Sakura, Torrance, California) using isopentane cooled liquid nitrogen, and stored at -80ºC until use. Muscles were cryosectioned at a thickness of 10 µm, and prepared for staining with Oil red O and haematoxylin or prepared for immunohistochemistry (IHC).17,18 Distribution of fibre type was determined by labeling sections with antibodies against myosin heavy chain type I (DSHB, Iowa City, Iowa), myosin heavy chain type IIA (DSHB) and myosin heavy chain type IIB (DSHB). The extracellular matrix (ECM) was identified using wheat germ agglutinin (WGA) lectin conjugated to AlexaFluor 488 (Invitrogen, Grand Island, New York). Type IIX fibres were detected by the absence of fluorescent signal. To identify macrophages and areas of lipid accumulation, slides were incubated with antibodies against the macrophage surface antigen F4/80 (AbCam, Cambridge, Massachusetts) and the neutral lipid stain BODIPY 493/503 (Invitrogen). Primary antibodies were detected using highly cross-adsorbed secondary antibodies conjugated to AlexaFluor fluorescent probes (Invitrogen). Images were obtained using an Axioplan 2 (Zeiss, Jena, Germany) microscope equipped with AxioCam (Zeiss) cameras. Quantitative histomorphometry was performed using ImageJ software (NIH, Bethesda, Maryland).
Muscle fibre contractility
Contractile measurements of permeabilised single muscle fibres from the proximal infraspinatus were performed as described.18,22 A total of ten to 20 type II fibres were tested from each infraspinatus muscle.
Gene expression
Samples of supraspinatus muscle were homogenised in Qiazol (Qiagen, Valencia, California) and separated using chloroform. Total RNA was further purified from the aqueous phase of the homogenised solution using a miRNeasy kit (Qiagen). DNAse I (Qiagen) was used to eliminate genomic DNA. The concentration of isolated RNA was measured on a NanoDrop (Thermo Scientific, Wilmington, Delaware). RNA (500 ng per sample) was reverse transcribed to cDNA using the RT2 First strand kit (Qiagen) according to the protocol of the manufacturer. RT2 SYBR Green qPCR mix was used to amplify cDNA along with a custom array of primers for specific mRNA and miRNA molecules (Qiagen) in a real-time thermal cycler (CFX96, Bio-Rad, Hercules, California). Expression of mRNA transcripts was normalised to the stable housekeeping gene β-actin, and miRNA transcripts to the stable housekeeping gene Rnu6 using the techniques of Schmittgen and Livak.23 The list of RNA transcripts and corresponding RefSeq and miRBase information is listed in Tables I and II.
Table I
Messenger RNA transcripts evaluated by qPCR
Gene | RefSeq | Name |
---|---|---|
Acat1 | NM_017075 | Acetyl-coenzyme A acetyltransferase 1 |
Actb | NM_031144 | Actin, beta |
Agpat6 | NM_001047849 | 1-acylglycerol-3-phosphate O-acyltransferase 6 |
Alox5 | NM_012822 | 5-LOX, Arachidonate 5-lipoxygenase |
Apoe | NM_138828 | Apolipoprotein E |
Atg16l1 | NM_001108809 | ATG16 autophagy related 16-like 1 (S. cerevisiae) |
Atg5 | NM_001014250 | ATG5 autophagy related 5 homolog (S. cerevisiae) |
Becn1 | NM_053739 | Beclin 1, autophagy related |
Cd11b | NM_012711 | Integrin, alpha M |
Cd68 | NM_001031638 | Cd68 molecule |
Cebpa | NM_012524 | CCAAT/enhancer binding protein (C/EBP), alpha |
Cgi58 | NM_212524 | Abhydrolase domain containing 5 |
Col1a2 | NM_053356 | Collagen, type I, alpha 2 |
Cox1 | NM_017043 | Prostaglandin-endoperoxide synthase 1 |
Cox2 | NM_017232 | Prostaglandin-endoperoxide synthase 2 |
Dgat1 | NM_053437 | Diacylglycerol O-acyltransferase homolog 1 (mouse) |
Emr1 | NM_001007557 | EGF-like module containing, mucin-like, hormone receptor-like 1 |
Fbxo32 | NM_133521 | Atrogin-1 |
Fitm1 | NM_001106037 | Fat storage-inducing transmembrane protein 1 |
Fitm2 | NM_001107799 | Fat storage-inducing transmembrane protein 2 |
Fsp1 | NM_012618 | S100 calcium-binding protein A4 |
Fsp27 | NM_001024333 | Cell death-inducing DFFA-like effector c |
Cd168 | NM_012964 | Hyaluronan mediated motility receptor (RHAMM) |
Il10 | NM_012854 | Interleukin 10 |
Il1b | NM_031512 | Interleukin 1 beta |
Il6 | NM_012589 | Interleukin 6 |
Mmp14 | NM_031056 | Matrix metallopeptidase 14 (membrane-inserted) |
Mmp2 | NM_031054 | Matrix metallopeptidase 2 |
Mmp9 | NM_031055 | Matrix metallopeptidase 9 |
eMHC | NM_012604 | Myosin, heavy chain 3, skeletal muscle, embryonic |
Pdgfra | NM_012802 | Platelet derived growth factor receptor, alpha polypeptide |
Pld1 | NM_030992 | Phospholipase D1 |
Plin | NM_013094 | Perilipin 1 |
Plin2 | NM_001007144 | Perilipin 2, ADRP, ADFP, Adiophilin |
Plin5 | NM_001134637 | Perilipin 5 |
Atgl | NM_001108509 | Adipose triglyceride lipase |
Pparg | NM_013124 | Peroxisome proliferator-activated receptor gamma |
Fat/Cd36 | XM_575338 | Similar to fatty acid translocase/CD36 |
Scx | NM_001130508 | Scleraxis |
Timp1 | NM_053819 | TIMP metallopeptidase inhibitor 1 |
Timp2 | NM_021989 | TIMP metallopeptidase inhibitor 2 |
Tnmd | NM_022290 | Tenomodulin |
Trim63 | NM_080903 | MuRF-1, tripartite motif-containing 63 |
Vps34 | NM_022958 | Phosphoinositide-3-kinase, class 3 |
Table II
microRNA transcripts evaluated by qPCR.
miRNA | miRBase Accession ID |
---|---|
rno-miR-1 | MIMAT0003125 |
rno-miR-103 | MIMAT0000824 |
rno-miR-107 | MIMAT0000826 |
rno-miR-126 | MIMAT0000832 |
rno-mir-130a | MI0000903 |
rno-miR-133a | MIMAT0000839 |
rno-miR-133b | MIMAT0003126 |
rno-miR-138 | MIMAT0000844 |
rno-miR-143 | MIMAT0000849 |
rno-miR-196a | MIMAT0000871 |
rno-miR-206 | MIMAT0000879 |
rno-miR-21 | MIMAT0000790 |
rno-miR-214 | MIMAT0000885 |
rno-miR-221 | MIMAT0000890 |
rno-miR-222 | MIMAT0000891 |
rno-mir-23a | MIMAT0000792 |
rno-miR-23b | MIMAT0000793 |
rno-mir-27a | MIMAT0000799 |
rno-miR-29b | MIMAT0000801 |
rno-miR-31 | MIMAT0000810 |
rno-miR-338 | MIMAT0000581 |
rno-miR-381 | MIMAT0003199 |
rno-miR-499 | MIMAT0003381 |
rno-let-7a | MIMAT0000774 |
rno-let-7b | MIMAT0000775 |
rno-let-7c | MIMAT0000776 |
rno-let-7d | MIMAT0000562 |
rno-let-7e | MIMAT0000777 |
Statistical analysis
Data are presented as mean and standard error of the mean (sem). Differences between the sham-operated shoulder and the shoulder with a rotator cuff tear were tested using Student’s t-tests (α = 0.05) in GraphPad Prism 6.0 (GraphPad, La Jolla, California).
Results
A total of 28 days after inducing a full-thickness rotator cuff tear, there was a 33% decrease in the mass of supraspinatus muscles (sham 417 mg; sem 20.3, tear 279 mg; sem 20.5, p < 0.05) and a 40% decrease in the mass of infraspinatus muscles (sham 394 mg; sem 19.7, tear 235 mg; sem 12.9 , p < 0.05). For single muscle contractility measurements, a rotator cuff tear did not affect muscle fibre CSA, but a 16% decrease in Fo and a 23% decrease in sFo was observed (Fig. 1). A rotator cuff tear increased the size and accumulation of type IIB muscle fibres by 30% and 18%, respectively, and decreased the amount of type I and IIA muscle fibres by approximately 10% (Fig. 2).
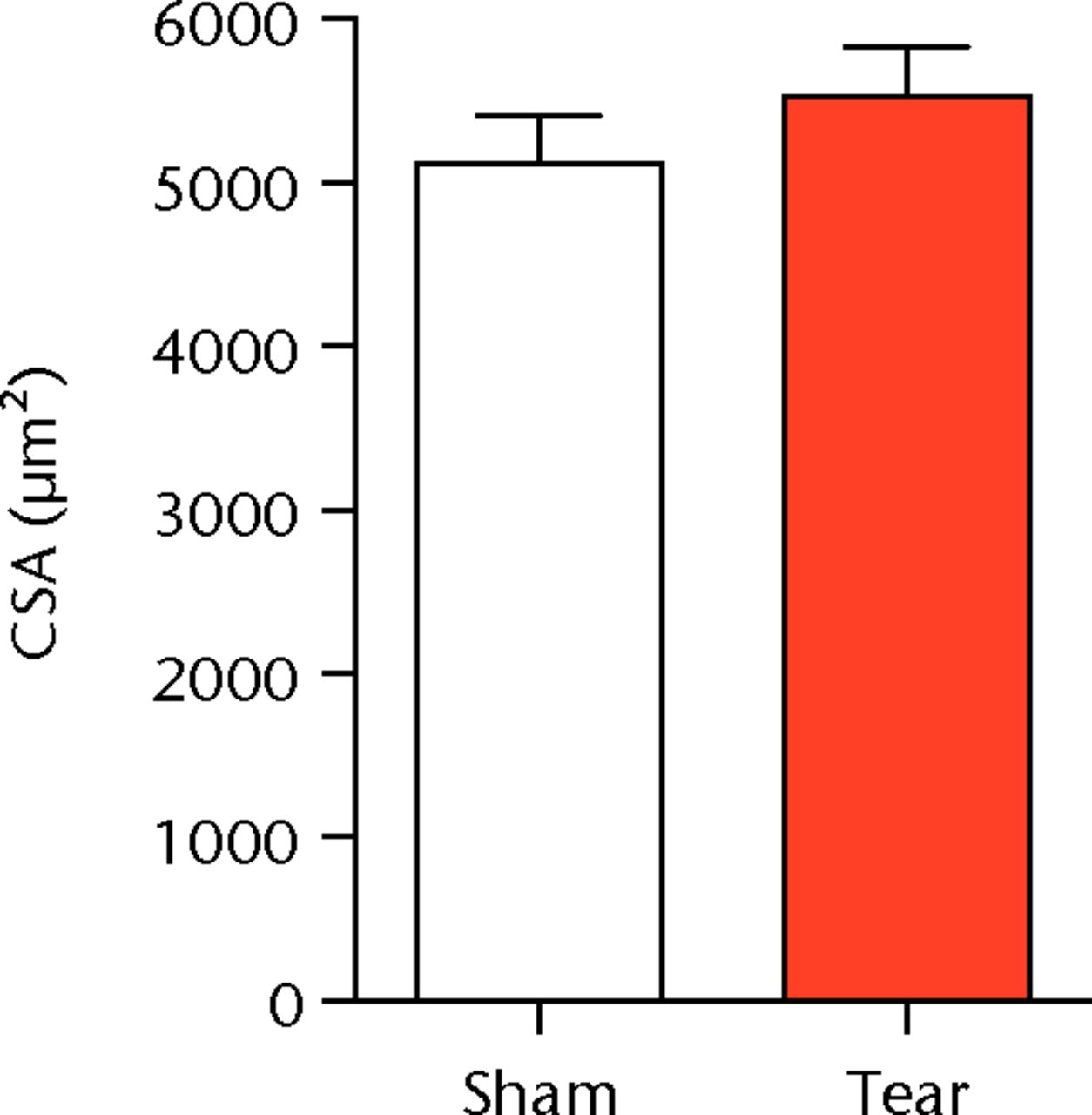
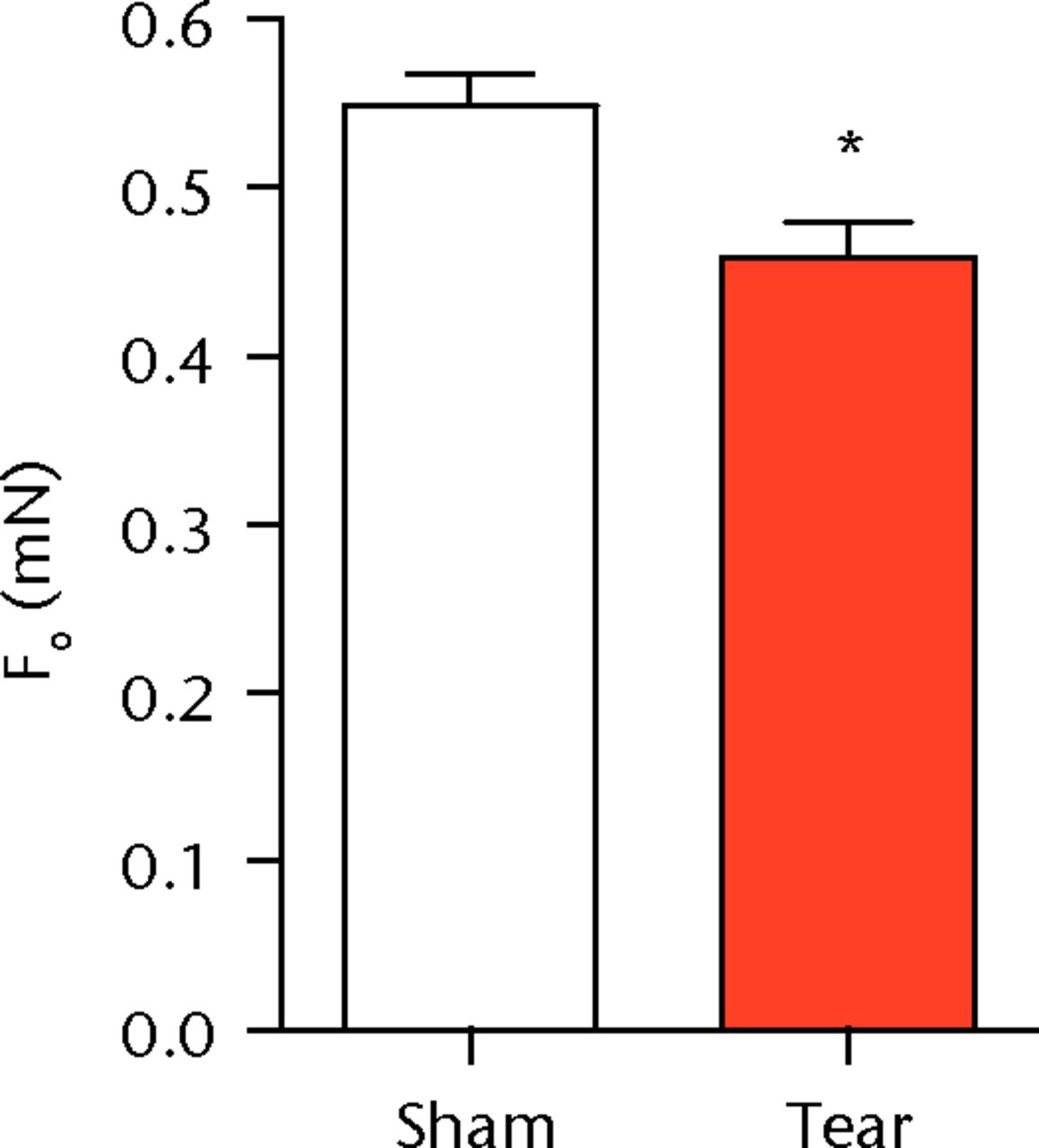
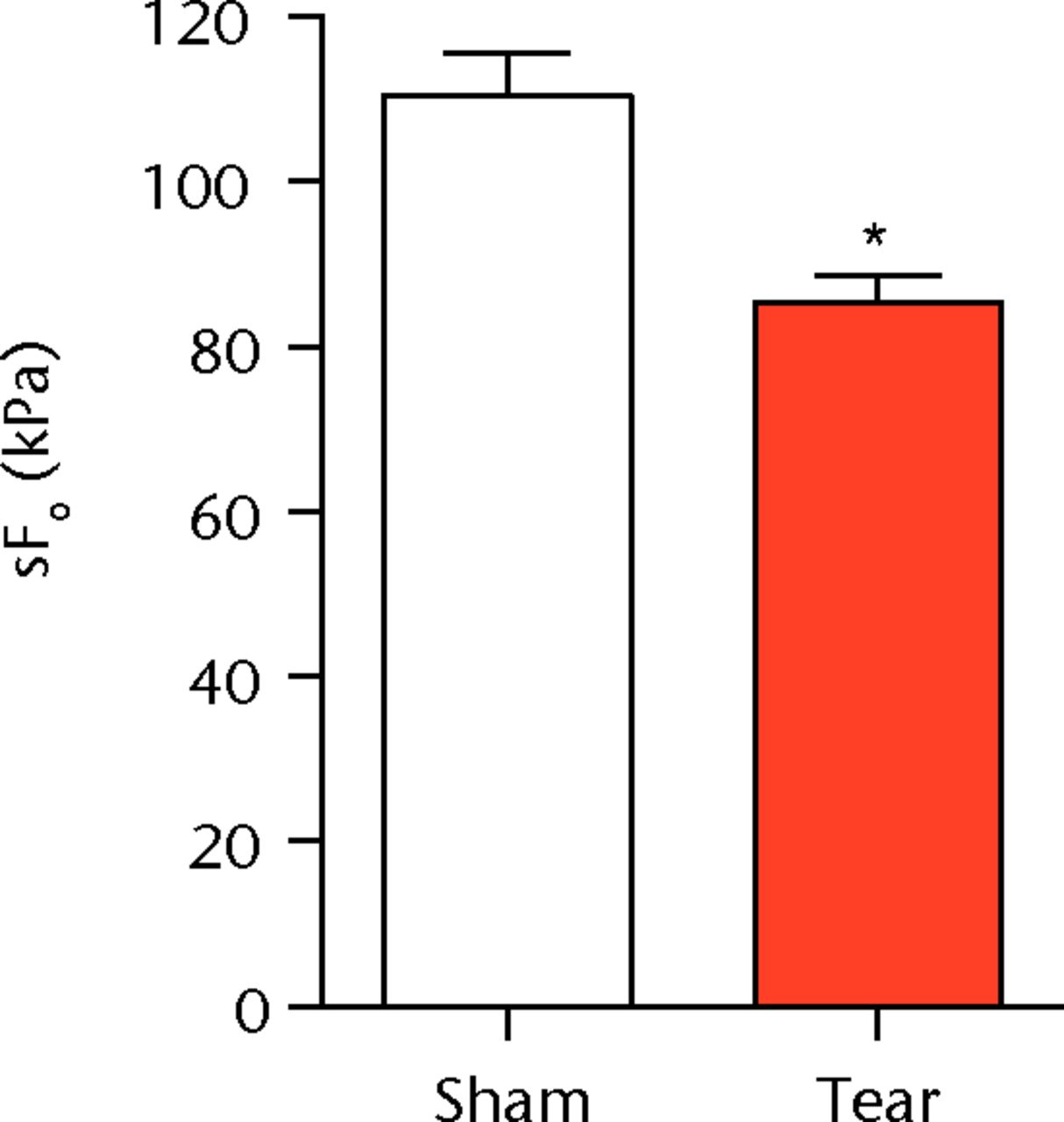
Figs. 1a - 1c
Graphs showing permeabilised muscle fibre size and contractile force. Figure 1a - Permeabilised fibre cross-sectional area (CSA); Figure 1b - maximum isometric force (Fo) and figure 1c - specific force (sFo) of sham-operated control and torn rotator cuff muscles. Values are mean and sem. n = 5 muscles from each group. * different from sham-operated control group (p < 0.05).
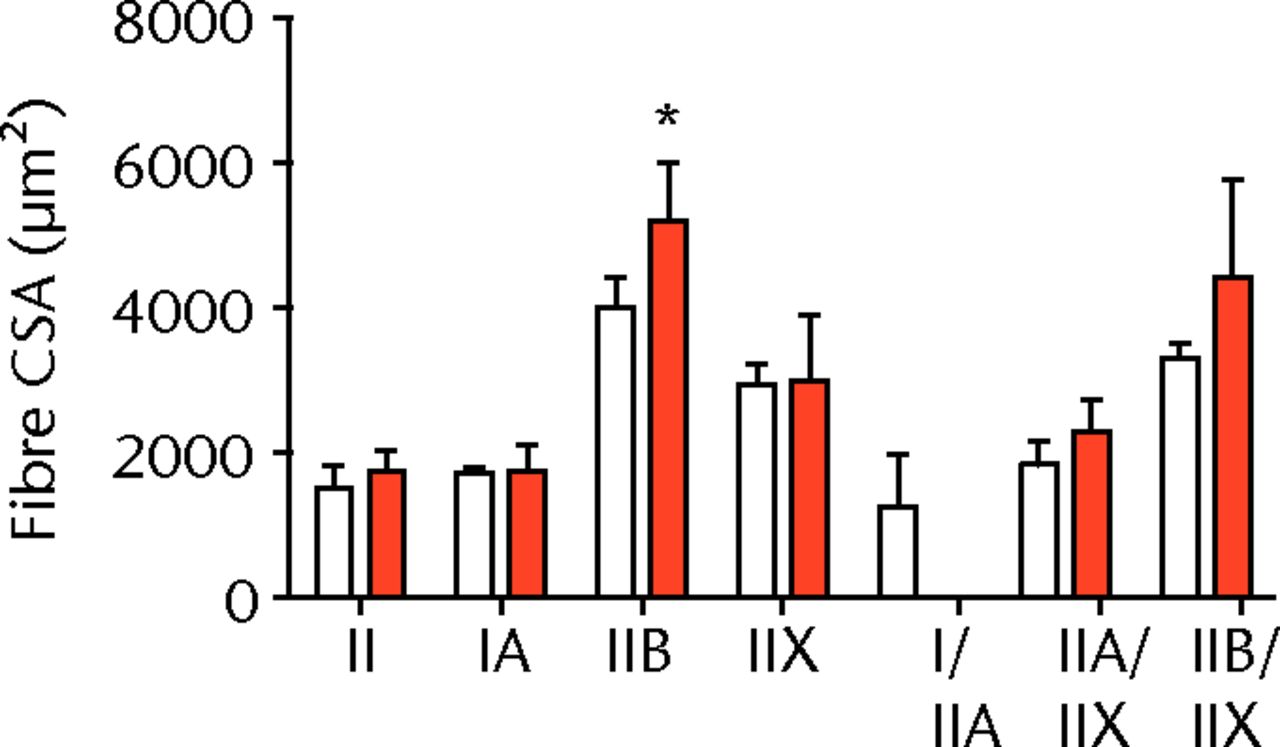
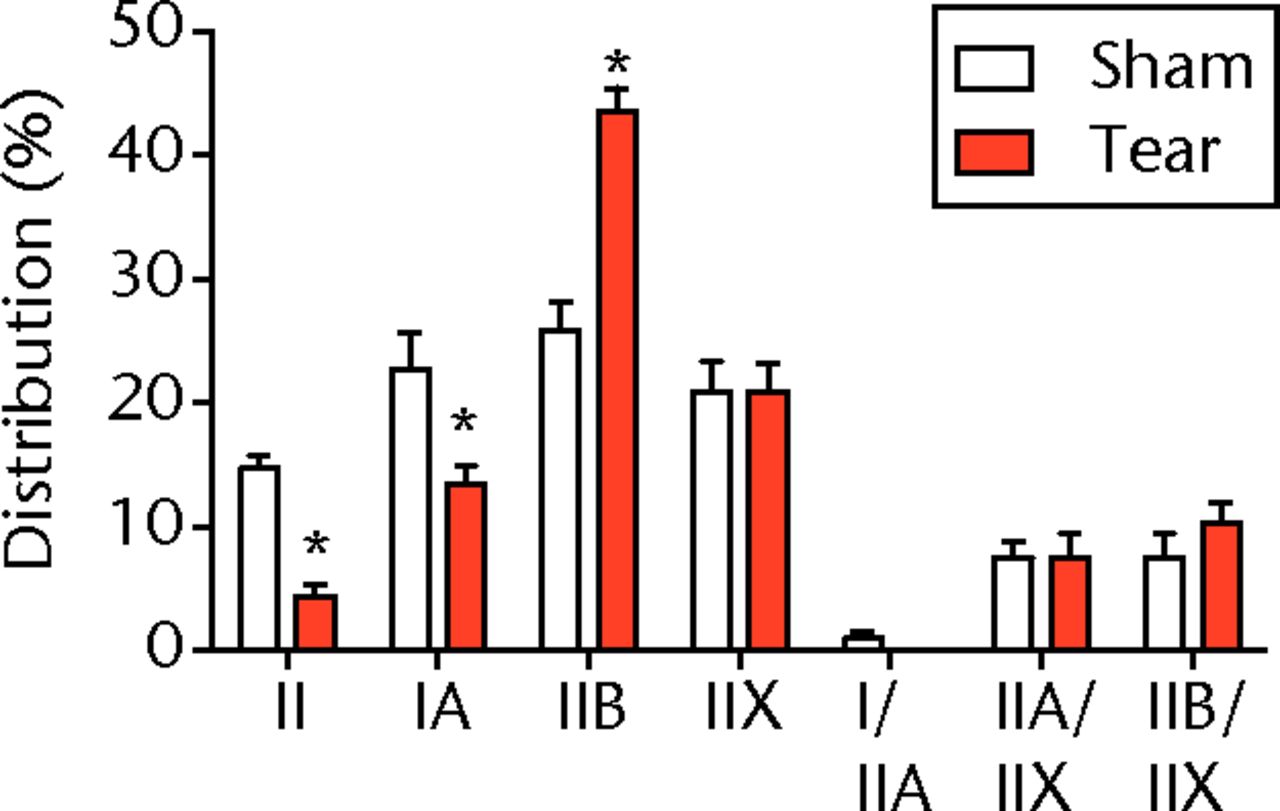
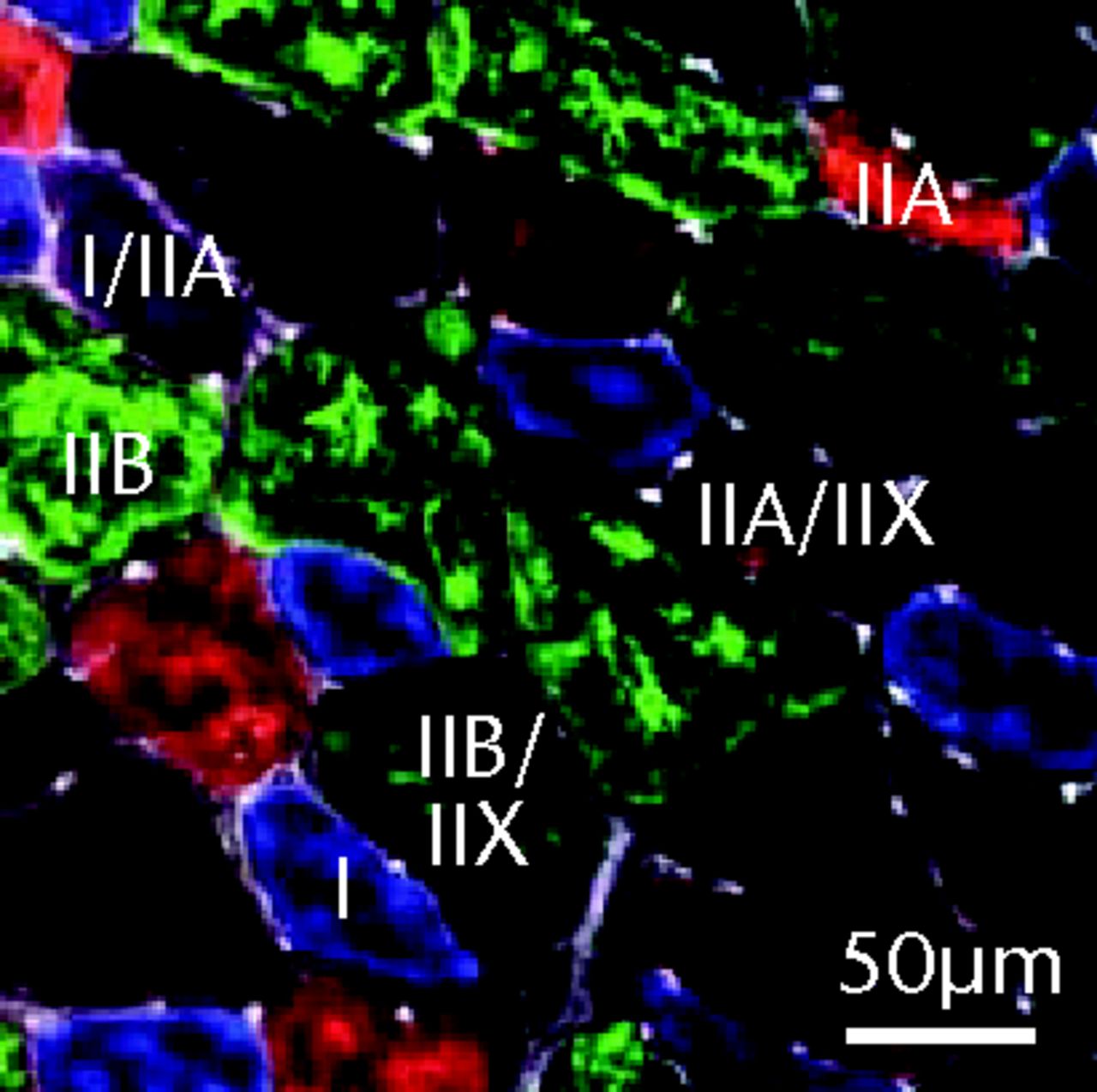
Figs. 2a - 2c
Muscle fibre type size and percent composition. Figure 2a – Graphs showing cross-sectional area (CSA) and b) percent distribution of myosin heavy chain isoform of muscle fibres from sham-operated control and torn rotator cuff muscles. Figure 2c - Representative immunohistochemistry demonstrating different fibre types. White, extracellular matrix (WGA-lectin); blue, MHC I; red, MHC IIA; green, MHC IIB; black, MHC IIX; blue-red, hybrid MHC I/IIA; red-black, hybrid MHC IIA/IIX; green-black, hybrid MHC IIB/IIX. Values are mean and sem. n = 5 muscles. * different from sham-operated control group (p < 0.05).
We next measured the expression of mRNAs and miRNAs involved in adipogenesis and lipid storage, ECM synthesis, autophagy, inflammation and macrophage accumulation. For genes involved with lipid synthesis and storage, there was an increase in PPAR-γ, PLD1 and miR-27a, while GPAT4 and FIT2 were downregulated (Figs. 3a and 3b, 4a and 4b). A significant upregulation in several ECM synthesis and remodelling genes were observed, including Type Iα2 collagen, MMP2, MMP9, MMP14, TIMP1, TIMP2, FSP-1, tenomodulin, miR-21, miR-31, miR-214 and miR-221 (Fig. 3c and 4c). There was also an increase in several genes that induce inflammation and muscle atrophy, including COX1, COX2, IL-1β, IL-6 (Fig. 3e), while miRNAs involved with muscle growth and adaptation, miR-133a, miR-133b and miR-499, were downregulated (Fig. 4a). Rotator cuff tear also increased the expression of the pan-macrophage marker F4/80, as well as M1 markers CD11b, CD68 and CCR7 (Fig. 3f). A decrease in the M2 marker CD168 was also observed (Fig. 3f). No differences in autophagy-related genes or the let-7 cluster of miRNAs were observed (Fig. 3d, 4d). Consistent with gene expression data, increases in lipid deposition and deposits of lipid-laden macrophages were also observed in torn rotator cuff muscles (Fig. 5).
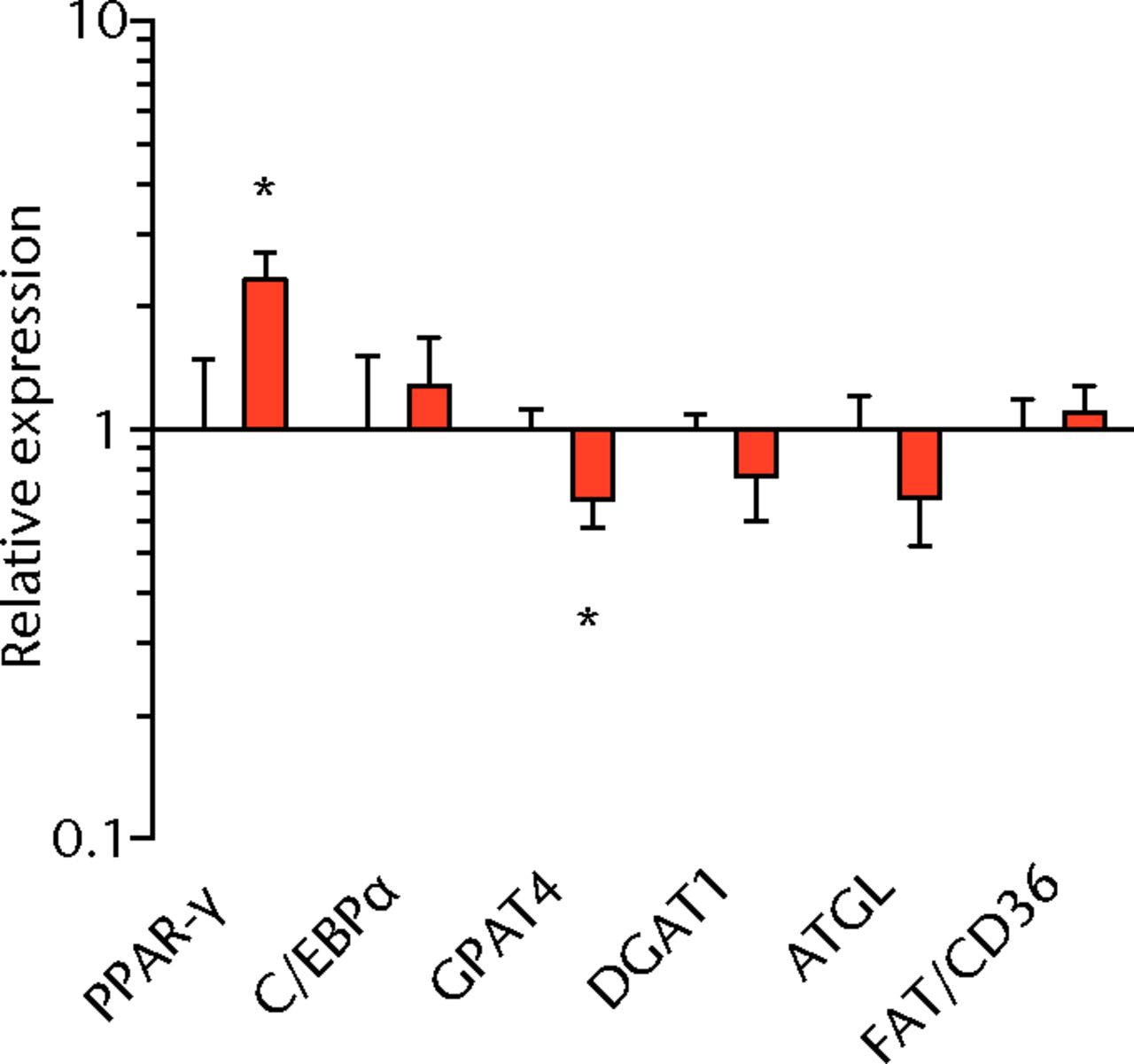
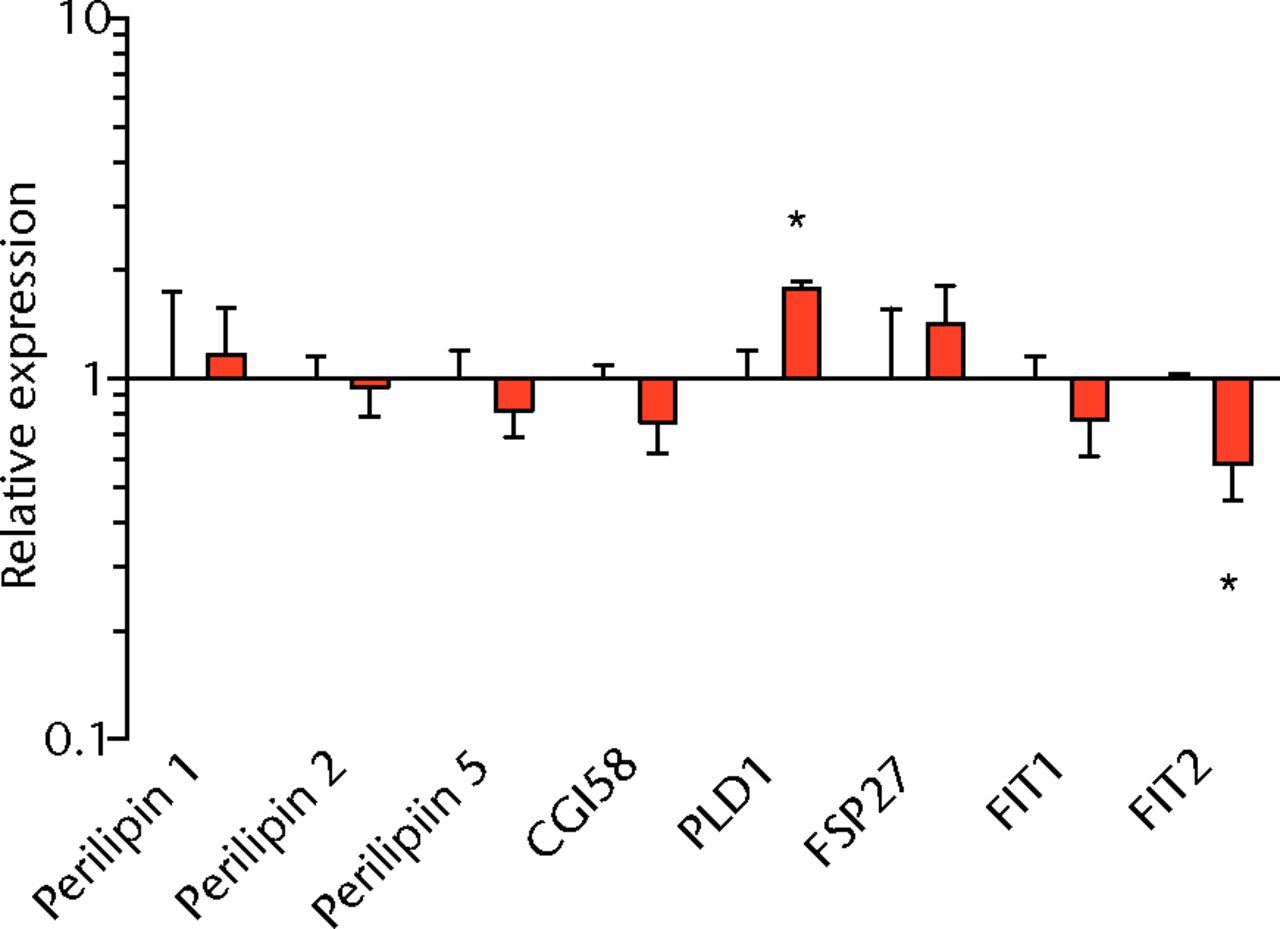
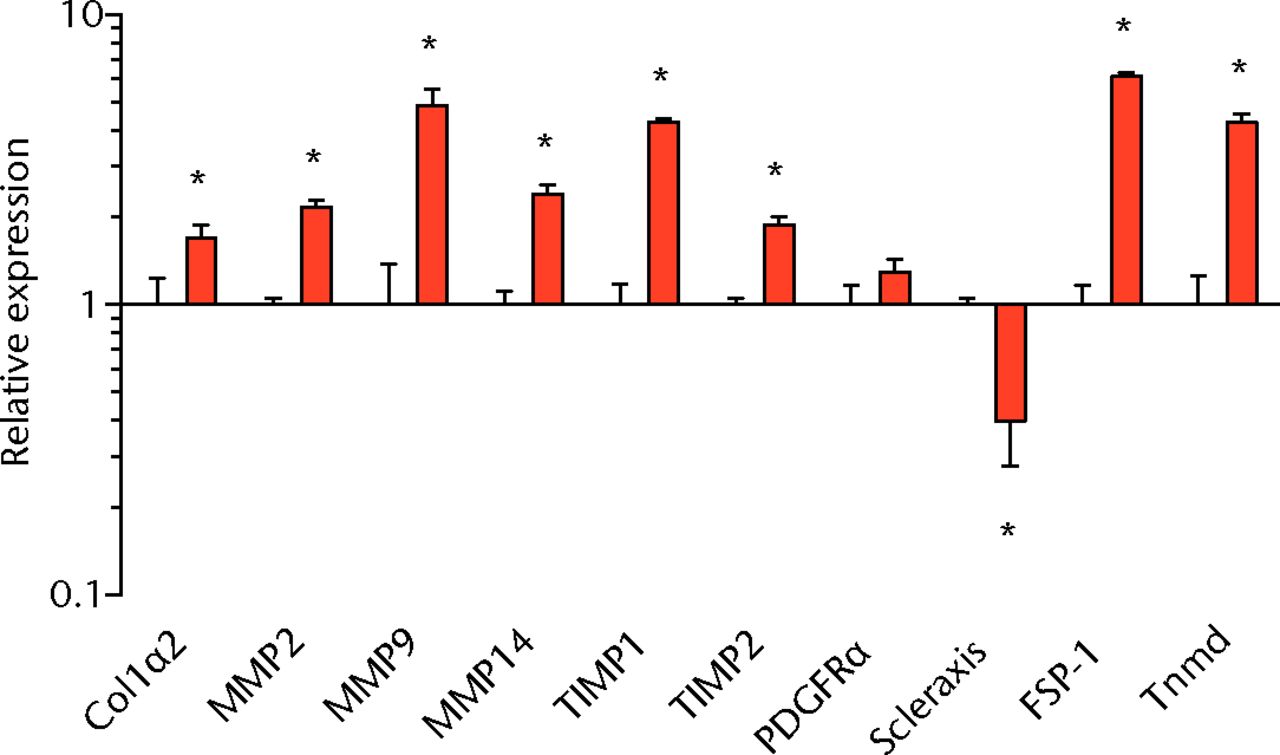
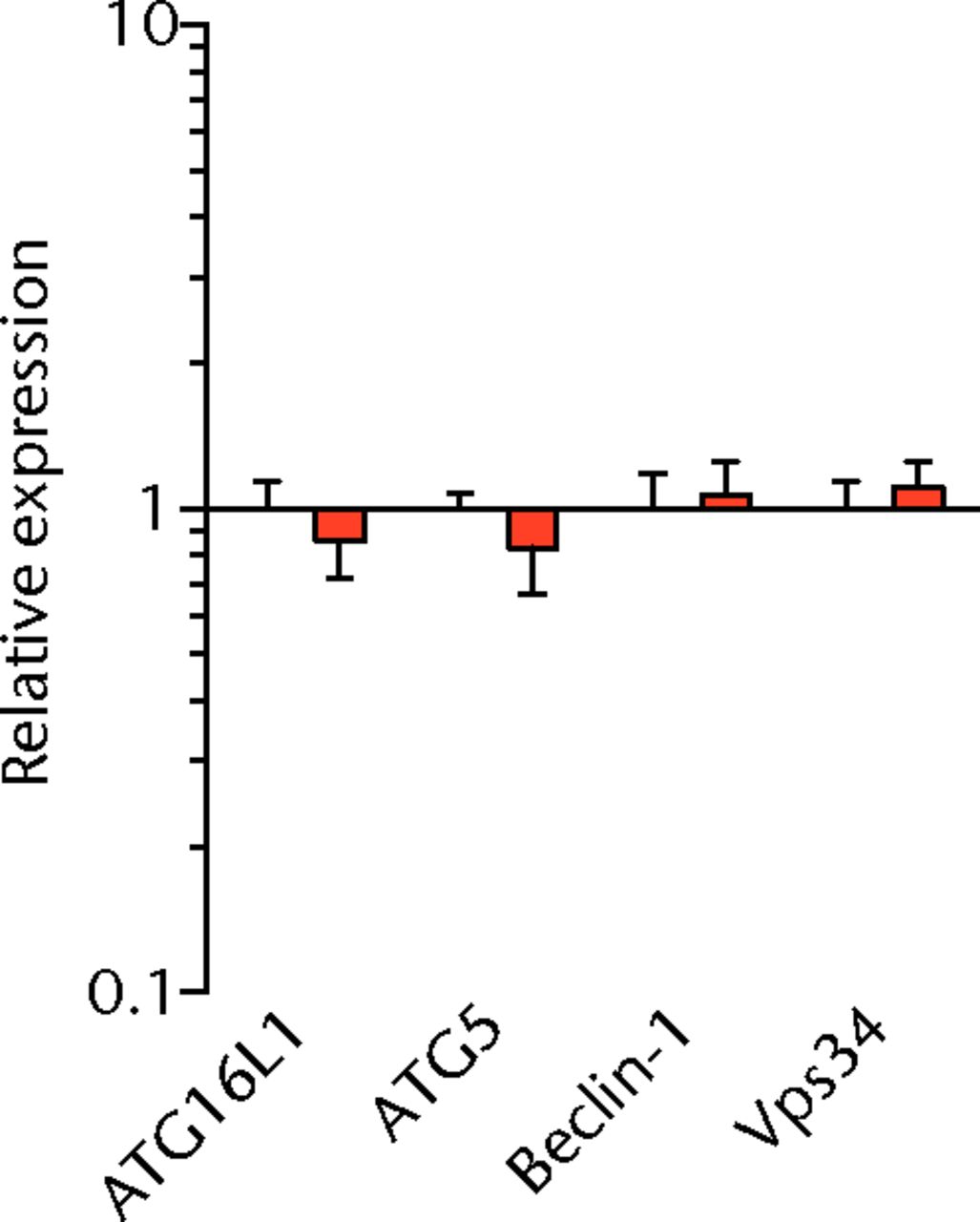
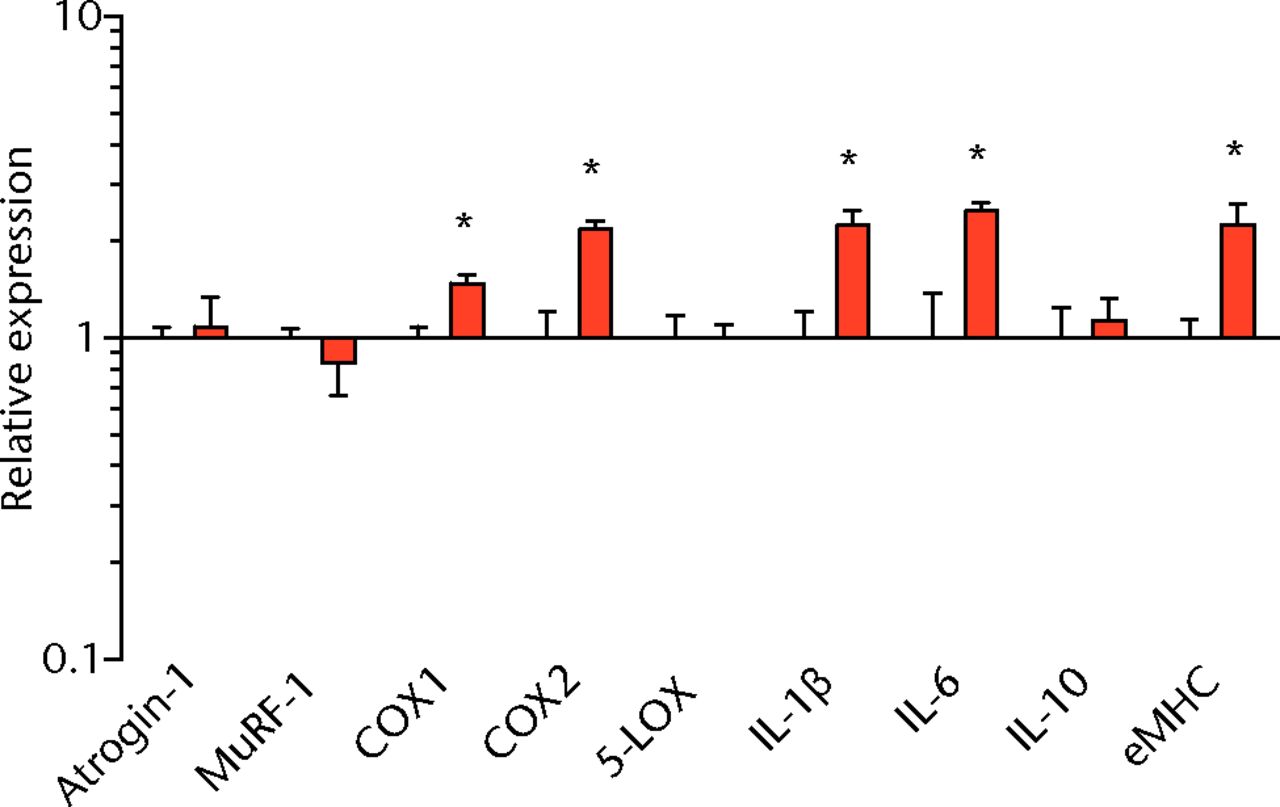
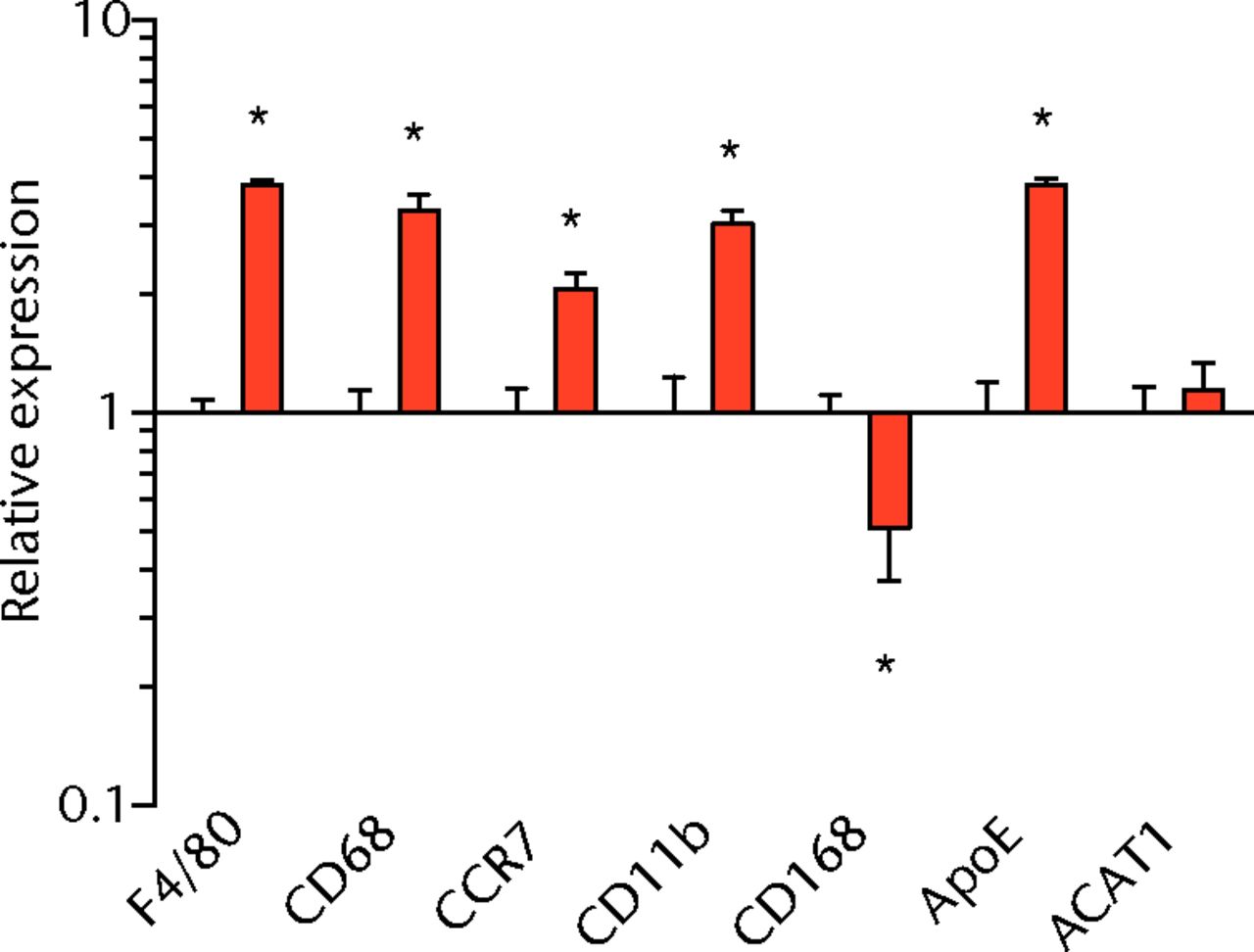
Figs. 3a - 3f
Graphs showing mRNA expression. Expression of genes associated with a) adipogenesis, b) lipid storage, c) extracellular matrix synthesis and fibrosis, d) autophagy, e) inflammation and atrophy and f) macrophage and fatty macrophage accumulation. Target gene expression was normalised to ß-actin. Sham-operated control values are plotted on the left, and torn muscles are plotted on the right and shown in orange. Values are mean and sem. n = 5 muscles from each group. * significantly different from sham-operated control group (p < 0.05).
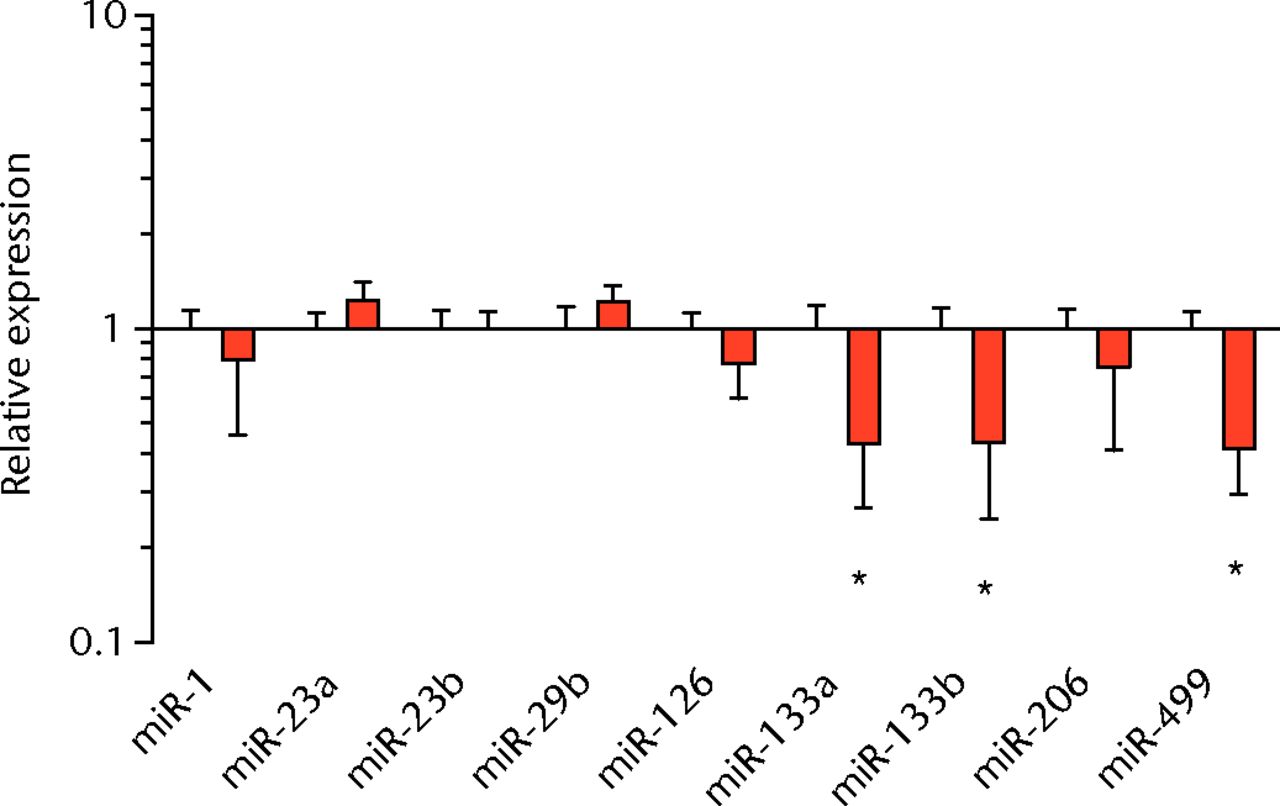
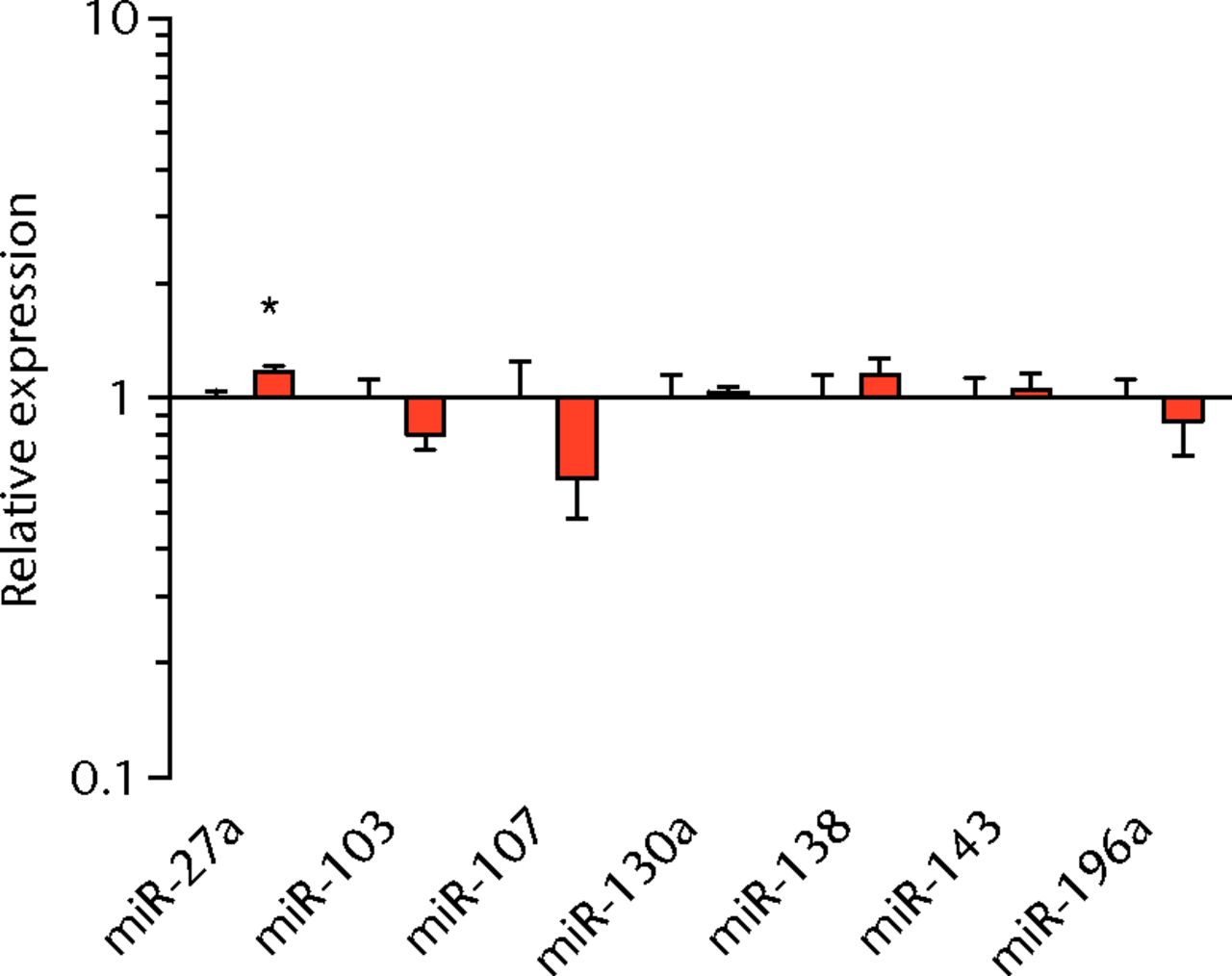
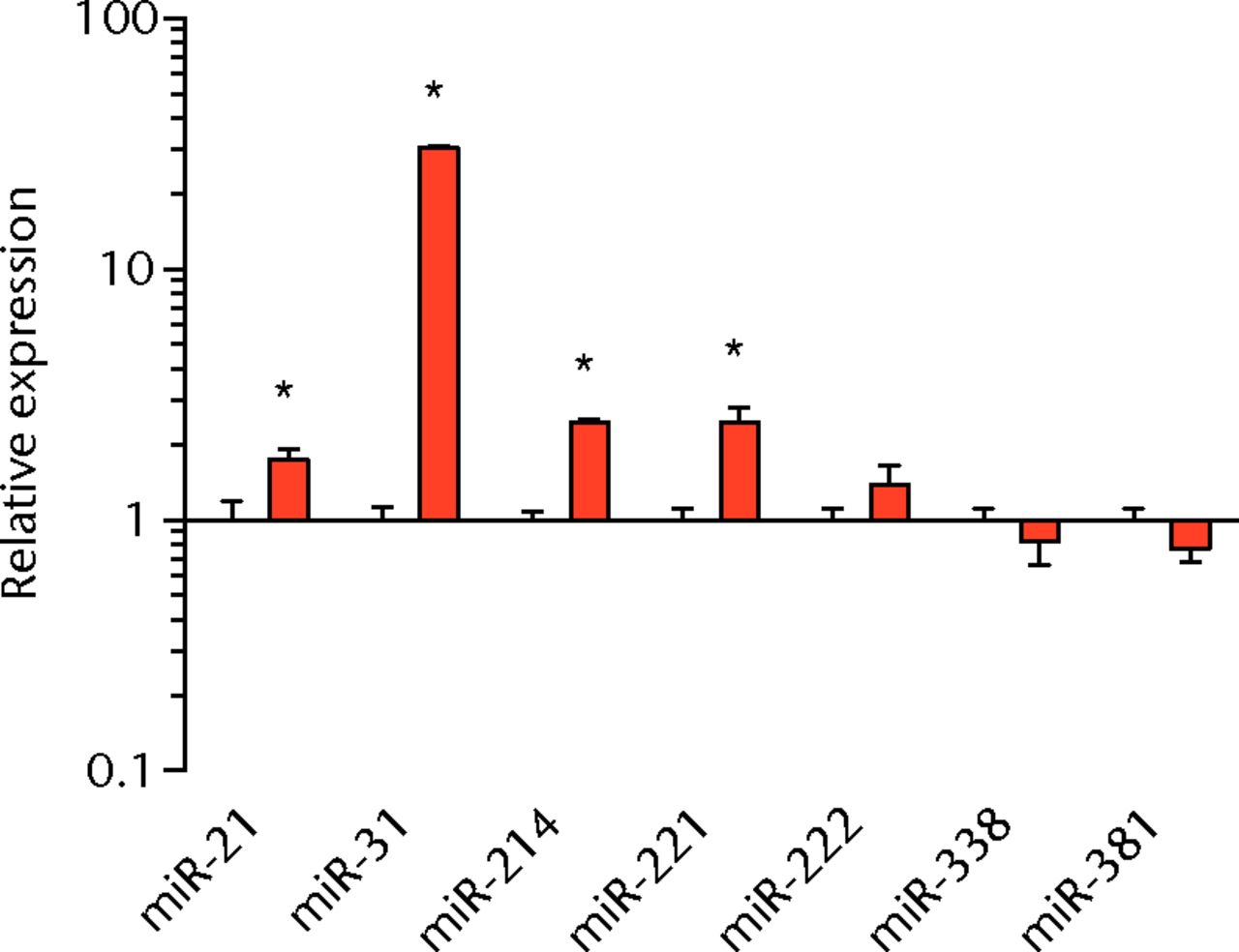
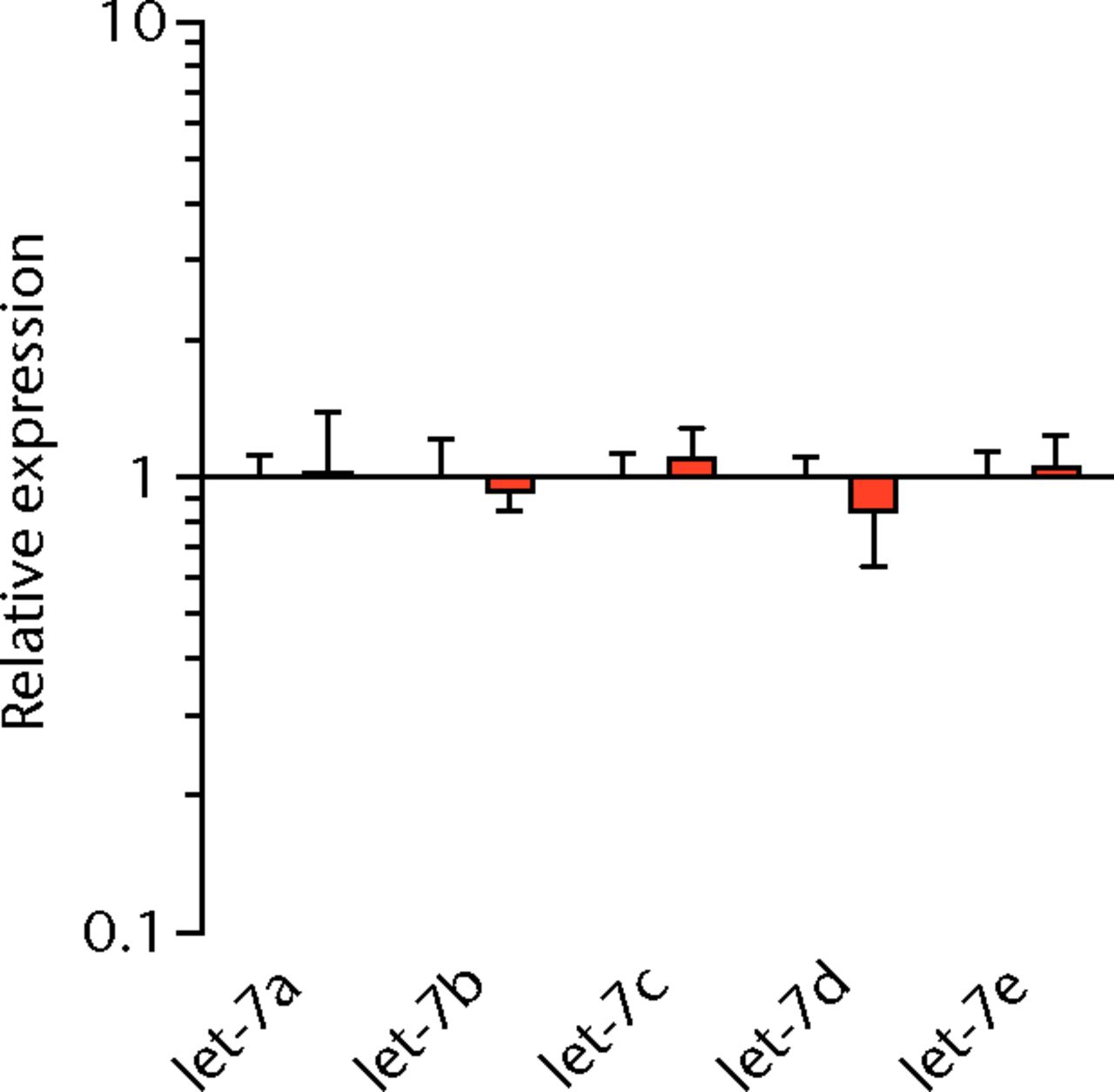
Figs. 4a - 4d
Graphs showing miRNA expression. Expression of miRNAs associated with a) muscle adaptation, b) lipid storage and synthesis, c) fibrosis and extracellular matrix synthesis and d) the let-7 cluster. Target miRNA expression was normalised to Rnu6. Sham-operated control values are plotted on the left, and torn muscles are plotted on the right and shown in orange. Values are mean and sem; n = 5 muscles from each group. * significantly different from sham-operated control group (p < 0.05).
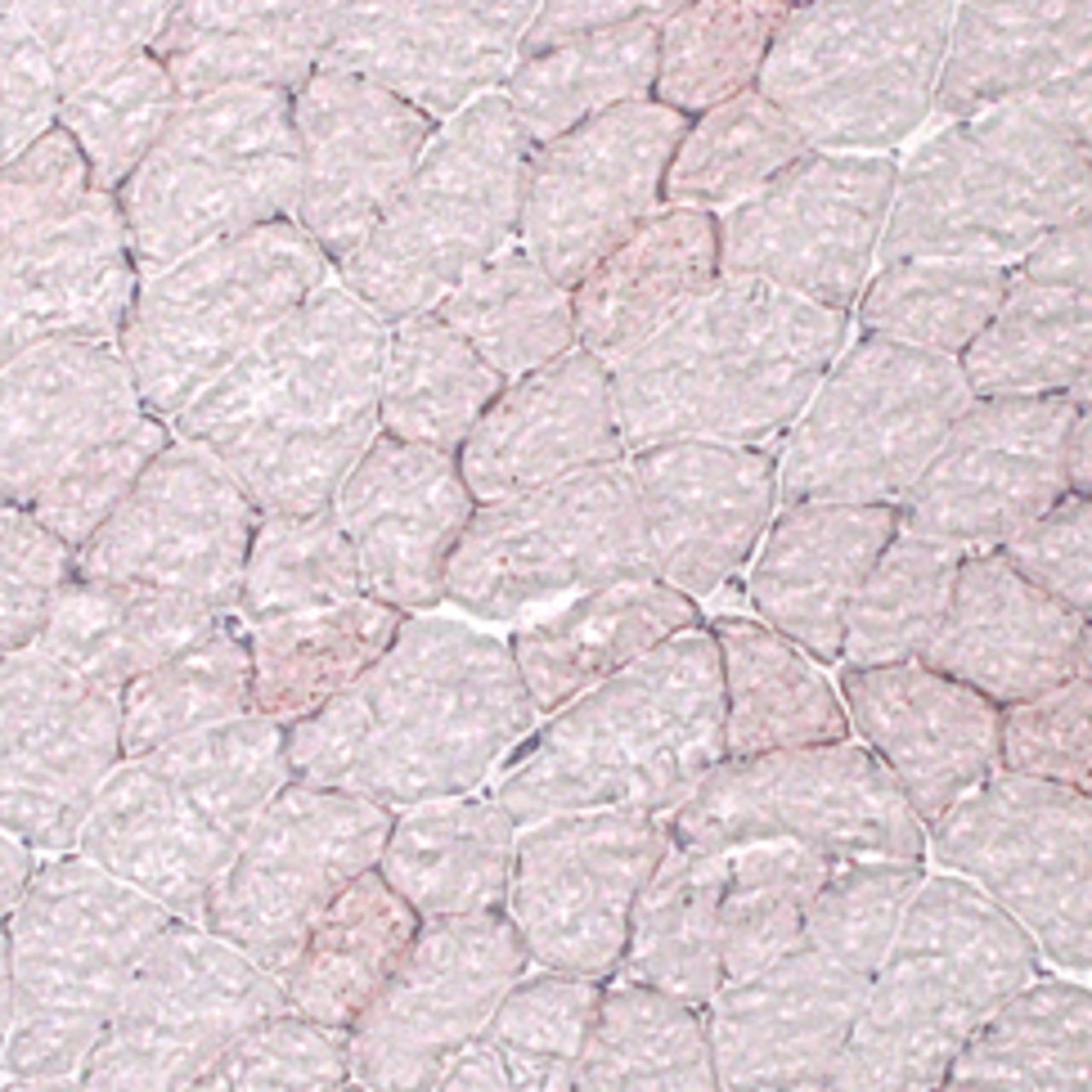
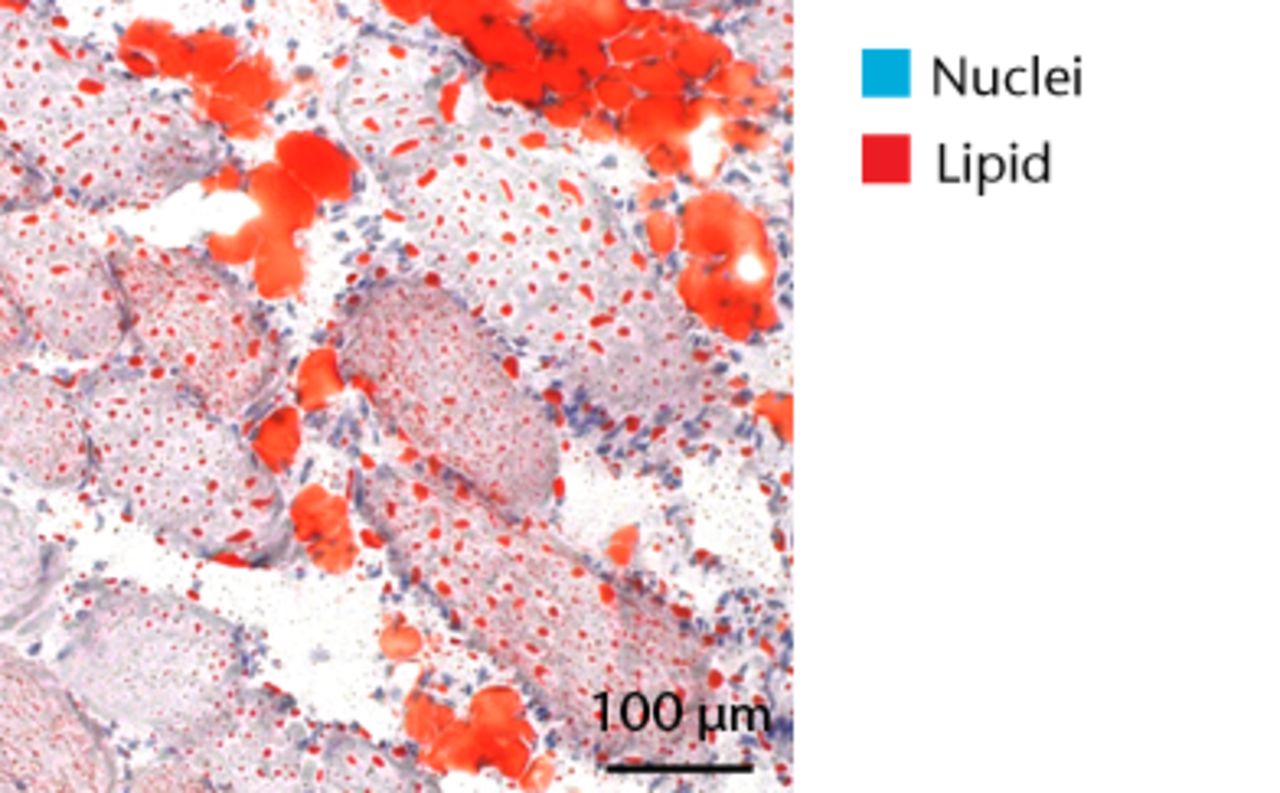
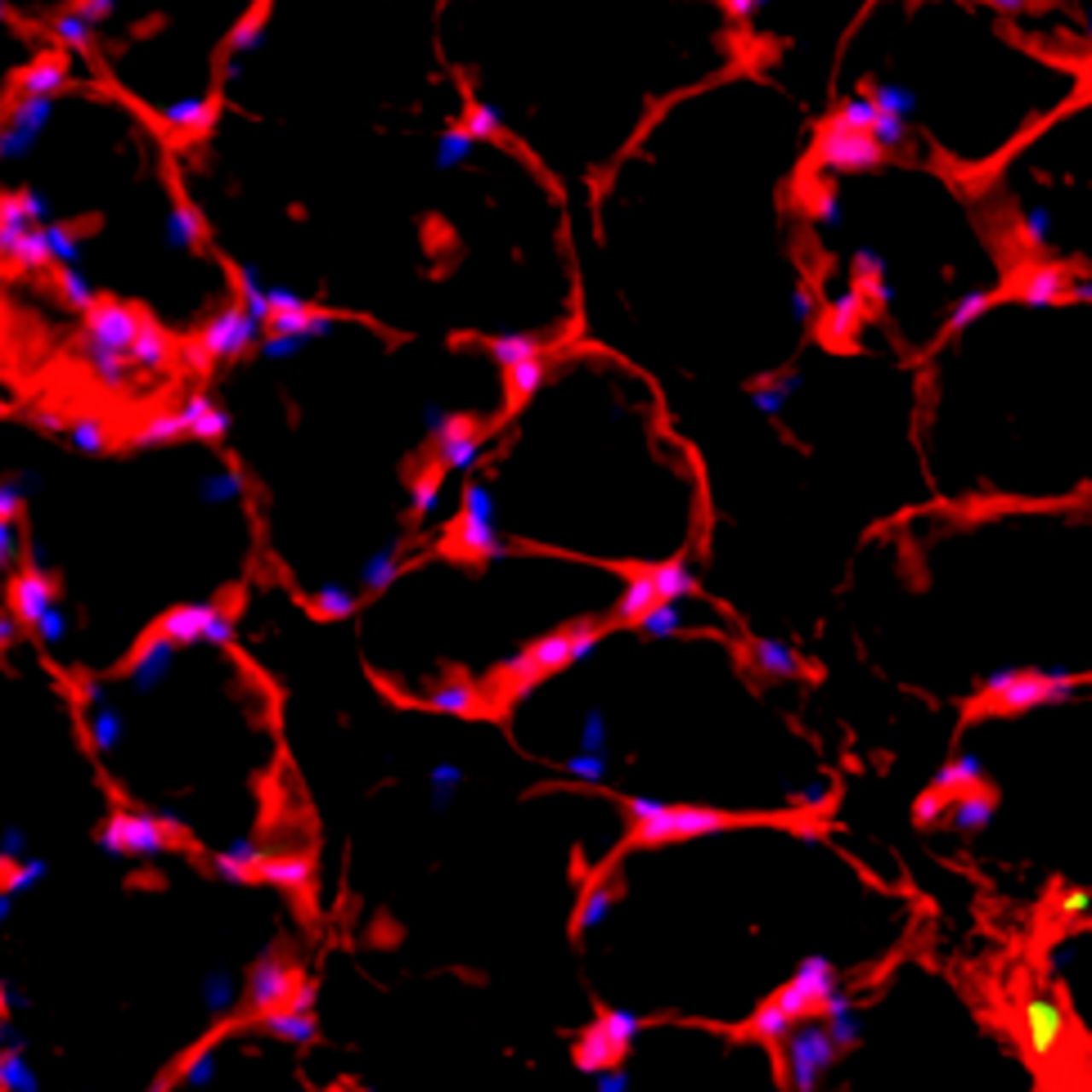
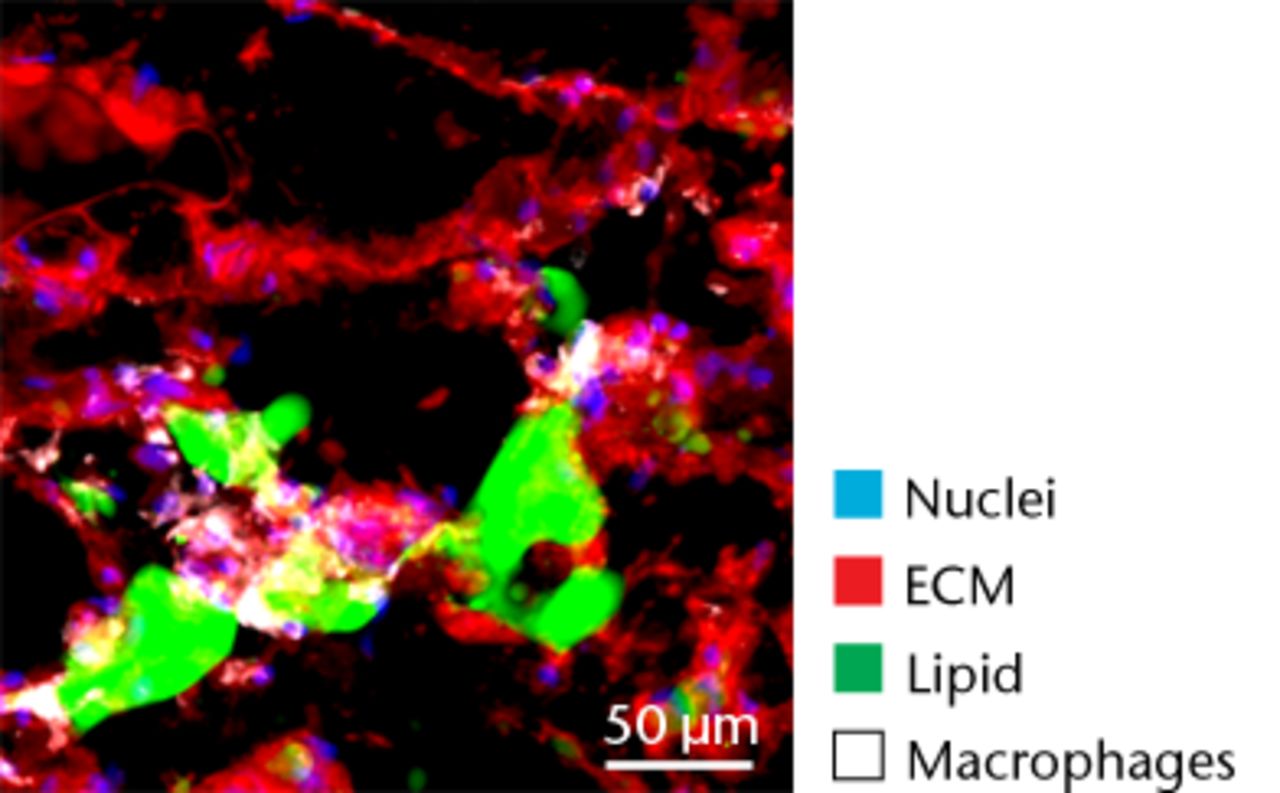
Figs. 5a - 5d
Representative Oil red O histology sections from a) sham-operated control and b) torn rotator cuff muscles. Blue represents haematoxylin; red, Oil red O. Representative immunohistochemistry from c) sham-operated control and d) torn rotator cuff muscles; blue represents nuclei (DAPI); red, extracellular matrix (WGA lectin); green, lipid (BODIPY); white, macrophages (F4/ 80).
Discussion
Chronic rotator cuff tears are a frequent and debilitating injury, and options to treat fatty degeneration after rotator cuff tear are currently limited. T lymphocytes are thought to play an important role in regulating the inflammatory response and regeneration of muscle after injury,7,8 and this was the first study, to our knowledge, to specifically evaluate the response of skeletal muscle to a chronic injury in an animal that lacked competent T cells. The sFo of sham-operated muscle fibres of nude rats, as well as the magnitude of mass and sFo reduction and increase in the number of type IIB muscle fibres that occurred after rotator cuff tear in nude rats, was similar to what was previously observed in immune competent adult rats.18,19 A reduction in sFo and accumulation of type IIB muscle fibres often occurs in states of chronic injury,18,24 and these results suggest that T-cell deficient rats develop a reduction in muscle function after a rotator cuff tear in a fashion that is generally similar to what is observed in T-cell competent rats.
T cells, along with many other inflammatory cell populations, secrete many different types of interleukins, prostaglandins, and leukotrienes, and several of these molecules have important roles in regulating muscle inflammation, fibrosis and atrophy after injury.5,25,26 IL-1β and IL-6 are pro-inflammatory cytokines that promote muscle atrophy5 and were upregulated following tear in T-cell deficient rats, although the expression of the anti-inflammatory gene IL-10 was not different. COX-1 and COX-2 catalyse the conversion of arachidonic acid into pro-inflammatory prostaglandins that inhibit muscle stem-cell proliferation and muscle regeneration,25,27 and were upregulated after tear, although 5-LOX which converts arachidonic acid into pro-inflammatory leukotrienes26 was not upregulated. Atrogin-1 and MuRF-1 are the major E3 ubiquitin ligases that serve as key rate-limiting enzymes in skeletal muscle protein degradation,28 but their expression was not different between sham-operated and torn rotator cuff muscles, which is consistent with observations from previous work in T-cell competent rats.18,29 In adult animals, eMHC is a marker of muscle fibres that have been injured or damaged in some fashion and are undergoing regeneration,24 and in nude rats, torn rotator cuff muscles had an increase in eMHC expression. While an induction in autophagy-related transcripts was observed in torn rotator cuff muscles from T-cell competent rats,18 no changes in the autophagy-related transcripts Atg16L1, Atg5, Beclin-1 or Vps34 were observed in the current study. For miRNAs which play a role in myogenesis and muscle regeneration,30,31 miR-1, -23a, -23b, -29b, -126 and -206 were unchanged following a tear, although miR-133a, -133b and -499 were downregulated in torn rotator muscles. The let-7 cluster of miRNAs play an important role in regulating myogenesis,32 but no change in the expression of any let-7 cluster member was observed in torn rotator cuff muscles. The results from this study suggest that although several pro-inflammatory markers are upregulated, similar to other chronic models of rotator cuff tear, persistent activation of atrogin-1 and MuRF-1 and activation of autophagy pathways are dispensable for chronic atrophy and weakness in torn rotator cuff muscles of T-cell deficient rats.
Fibrosis is often observed in chronically torn rotator cuff muscles,3,18,19 and in the current study, torn rotator cuff muscles had an increase in type I collagen gene expression and a robust accumulation of connective tissue apparent from immunohistochemistry. Genes associated with ECM degradation and remodelling, MMP2, MMP9 and MMP1433 were elevated, but we also observed increases in the MMP inhibitors TIMP1 and TIMP2. FSP-1 and tenomodulin are markers of fibroblast cells,34,35 and are elevated in torn muscles. For other fibroblast markers, PDGFR-α was not different, and scleraxis was downregulated. MiRNAs associated with fibrosis, miR-21, -214, -221, and -3136-38 were upregulated following a chronic rotator cuff tear, however, miR-222, which is closely associated with miR-221 functionally,36-38 was not different. Overall, the changes in mRNA and miRNA transcripts, and the gross appearance of muscles via histology indicate that the regulation of ECM production and fibroblast proliferation was similar to what was seen in our previous study in T-cell competent rats.18,19
The deposition of fat in and around muscle fibres is commonly observed after a chronic rotator cuff tear.3,18,19 In the T-cell deficient rats, the torn rotator cuff muscles from nude rats exhibited lipid accumulation similar to that observed in T-cell competent rats.18,19 PPAR-γ and C/EBPα direct the transport and storage of fatty acids, as well as the synthesis of intracellular lipid droplets. In agreement with previous studies,18,39-41 PPAR-γ expression was elevated after rotator cuff tear in nude rats, although C/EBPα expression was not different between sham-operated control and torn muscles. MIR-27a, which functions to inhibit adipogenesis by binding to the 3’ regulatory region of PPAR-γ,42 was upregulated in torn rotator cuff muscles. In our previous study,18 there was a much greater increase in PPAR-γ expression in torn rotator cuff muscles, but no change in miR-27a was observed. The increase in miR-27a expression in the current study may explain the smaller increase in PPAR-γ expression that occurred after a rotator cuff tear. Although further study is necessary, T cells may be responsible for the attenuation of miR-27a expression after a rotator cuff tear, leading to a larger increase in PPAR-γ in T-cell competent rats.
Lipid droplets in many tissues are dynamic organelles and direct many cellular activities ranging from energy metabolism to inflammation and apoptosis.43,44 The biology of lipid droplet formation in skeletal muscle tissue has been extensively studied in the context of endurance training or diabetes, which share many overlapping molecular pathways.45,46 In oil red O images, the size of intramyocellular lipid droplets in T-cell deficient rats is roughly the same size as a typical observation in T-cell competent animals after a rotator cuff tear,18,19 but is several orders of magnitude larger than what is typically observed when lipid accumulates in skeletal muscle tissue of endurance-trained athletes or type 2 diabetics.45,46 To gain greater insight into the processes that regulate lipid droplet formation, we evaluated the expression of several canonical genes that directly regulate the size, number and mobilisation of lipid droplets both in muscle and non-muscle cells, which are discussed in detail elsewhere.18,21,47 These genes included perilipin 1, 2 and 5, as well as FAT/CD36, DGAT1, GPAT, FIT1, FIT2, FSP27, CGI58, ATGL and PLD1. Despite the presence of extremely large lipid droplets, there were no changes in perilipin 1, 2 and 5, FSP27, FAT/CD36, DGAT1, and FIT1 in T-cell deficient rats, and a decrease in GPAT4 and FIT2 expression. Although this is in agreement with prior observations in T-cell competent rats,18,19 these genes would otherwise be anticipated to be upregulated in the context of lipid accumulation in endurance-trained or diabetic muscle.48 Only one lipid accumulation gene, PLD1, was upregulated in the torn cuff muscles of T-cell deficient rats. MiRNAs involved in adipogenesis,49 miR-103, -107, -130a, -138, -143 and -196a, were not different between sham-operated control and torn rotator cuff muscles. However, miR-133a and -133b, which are involved in inhibiting the conversion of satellite cells into brown adipocytes,50,51 were downregulated. For intramyocellular lipid, many of the fat deposits in the current study contained macrophages, which is consistent with our previous findings in T-cell competent rats.18,19 Together, these results indicate that T cells are not required for the development of fat accumulation after a rotator cuff tear. The lack of change in the expression of many canonical lipid uptake and storage genes after a rotator cuff tear further support the idea that the dramatic increase in fat that occurs with fatty degeneration, is not being actively used for metabolic purposes, and may be a pathological response to a rotator cuff tear.
There are at least two populations of macrophages that are thought to play a central role in regulating skeletal muscle degeneration and regeneration following injury. Classically-activated M1 macrophages promote muscle necrosis and degeneration, while alternately-activated M2 macrophages help to reduce local inflammation and stimulate regeneration.6 Following many muscle injuries, M1 marker levels increase immediately following muscle injury and return to baseline levels four to six days after injury, while M2 macrophages increase during this time and return to baseline levels ten to 14 days after injury.6 We previously demonstrated a marked accumulation of macrophages in torn rotator cuff muscles of T-cell competent rats,18 although we did not assess markers of specific M1 and M2 macrophages. In the current study, an increase in the expression of the pan-macrophage marker F4/80 was observed, as there was for M1 markers CD11b, CD68 and CCR7. A downregulation in the M2 marker CD168 was observed. The sustained elevation of M1 macrophage transcripts with a concurrent decrease in M2 transcripts suggests a chronic inflammatory state within torn rotator cuff muscles that is consistent with previous findings.21
While adipocytes accumulate after a rotator cuff tear and contribute to the overall increase in lipid content in torn muscles,3,41 we also identified a class of lipid-laden macrophages that are found in a torn rotator cuff muscles,18,21 which resemble a class of fatty macrophages seen in atherosclerosis. These fatty macrophages are often referred to as ‘foam cells’ and function to promote local inflammation in atherosclerotic plaques.48 In atherosclerosis, fatty macrophages can enhance lipid plaque formation which subsequently exacerbates the severity of the disorder,48 and these cells may also play an important role in fatty degeneration in rotator cuff tears. Differentiation of macrophages into fatty macrophages shares some overlap with genetic programmes that regulate adipocyte differentiation and maturation. PPAR-γ promotes the differentiation of macrophages into fatty macrophages in atherosclerotic plaques, and once differentiated, these cells highly express ApoE, FSP27 and ACAT1.52,53 In the current study, elevations in PPAR-γ and ApoE were observed in torn rotator cuff muscles, although no changes in ACAT1 or FSP27 were present. Additionally, we observed fatty macrophages that accumulate around lipid plaques in T-cell deficient rats via immunohistochemistry. T cells are thought to play an important role in coordinating the inflammation and regeneration of muscle after injury through direct interactions with muscle fibres as well as through the regulation of M1 and M2 macrophages,7-9 however, the results from the current study indicate that T cells are not required for fatty macrophage accumulation in skeletal muscle after injury.
There are several limitations to this study. Due to many shared anatomical features with humans, the rat is an ideal animal model for the study of rotator cuff tears, however, rats do not develop the severity of fatty degeneration seen in human patients.3,19,20,41 We chose to evaluate chronic rotator cuff tears using a single time point of one month to allow for comparisons to our previous studies,18,19 but we did not evaluate changes at other time points. We used the contralateral side as the sham-operated control muscle, and animals were still able to use both forelimbs for ambulation. While we did not notice any signs of muscle injury in sham-operated control muscles, it is possible that these muscles experienced a small amount of muscle hypertrophy. For single fibre contractility measurements, we used type II muscle fibres, and did not measure changes by specific type II myosin isoform. We selected this approach due to the greater amount of type II fibres in the cuff, and sFo does not change between fibres of different type II myosin isoforms.54 While we measured the expression of multiple mRNA molecules, we did not directly quantify protein levels, and changes in gene expression may not reflect changes in protein abundance. We point to many similarities between the current study and our previous study using immunocompetent rats, and while we used slightly more mature rats in the previous study, we do not believe this affects the ability to make these comparisons with the current study. Despite the presence of these limitations, this study provided insight into the cellular and molecular changes in rotator cuff muscles of T-cell deficient rats.
Conclusion
T lymphocytes are thought to play an important role in modulating the inflammatory and regeneration responses of injured skeletal muscle, but their function in directing fatty degeneration in the rotator cuff had not previously been studied. Compared with a similar injury in T-cell competent rats,18,19 nude rats displayed a similar response to a chronic rotator cuff tear. These results suggest that T cells may not play an important role in regulating muscle degeneration or remodelling following chronic muscle unloading. Furthermore, as there has been much interest in the use of stem-cell therapy to enhance rotator cuff regeneration,15 and evaluating human-derived stem cells in animal models of injury and disease is important in identifying safe and effective stem-cell therapies,17 the general similarities between nude rats and T-cell competent rats indicate that the nude rats are likely a favourable preclinical model for the study of human-derived stem cells or other xenografts that may enhance the treatment of chronically torn rotator cuff muscles. However, further work may need to be undertaken to investigate this definitively.
1 Colvin AC , EgorovaN, HarrisonAK, MoskowitzA, FlatowEL. National trends in rotator cuff repair. J Bone Joint Surg [Am]2012;94-A:227–233.CrossrefPubMed Google Scholar
2 Bedi A , DinesJ, WarrenRF, DinesDM. Massive tears of the rotator cuff. J Bone Joint Surg [Am]2010;92-A:1894–1908. Google Scholar
3 Goutallier D , PostelJM, BernageauJ, LavauL, VoisinMC. Fatty muscle degeneration in cuff ruptures. Pre- and postoperative evaluation by CT scan. Clin Orthop Relat Res1994;304:78–83.PubMed Google Scholar
4 Gladstone JN , BishopJY, LoIK, FlatowEL. Fatty infiltration and atrophy of the rotator cuff do not improve after rotator cuff repair and correlate with poor functional outcome. Am J Sports Med2007;35:719–728. Google Scholar
5 Smith C , KrugerMJ, SmithRM, MyburghKH. The inflammatory response to skeletal muscle injury: illuminating complexities. Sports Med2008;38:947–969.CrossrefPubMed Google Scholar
6 Tidball JG , VillaltaSA. Regulatory interactions between muscle and the immune system during muscle regeneration. Am J Physiol Regul Integr Comp Physiol2010;298:R1173–R1187.CrossrefPubMed Google Scholar
7 Mann CJ , PerdigueroE, KharrazY, et al.Aberrant repair and fibrosis development in skeletal muscle. Skelet Muscle2011;1:21.CrossrefPubMed Google Scholar
8 Sugihara T , OkiyamaN, SuzukiM, et al.Definitive engagement of cytotoxic CD8 T cells in C protein-induced myositis, a murine model of polymyositis. Arthritis Rheum2010;62:3088–3092.CrossrefPubMed Google Scholar
9 Wolfs IM , DonnersMM, de WintherMP. Differentiation factors and cytokines in the atherosclerotic plaque micro-environment as a trigger for macrophage polarisation. Thromb Haemost2011;106:763–771.CrossrefPubMed Google Scholar
10 Olefsky JM , GlassCK. Macrophages, inflammation, and insulin resistance. Annu Rev Physiol2010;72:219–246.CrossrefPubMed Google Scholar
11 Njobvu P , McGillP. Soft tissue rheumatic lesions and HIV infection in Zambians. J Rheumatol2006;33:2493–2497.PubMed Google Scholar
12 Nehls M , PfeiferD, SchorppM, HedrichH, BoehmT. New member of the winged-helix protein family disrupted in mouse and rat nude mutations. Nature1994;372:103–107.CrossrefPubMed Google Scholar
13 Vos JG , BerkvensJM, KruijtBC. The athymic nude rat. I. Morphology of lymphoid and endocrine organs. Clin Immunol Immunopathol1980;15:213–228.CrossrefPubMed Google Scholar
14 Whitby EH , SparshottSM, BellEB. Allograft rejection in athymic nude rats by transferred T-cell subsets. I. The response of naive CD4+ and CD8+ thoracic duct lymphocytes to complete allogeneic incompatibilities. Immunology1990;69:78–84.PubMed Google Scholar
15 Isaac C , GharaibehB, WittM, WrightVJ, HuardJ. Biologic approaches to enhance rotator cuff healing after injury. J Shoulder Elbow Surg2012;21:181–190.CrossrefPubMed Google Scholar
16 Caplan AI . Adult mesenchymal stem cells for tissue engineering versus regenerative medicine. J Cell Physiol2007;213:341–347.CrossrefPubMed Google Scholar
17 Zhou P , HohmS, CapocciaB, et al.Immunodeficient mouse models to study human stem cell-mediated tissue repair. Methods Mol Biol2008;430:213–225.CrossrefPubMed Google Scholar
18 Gumucio JP , DavisME, BradleyJR, et al.Rotator cuff tear reduces muscle fiber specific force production and induces macrophage accumulation and autophagy. J Orthop Res2012;30:1963–1970.CrossrefPubMed Google Scholar
19 Liu X , ManzanoG, KimHT, FeeleyBT. A rat model of massive rotator cuff tears. J Orthop Res2011;29:588–595.CrossrefPubMed Google Scholar
20 Soslowsky LJ , CarpenterJE, DeBanoCM, BanerjiI, MoalliMR. Development and use of an animal model for investigations on rotator cuff disease. J Shoulder Elbow Surg1996;5:383–392.CrossrefPubMed Google Scholar
21 Gumucio JP , KornMA, SaripalliAL, et al.Aging-associated exacerbation in fatty degeneration and infiltration after rotator cuff tear. J Shoulder Elbow Surg2014;23:99–108.CrossrefPubMed Google Scholar
22 Mendias CL , KayupovE, BradleyJR, BrooksSV, ClaflinDR. Decreased specific force and power production of muscle fibers from myostatin-deficient mice are associated with a suppression of protein degradation. J Appl Physiol (1985)2011;111:185–191.CrossrefPubMed Google Scholar
23 Schmittgen TD , LivakKJ. Analyzing real-time PCR data by the comparative C(T) method. Nat Protoc2008;3:1101–1108.CrossrefPubMed Google Scholar
24 Schiaffino S , ReggianiC. Molecular diversity of myofibrillar proteins: gene regulation and functional significance. Physiol Rev1996;76:371–423.CrossrefPubMed Google Scholar
25 Mendias CL , TatsumiR, AllenRE. Role of cyclooxygenase-1 and -2 in satellite cell proliferation, differentiation, and fusion. Muscle Nerve2004;30:497–500.CrossrefPubMed Google Scholar
26 Stables MJ , GilroyDW. Old and new generation lipid mediators in acute inflammation and resolution. Prog Lipid Res2011;50:35–51.CrossrefPubMed Google Scholar
27 Shen W , LiY, TangY, CumminsJ, HuardJ. NS-398, a cyclooxygenase-2-specific inhibitor, delays skeletal muscle healing by decreasing regeneration and promoting fibrosis. Am J Pathol2005;167:1105–1117.CrossrefPubMed Google Scholar
28 Sandri M . Signaling in muscle atrophy and hypertrophy. Physiology (Bethesda)2008;23:160–170.CrossrefPubMed Google Scholar
29 Liu X , JoshiSK, SamaghSP, et al.Evaluation of Akt/mTOR activity in muscle atrophy after rotator cuff tears in a rat model. J Orthop Res2012;30:1440–1446.CrossrefPubMed Google Scholar
30 Wada S , KatoY, OkutsuM, et al.Translational suppression of atrophic regulators by microRNA-23a integrates resistance to skeletal muscle atrophy. J Biol Chem2011;286:38456–38465.CrossrefPubMed Google Scholar
31 Eisenberg I , AlexanderMS, KunkelLM. miRNAS in normal and diseased skeletal muscle. J Cell Mol Med2009;13:2–11.CrossrefPubMed Google Scholar
32 Crippa S , CassanoM, SampaolesiM. Role of miRNAs in muscle stem cell biology: proliferation, differentiation and death. Curr Pharm Des2012;18:1718–1729.CrossrefPubMed Google Scholar
33 Davis ME , GumucioJP, SuggKB, BediA, MendiasCL. MMP inhibition as a potential method to augment the healing of skeletal muscle and tendon extracellular matrix. J Appl Physiol (1985)2013;115:884–891.CrossrefPubMed Google Scholar
34 Calve S , IsaacJ, GumucioJP, MendiasCL. Hyaluronic acid, HAS1, and HAS2 are significantly upregulated during muscle hypertrophy. Am J Physiol Cell Physiol2012;303:C577–C588.CrossrefPubMed Google Scholar
35 Joe AW , YiL, NatarajanA, et al.Muscle injury activates resident fibro/adipogenic progenitors that facilitate myogenesis. Nat Cell Biol2010;12:153–163.CrossrefPubMed Google Scholar
36 Bauersachs J . Regulation of myocardial fibrosis by MicroRNAs. J Cardiovasc Pharmacol2010;56:454–459.CrossrefPubMed Google Scholar
37 Ogawa T , EnomotoM, FujiiH, et al.MicroRNA-221/222 upregulation indicates the activation of stellate cells and the progression of liver fibrosis. Gut 2012;61:1600–1609.CrossrefPubMed Google Scholar
38 Iizuka M , OgawaT, EnomotoM, et al.Induction of microRNA-214-5p in human and rodent liver fibrosis. Fibrogenesis Tissue Repair2012;5:12.CrossrefPubMed Google Scholar
39 Frey E , RegenfelderF, SussmannP, et al.Adipogenic and myogenic gene expression in rotator cuff muscle of the sheep after tendon tear. J Orthop Res2009;27:504–509.CrossrefPubMed Google Scholar
40 Itoigawa Y , KishimotoKN, SanoH, KanekoK, ItoiE. Molecular mechanism of fatty degeneration in rotator cuff muscle with tendon rupture. J Orthop Res2011;29:861–866.CrossrefPubMed Google Scholar
41 Kim HM , GalatzLM, LimC, HavliogluN, ThomopoulosS. The effect of tear size and nerve injury on rotator cuff muscle fatty degeneration in a rodent animal model. J Shoulder Elbow Surg2012;21:847–858.CrossrefPubMed Google Scholar
42 Kim SY , KimAY, LeeHW, et al.miR-27a is a negative regulator of adipocyte differentiation via suppressing PPARgamma expression. Biochem Biophys Res Commun2010;392:323–328.CrossrefPubMed Google Scholar
43 Beller M , ThielK, ThulPJ, JäckleH. Lipid droplets: a dynamic organelle moves into focus. FEBS Lett2010;584:2176–2182.CrossrefPubMed Google Scholar
44 Bozza PT , ViolaJP. Lipid droplets in inflammation and cancer. Prostaglandins Leukot Essent Fatty Acids2010;82:243–250.CrossrefPubMed Google Scholar
45 Amati F , DubéJJ, Alvarez-CarneroE, et al.Skeletal muscle triglycerides, diacylglycerols, and ceramides in insulin resistance: another paradox in endurance-trained athletes?Diabetes2011;60:2588–2597.CrossrefPubMed Google Scholar
46 Minnaard R , SchrauwenP, SchaartG, et al.Adipocyte differentiation-related protein and OXPAT in rat and human skeletal muscle: involvement in lipid accumulation and type 2 diabetes mellitus. J Clin Endocrinol Metab2009;94:4077–4085.CrossrefPubMed Google Scholar
47 Watt MJ , HoyAJ. Lipid metabolism in skeletal muscle: generation of adaptive and maladaptive intracellular signals for cellular function. Am J Physiol Endocrinol Metab2012;302:E1315–E1328.CrossrefPubMed Google Scholar
48 Cascieri MA . The potential for novel anti-inflammatory therapies for coronary artery disease. Nat Rev Drug Discov2002;1:122–130.CrossrefPubMed Google Scholar
49 McGregor RA , ChoiMS. microRNAs in the regulation of adipogenesis and obesity. Curr Mol Med2011;11:304–316.CrossrefPubMed Google Scholar
50 Liu W, Bi P, Shan T, et al. miR-133a regulates adipocyte browning in vivo. PLoS Genet 2013;9:e1003626. Google Scholar
51 Yin H , PasutA, SoleimaniVD, et al.MicroRNA-133 controls brown adipose determination in skeletal muscle satellite cells by targeting Prdm16. Cell Metab2013;17:210–224.CrossrefPubMed Google Scholar
52 Li H , SongY, LiF, et al.Identification of lipid droplet-associated proteins in the formation of macrophage-derived foam cells using microarrays. Int J Mol Med2010;26:231–239.CrossrefPubMed Google Scholar
53 Tontonoz P , NagyL, AlvarezJG, ThomazyVA, EvansRM. nPPARgamma promotes monocyte/macrophage differentiation and uptake of oxidized LDL. Cell1998;93:241–252. Google Scholar
54 Bottinelli R , CanepariM, PellegrinoMA, ReggianiC. Force-velocity properties of human skeletal muscle fibres: myosin heavy chain isoform and temperature dependence. J Physiol1996;495:573–586.CrossrefPubMed Google Scholar
Funding statement:
The authors report that their institution has received grants from the US Department of Defence and the US National Institute of Health which have contributed to this study.
Author contributions:
J. Gumucio: Study design, Data collection, Data analysis, Writing paper
M. Flood: Study design, Data collection, Data analysis
J. Harning: Data collection
A. Phan: Data collection
S. Roche: Data collection, Data analysis
E. Lynch: Data collection
A. Bedi: Study design, Data analysis, Writing paper
C. Mendias: Study design, Data collection, Data analysis, Writing paper
ICMJE Conflict of Interest:
None declared
©2014 The British Editorial Society of Bone & Joint Surgery. This is an open-access article distributed under the terms of the Creative Commons Attributions licence, which permits unrestricted use, distribution, and reproduction in any medium, but not for commercial gain, provided the original author and source are credited.