Abstract
Objectives
Electromagnetic fields (EMF) are widely used in musculoskeletal disorders. There are indications that EMF might also be effective in the treatment of osteoporosis. To justify clinical follow-up experiments, we examined the effects of EMF on bone micro-architectural changes in osteoporotic and healthy rats. Moreover, we tested the effects of EMF on fracture healing.
Methods
EMF (20 Gauss) was examined in rats (aged 20 weeks), which underwent an ovariectomy (OVX; n = 8) or sham-ovariectomy (sham-OVX; n = 8). As a putative positive control, all rats received bilateral fibular osteotomies to examine the effects on fracture healing. Treatment was applied to one proximal lower leg (three hours a day, five days a week); the lower leg was not treated and served as a control. Bone architectural changes of the proximal tibia and bone formation around the osteotomy were evaluated using in vivo microCT scans at start of treatment and after three and six weeks.
Results
In both OVX and sham-OVX groups, EMF did not result in cancellous or cortical bone changes during follow-up. Moreover, EMF did not affect the amount of mineralised callus volume around the fibular osteotomy.
Conclusions
In this study we were unable to reproduce the strong beneficial findings reported by others. This might indicate that EMF treatment is very sensitive to the specific set-up, which would be a serious hindrance for clinical use. No evidence was found that EMF treatment can influence bone mass for the benefit of osteoporotic patients.
Cite this article: Bone Joint Res 2014;3:230–5.
Article focus
Does EMF treatment induce beneficial trabecular and/or cortical bone changes in osteoporotic or healthy rats?
Does EMF stimulate bone healing in a fracture model in healthy and/or osteoporotic rats?
Might EMF treatment be beneficial for osteoporotic patients?
Key messages
No effect of EMF on trabecular and cortical bone was detected during six-week follow-up.
In the literature there are contradictory findings on the effect of EMF on bone mass.
EMF might be very sensitive to specific set-up, which might impede clinical implementation.
Strengths and limitations
In vivo microCT imaging is a very sensitive method to analyse bone changes in small animals over time.
Both animals which underwent an ovariectomy and healthy animals were examined.
In vivo microCT imaging was the only analysing technique; no histomorphometric or biomechanical analyses were performed.
Introduction
Osteoporosis is a disease characterised by progressive bone loss and deterioration of the micro-architecture leading to an increased risk of fracture. Several pharmaceutical treatments that aim to reduce further bone loss or increase bone mass are available. However, these therapies require a regular and probably lifelong intake, with potential side effects and related high costs. Electromagnetic fields (EMF) are also reported to have a beneficial effect on bone micro-architecture and might reduce the risk of fracture.
EMF were developed based on the finding that electrical currents exist in mechanically-loaded bone and are important for physiological regulation of bone metabolism.1 There is some evidence for the effectiveness of EMF. However, due to the large variation in the characteristics of EMF signals of different generators, comparison of different in vitro and in vivo studies is difficult.
Experimental studies examining the effects of EMF on bone healing in osteotomy gap models have shown the stimulatory effects of EMF on callus formation and mechanical capacities, even when treated for only one hour per day.2-5 The underlying stimulatory mechanisms of electromagnetic fields have been studied in vitro using mesenchymal stem cells, osteoblasts or osteoblast-like cells and are related to BMP-2, TGF-β, IGF-II, prostaglandins, nitric oxide synthase phosphorylation and mitogen-activated protein kinase activation.6-13
Although experimental evidence of the effectiveness of EMF on bone formation seems quite extensive, clinical evidence is lacking. EMF are, for example, widely used for the treatment of nonunion, but their effectiveness is limited to observational studies14-18 and some smaller control-based studies with suboptimal study design.19-21 Therefore, the use of EMF in nonunions is still under debate.22
Few studies have investigated the potential role of EMF treatment for osteoporosis. In 1990, Tabrah et al23 reported a positive effect of EMF on the bone mineral density (BMD) of radii in older women. Furthermore, few animal studies address the topic. Chang and Chang24 showed that whole-body EMF completely prevented OVX-induced trabecular bone loss in rats that were treated for eight hours per day. Jing et al25 also demonstrated preventive effects of EMF on OVX-induced trabecular bone loss using BMD analysis. In another study by Sert et al,26 it was shown that electromagnetic treatment induced an almost two fold increase in cortical bone mass in the tibias of OVX rats. In our previous work, we examined several systemic EMF signals and treatment protocols with the use of in vivo microCT imaging, but we were unable to demonstrate positive effects on bone micro-architecture in OVX rats.27 One difference between this previous study and those that found positive effects is that we used lower magnetic fields, which might explain the lack of success.
Since many in vitro and in vivo studies show strong positive effects of EMF on bone formation, this non-invasive treatment has a potentially important role in the treatment of low bone mass and osteoporosis. To justify clinical follow-up experiments, we examined the effects of 20 Gauss EMF on the bone micro-architecture using in vivo microCT scanning. This is a stronger EMF than we used in our previous paper and is similar to signals that did find anabolic effects.26,27In vivo microCT scanning is a sensitive technique in which both cancellous and cortical bone changes can be analysed in longitudinal and 3D fashion. The effects were examined in both ovariectomised28 and sham-ovariectomised rats. As a putative positive control, a bilateral fibular osteotomy was added to the model to examine the effect of EMF on fresh fracture healing.
Materials and Methods
A total of 16 female Wistar rats were obtained, age 20 weeks (Harlan, the Netherlands). All were housed in the institute’s animal facility with a 12-hour light/dark regime, and all received standard food pellets and water ad libitum. The study protocol was approved by the local Animal Experiments Committee (EUR 687) and was in accordance with Dutch law on animal experimentation.
Research model
Two groups were made three weeks prior to EMF treatment. The rats underwent either a bilateral ovariectomy (OVX) to simulate osteoporosis (n = 8) or a sham-ovariectomy (n = 8). This procedure was performed under sterile conditions using gas anaesthesia (oxygen with 3% isoflurane; Rhodia Organique Fine Ltd, Bristol, UK). Analgesics were given for three days: 0.05 mg/kg/12 hour buprenorphine (Schering-Plough, Kenilworth, New Jersey). In sham-ovariectomy, the procedures were exactly the same, except that the ovaries were only manipulated and not removed.
A bilateral fibula osteotomy was performed in all rats 19 days after (sham-)OVX. It has been shown that EMF stimulated fracture healing using the fibula osteotomy model.5 To include a positive control, this fresh-fracture model was added to our osteoporosis model. In a previous paper, we demonstrated that there is no interaction of OVX on mineralised callus volume and vice versa.29 The hind legs were shaved under general anaesthesia (oxygen/3% isoflurane). Then, under sterile conditions, a 1 cm incision at the lateral side of the calf muscle was made through the skin and fascia. Under microscopic control, the fibula was presented in a blunt manner. At 0.4 cm distal to the fibulo-tibial joint, an osteotomy (including the periosteum) was made using a high-speed mini-saw. The thickness of the osteotomy was the same as that of the saw blade, i.e. 0.1 mm. Fascia and skin were stitched. These rats received analgesics (buprenorphine 0.05 mg/kg/12 hour) for two days.
EMF
EMF treatment was started 21 days after (sham-)OVX and two days after fibular osteotomy. During EMF treatment, the rats were placed in a custom-made harness.
This fixating procedure enabled application of the EMF coil to the hind leg without the use of an anaesthetic. Three weeks prior to the start of EMF treatment, rats were placed in the harness with an increasing time period in order to get acclimatised to the harness.
EMF was given to one hind leg, five days a week for three hours a day. The EMF signal was produced by a custom-made generator producing a 20 Gauss sinusoid waveform at 50 Hz. This is a higher EMF than we used in our previous paper. For technical reasons, this has consequences for the characteristics of the signal (sinusoidal instead of pulse bursts). This higher EMF field is similar to signals that did find anabolic effects.26,27 The coil extended from the knee to the ankle joint.
The other hind leg was left untreated and served as a control. µ-copper foil was placed around the control leg to exclude any effect of the coil on the contra lateral side.
MicroCT scanning
To evaluate bone changes in the proximal tibia, in vivo microCT scans were made at the start of EMF treatment and three and six weeks after EMF treatment was started, so at three, six and nine weeks after (sham-)OVX. Under gas anaesthesia, the hind leg of the rat (from distal femur to the tibial diaphysis) was scanned with an in vivo microCT scanner (Skyscan 1076 Microtomograph, Kontich, Belgium) at a voltage of 60 kV, a current of 167 μA and a 0.5 mm aluminium filter, over 196° with a rotation step of 1°, taking eight minutes per scan.
Using NRecon (NRecon software version 1.5, Skyscan, Kontich, Belgium) three-dimensional reconstructions (isotropic voxelsize of 18 microns) of two regions of interest were made, one at the proximal metaphysis which mainly contains cancellous bone and another at the mid-diaphysis, which contains mainly cortical bone (Fig. 1) three-dimensional reconstructions (isotropic voxelsize of 18 microns) of two regions of interest were made, one at the proximal metaphysis which mainly contains cancellous bone and another at the mid-diaphysis, which contains mainly cortical bone (Fig. 1) of two regions of interest were made, one at the proximal metaphysis which mainly contains cancellous bone and another at the mid-diaphysis, which contains mainly cortical bone (Fig. 1).
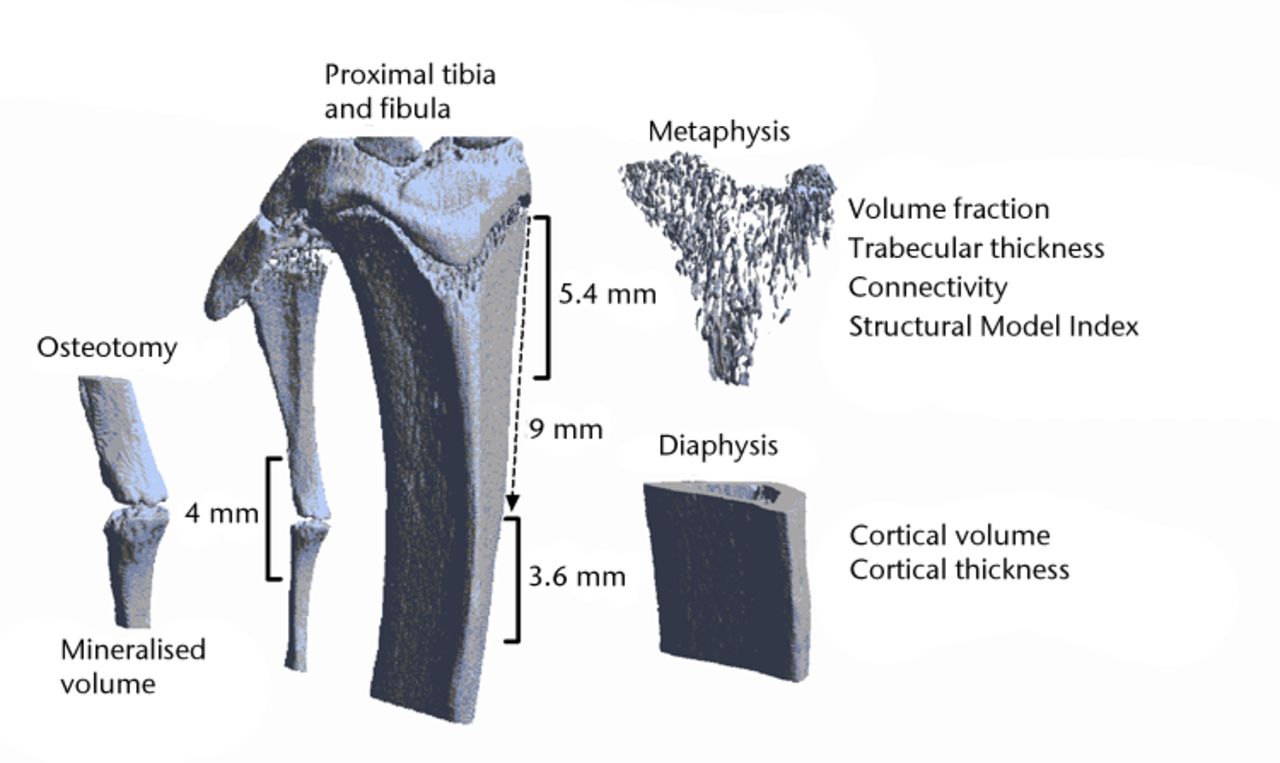
Fig. 1
Three-dimensional reconstruction of a microCT scan of the proximal tibia and the proximal fibula. The analysed regions of interest are indicated.
The reconstruction of the proximal metaphysis was selected manually, starting just distally of the epiphysis and continuing 5.4 mm beyond that. The reconstruction of the diaphysis was defined by a region of 3.6 mm, starting 9 mm distally from the epiphysis. Bony and non-bony structures were separated using a local threshold algorithm (software freely available)30 resulting in binary datasets.31 Cortical and trabecular bone were automatically separated using in-house software. Trabecular architecture in the proximal metaphysis was characterised by determining trabecular volume fraction (BV/TV), connectivity density (Conn/TV), structure model index (SMI) and 3D trabecular thickness (TbTh). Cortical architecture was assessed in the diaphysis and was characterised by cortical volume (CtV) and cortical thickness (CtTh).
Analysis of the mineralised callus formation at the fibular osteotomy was performed on the same microCT scans. In reconstructed datasets, the osteotomy site was selected and an area of 2 mm proximal and 2 mm dorsal to the fracture was selected for further analysis (Fig. 1).
Because the degree of mineralistion within the callus site varied, a global threshold was used. The threshold was set in such a way that the cortical bone visually included the selection; in this way the mineralised callus volume was measured.
Statistical analysis
Differences between the treated legs and the untreated control legs were statistically analysed for each time point using paired t-tests for all parameters (GraphPad Software, San Diego, California). A p-value < 0.05 was considered statistically significant.
Results
Due to complications during sham-OVX, one rat died. In addition, because the fibular osteotomy resulted in additional fractures in the fibular, two additional animals were excluded from analyses of the mineralised callus volume.
At the start of EMF, the mean treatment body weight in sham-OVX rats was 251.3 g (sd 12.0) and the mean increase in body weight was 10.7 g (sd 2.9). In OVX rats, the mean body weight was 299.9 g (sd 15.0) at start of EMF treatment and the mean increase in the six-week follow-up period was 14.1 g (sd 10.3). None of the rats experienced weight loss during follow-up.
In sham-OVX rats, the BV/TV in non-treated control tibias was 27.7% (sd 2.8), 26.9% (sd 3.0) and 25.3% (sd 3.1) at weeks 0, three and six, respectively; this was not significantly different from EMF-treated tibias (Fig. 2a).
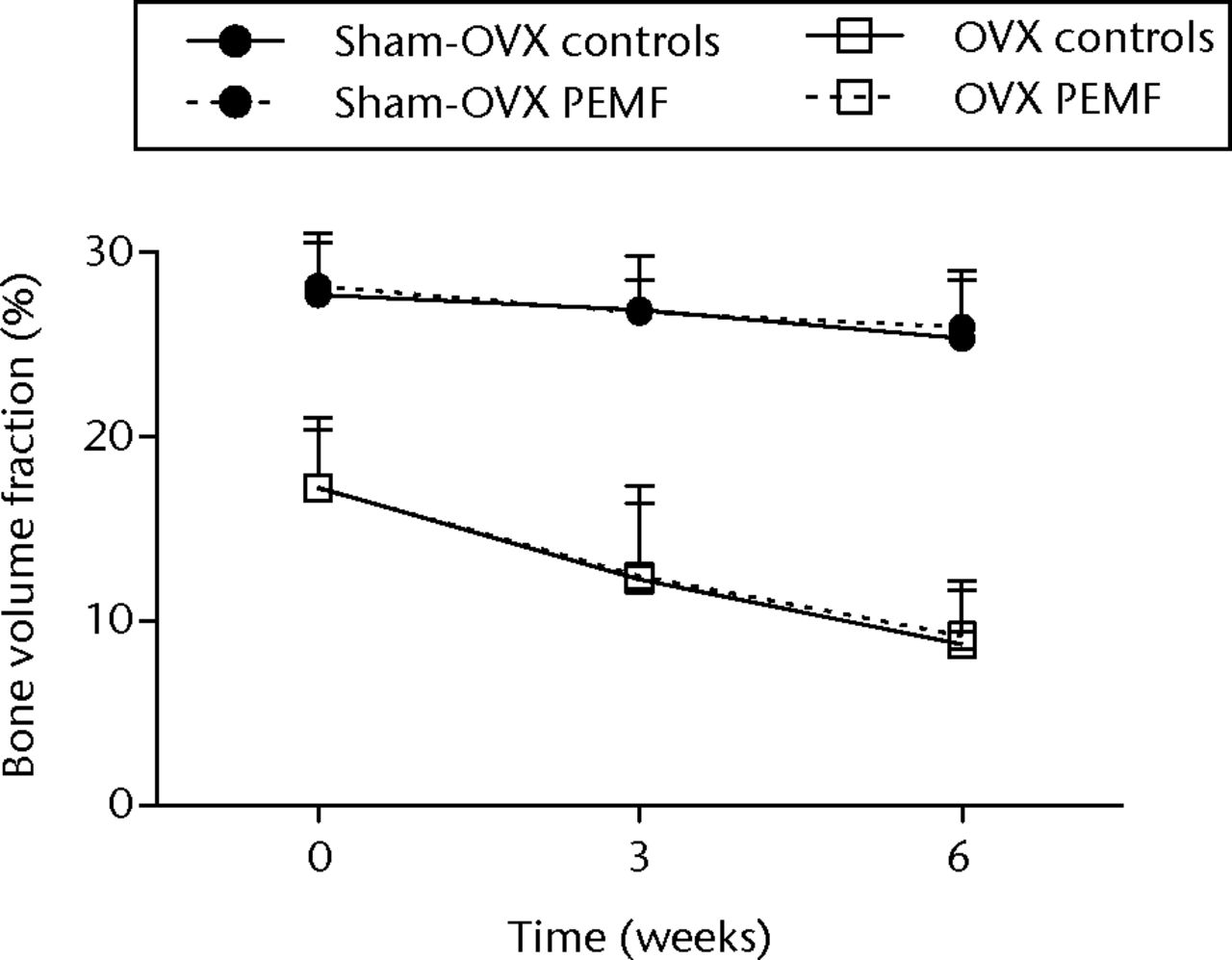
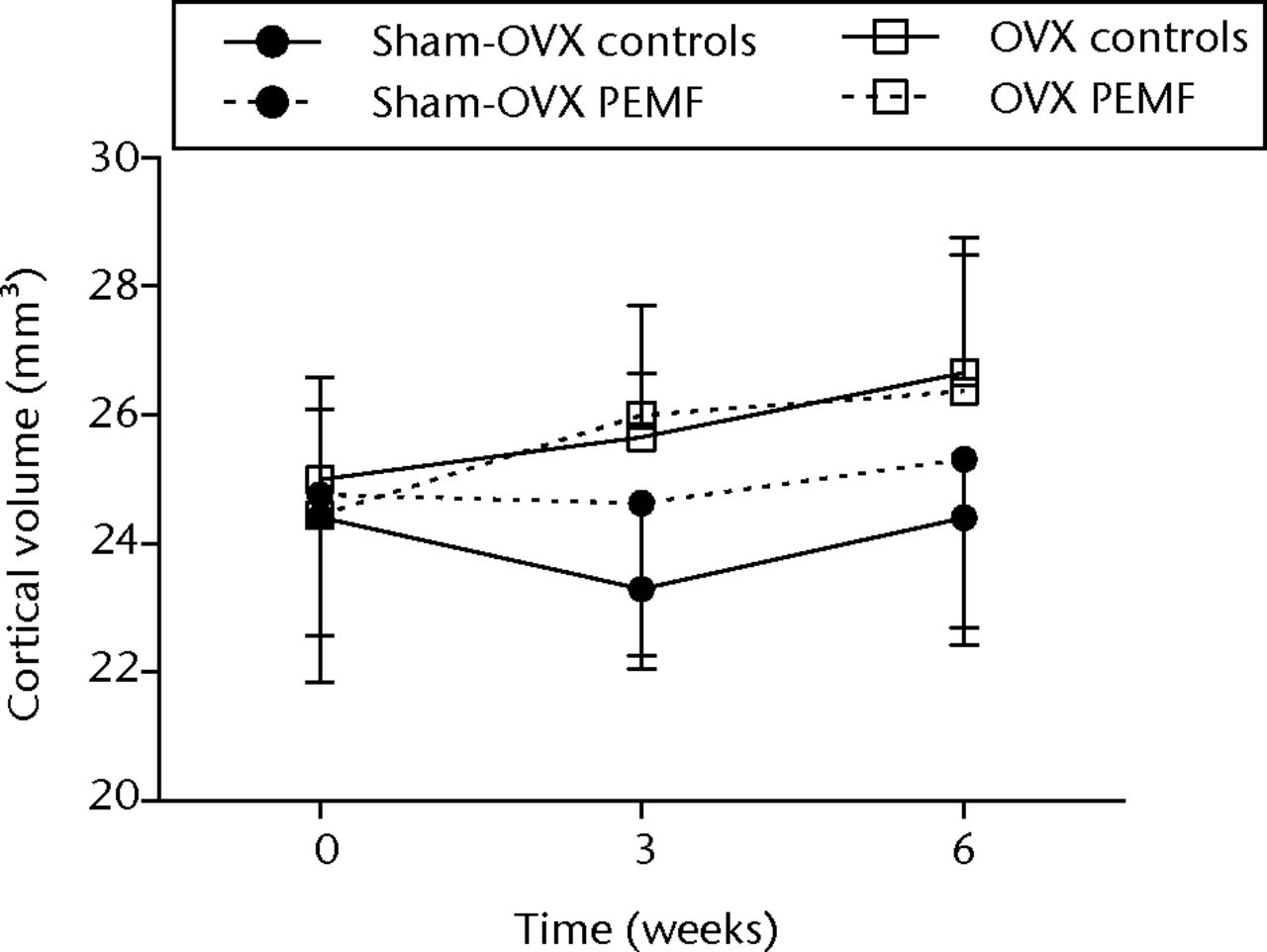
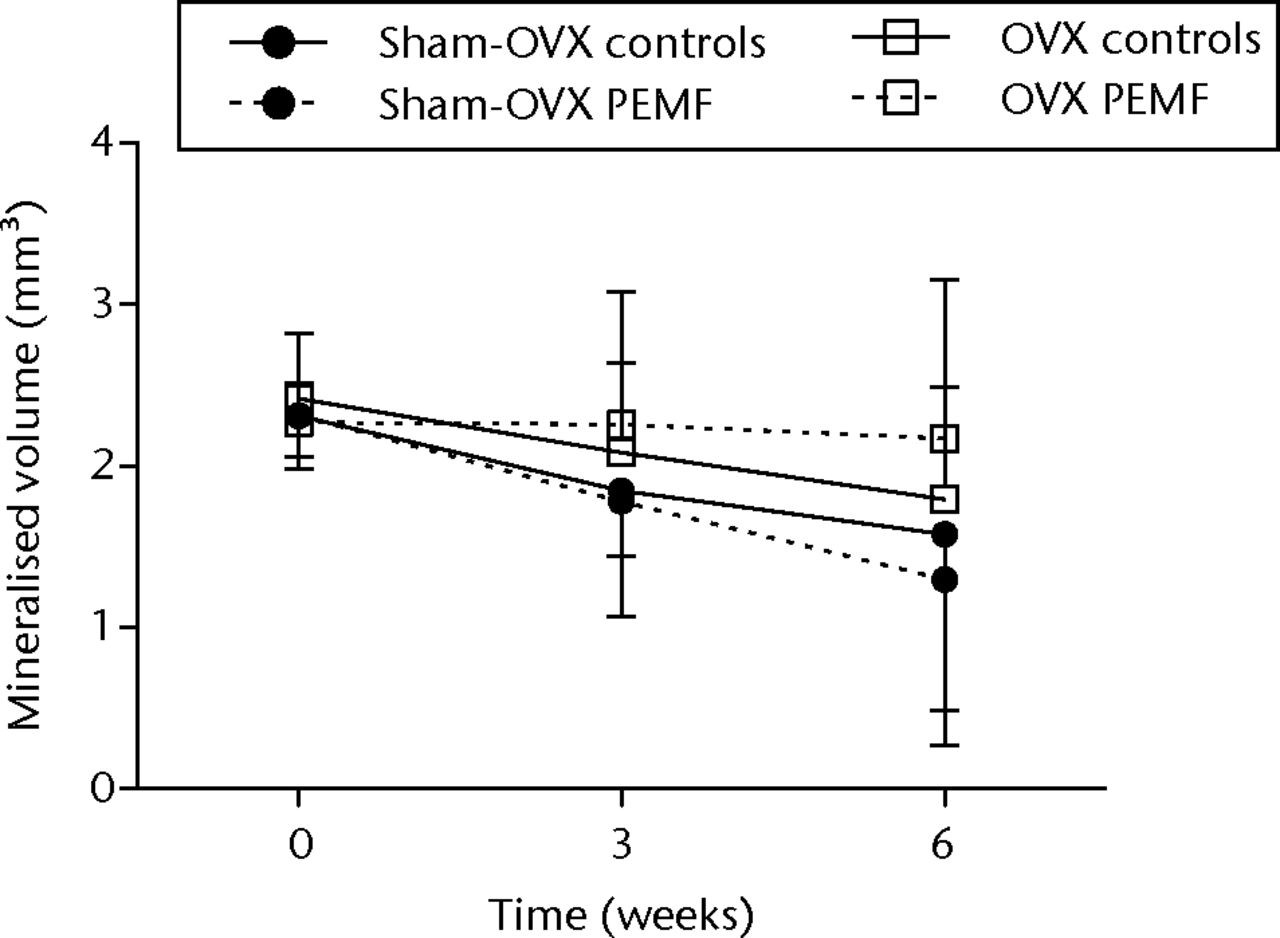
Figs. 2a - 2c
Graphs showing a) trabecular volume fractions, b) cortical volume and c) mineralised callus volume of electromagnetic field-treated and non-treated control tibias in sham-ovariectomised (n = 7) and in ovariectomised (n = 8) rats. Data are presented as mean values and standard deviations.
In sham-OVX rats, no differences were observed in morphometric or cortical parameters between non-treated control tibias and EMF-treated tibias at three and six weeks after the start of treatment (Fig. 2b, Table I).
Table I
Bone changes in the tibia of non-treated tibias and electromagnetic field (EMF)-treated tibias of sham-ovariectomised rats. Mean values with standard deviations and p-value (paired t-tests) are given (n = 7).
Week three | Week six | ||||||
---|---|---|---|---|---|---|---|
Controls | EMF | p-value | Controls | EMF | p-value | ||
Trabecular volume fraction (BV/TV)(%) | 26.9 (3.0) | 26.8 (1.7) | 0.42 | 25.3 (3.1) | 25.9 (3.1) | 0.68 | |
Mean trabecular thickness (µm) | 106.7 (5.8) | 108 (5.0) | 0.19 | 108.7 (4.1) | 109.6 (6.0) | 0.74 | |
Connectivity density (mm3) | 75.4 (5.1) | 69.5 (10.5) | 0.14 | 63.7 (7.7) | 52.0 (10.5) | 0.28 | |
Structure model index | 1.85 (0.11) | 1.83 (0.18) | 0.15 | 1.87 (0.15) | 1.81 (0.24) | 0.33 | |
Cortical volume (mm3) | 23.3 (1.2) | 24.6 (2.4) | 0.3 | 24.4 (2.0) | 25.3 (2.6) | 0.27 | |
Cortical thickness (µm) | 458.5 (11.1) | 469.7 (20.4) | 0.74 | 476.6 (18.4) | 487.4 (30.0) | 0.44 |
Mineralised callus formation at the osteotomy site showed a wide variation between and within rats. The average mineralised callus volume decreased during follow-up in both the controls and the EMF-treated tibias but the differences were not significant (Fig. 2c).
In OVX rats, the BV/TV in non-treated control tibias was 17.2% (sd 3.2), 12.3% (sd 5.1) and 8.7% (sd 3.0) at weeks 0, three and six, respectively (Fig. 2a).
BV/TV and morphometric parameters showed no significant differences from EMF-treated tibias at any time point (Table II).
Table II
Bone changes in the tibia of non-treated tibias and electromagnetic field (EMF)-treated tibias of ovariectomised rats. Mean values with standard deviations and p-value (pairedt-tests) are given (n = 8)
Week Three | Week Six | ||||||
---|---|---|---|---|---|---|---|
Controls | EMF | p-value | Controls | EMF | p-value | ||
Trabecular volume fraction BV/TV (%) | 12.3 (5.1) | 12.4 (3.9) | 0.78 | 8.7 (3.0) | 9.2 (3.0) | 0.85 | |
Mean trabecular thickness (µm) | 101.0 (6.3) | 102.6 (5.74) | 0.27 | 100.3 (3.8) | 101.7 (4.8) | 0.66 | |
Connectivity density (mm3) | 16.9 (13.4) | 17.1 (10.4) | 0.71 | 8.5 (7.5) | 8.7 (7.0) | 0.68 | |
Structure model index | 2.50 (0.20) | 2.48 (0.18) | 0.91 | 2.62 (0.16) | 2.58 (0.17) | 0.38 | |
Cortical volume (mm3) | 25.7 (1.0) | 26.0 (1.7) | 0.54 | 26.7 (1.8) | 26.4 (2.4) | 0.41 | |
Cortical thickness (µm) | 469.2 (22.0) | 477.9 (23.8) | 0.45 | 504.0 (28.4) | 495.8 (27.5) | 0.24 |
CtV in non-treated control tibias was 25.0 (sd 1.6), 25.7 (sd 1.0) and 26.7 (sd 1.8) at 0, three and six weeks, respectively. Neither CtV nor CtTh were significantly different from that in EMF-treated tibias (Fig. 2b, Table II).
Mineralised callus formation showed a wide variation; there were no significant differences between controls and EMF-treated tibias (Fig. 2c).
Discussion
This study examines the effects of EMF on changes in both cancellous and cortical bone to establish whether EMF might serve as a treatment for osteoporosis. Although in vivo microCT scanning allowed us to follow morphometric bone changes in high detail over time, we were unable to detect any differences between EMF-treated and untreated legs.
There are many indications from in vitro studies that EMF might have beneficial effects on the bone architecture for the benefit of those suffering with osteoporosis. However, few animal experiments using ovariectomised animals exist. Chang and Chang24 showed that OVX-induced trabecular bone loss was prevented by EMF treatment using histomorphometric analyses at 30 days of treatment. EMF was given with a single pulse at 7.5 Hz and a maximum magnetic field of 8 Gauss for eight hours per day in the whole animal. In another study using the OVX rat model, whole-body EMF led to an increase of 71% in cortical thickness compared with non-treated controls.26 EMF was applied for four hours a day with a 50 Hz sinusoidal waveform of 10 Gauss. In our previous work, we could not demonstrate positive effects on cortical or trabecular bone when OVX rats were treated with systemic EMF.27 In that study, different EMF signals, including those with both pulse bursts and single pulses, were examined, however, the magnetic field of 1 G was lower than the studies of Chang and Chang24 and of Sert et al.26 In the current study we therefore used a EMF signal with 20 G. Although the characteristics of EMF used in previous studies by others and in the current paper are comparable, especially between the paper of Sert et al26 and the current study, we were unable to confirm the pronounced effects of EMF on cancellous or cortical bone. These contradictory findings suggest that the effects of EMF on osteoporosis are very sensitive to the specific experimental set-up used. This is confirmed in a more recent paper in which ovariectomised rats that received EMF during the day showed a higher BMD than those receiving EMF at night.25 The cause of this difference is unknown.
Although many pre-clinical experiments show positive effects of EMF on cortical and trabecular bone, it has not resulted in many clinical studies. Tabrah et al23 found that the BMD of both the treated and the opposite untreated radius of women prone to osteoporosis increased during a 12-week period, suggesting that a systemic effect might be induced by EMF that influenced BMD. However, this study has never been repeated in a randomised controlled trial. According to a review in 2008, more clinical studies are described in Chinese literature.32 These data suggest that the effects of EMF on BMD in osteoporosis are controversial.
In contrast to other studies, we examined the effects of locally applied EMF on osteoporosis. As a putative control we added a fracture model to that of osteoporosis. We demonstrated earlier that there is no interaction between the OVX and the fracture model.29 It has been shown that EMF stimulate fracture healing at the fibular osteotomy.5 As in the study of Midura et al,5 we found large variations in mineralised callus volume. However, the amount of nonunions, characterised by progressive gap size due to resorption of the bony ends was much higher in the current study. In our hands, 11 of the 30 fibular osteotomies resulted in a nonunion, irrespective of the study group. We speculate that (micro-)movement is the cause of the large number of failures. Although the EMF signal was highly comparable with that of Midura et al5 (both maximum electrical field of 20 Gauss, treated for three hours a day), we were unable to confirm the stimulating effects of EMF on fresh fracture healing.
A limitation of the current study is that only bone micro-architecture was analysed during follow-up and no mechanical testing or histology was performed. Regarding histological parameters, it is still possible that EMF affected bone turnover, without inducing changes in bone architecture.
In conclusion, we could not confirm any positive effects of EMF for the treatment of osteoporosis or for fracture healing. It is also possible that EMF works under specific circumstances, but that its effects are highly sensitive to the precise set-up, e.g. signal characteristics (including exact waveform of the electromagnetic field), timing of the EMF treatment, and animal strain. This would be a serious hindrance to clinical use. However, the present findings provide no evidence to support EMF as a beneficial treatment for osteoporotic patients.
1 Fukada E , YasudaI. On the piezoelectric effect of bone. J Phys Soc1957;12:1158–1162. Google Scholar
2 Fredericks DC , NepolaJV, BakerJT, AbbottJ, SimonB. Effects of pulsed electromagnetic fields on bone healing in a rabbit tibial osteotomy model. J Orthop Trauma2000;14:93–100.CrossrefPubMed Google Scholar
3 Ibiwoye MO , PowellKA, GrabinerMD, et al.Bone mass is preserved in a critical-sized osteotomy by low energy pulsed electromagnetic fields as quantitated by in vivo micro-computed tomography. J Orthop Res2004;22:1086–1093.CrossrefPubMed Google Scholar
4 Inoue N , OhnishiI, ChenD, et al.Effect of pulsed electromagnetic fields (PEMF) on late-phase osteotomy gap healing in a canine tibial model. J Orthop Res2002;20:1106–1114.CrossrefPubMed Google Scholar
5 Midura RJ , IbiwoyeMO, PowellKA, et al.Pulsed electromagnetic field treatments enhance the healing of fibular osteotomies. J Orthop Res2005;23:1035–1046.CrossrefPubMed Google Scholar
6 Aaron RK , WangS, CiomborDM. Upregulation of basal TGFbeta1 levels by EMF coincident with chondrogenesis--implications for skeletal repair and tissue engineering. J Orthop Res2002;20:233–240.CrossrefPubMed Google Scholar
7 Bodamyali T , BhattB, HughesFJ, et al.Pulsed electromagnetic fields simultaneously induce osteogenesis and upregulate transcription of bone morphogenetic proteins 2 and 4 in rat osteoblasts in vitro. Biochem Biophys Res Commun1998;250:458–461.CrossrefPubMed Google Scholar
8 Fitzsimmons RJ , RyabyJT, MohanS, MageeFP, BaylinkDJ. Combined magnetic fields increase insulin-like growth factor-II in TE-85 human osteosarcoma bone cell cultures. Endocrinology1995;136:3100–3106.CrossrefPubMed Google Scholar
9 Guerkov HH , LohmannCH, LiuY, et al.Pulsed electromagnetic fields increase growth factor release by nonunion cells. Clin Orthop Relat Res2001;384:265–279.CrossrefPubMed Google Scholar
10 Nie K , HendersonA. MAP kinase activation in cells exposed to a 60 Hz electromagnetic field. J Cell Biochem2003;90:1197–1206.CrossrefPubMed Google Scholar
11 Schnoke M , MiduraRJ. Pulsed electromagnetic fields rapidly modulate intracellular signaling events in osteoblastic cells: comparison to parathyroid hormone and insulin. J Orthop Res2007;25:933–940.CrossrefPubMed Google Scholar
12 Schwartz Z , SimonBJ, DuranMA, et al.Pulsed electromagnetic fields enhance BMP-2 dependent osteoblastic differentiation of human mesenchymal stem cells. J Orthop Res2008;26:1250–1255.CrossrefPubMed Google Scholar
13 Wang Z , ClarkCC, BrightonCT. Up-regulation of bone morphogenetic proteins in cultured murine bone cells with use of specific electric fields. J Bone Joint Surg [Am]2006;88-A:1053–1065.CrossrefPubMed Google Scholar
14 Adams BD , FrykmanGK, TaleisnikJ. Treatment of scaphoid nonunion with casting and pulsed electromagnetic fields: a study continuation. J Hand Surg Am1992;17:910–914.CrossrefPubMed Google Scholar
15 Gossling HR , BernsteinRA, AbbottJ. Treatment of ununited tibial fractures: a comparison of surgery and pulsed electromagnetic fields (PEMF). Orthopedics1992;15:711–719.CrossrefPubMed Google Scholar
16 Holmes GB Jr . Treatment of delayed unions and nonunions of the proximal fifth metatarsal with pulsed electromagnetic fields. Foot Ankle Int1994;15:552–556.CrossrefPubMed Google Scholar
17 Madroñero A , PitillasI, MansoFJ. Pulsed electromagnetic field treatment failure in radius non-united fracture healing. J Biomed Eng1988;10:463–466.CrossrefPubMed Google Scholar
18 Punt BJ , Den HoedPT, FontijneWPJ. Pulsed electromagnetic fields in the treatment of nonunion. Eur J Orthop Surg Traumatol2008;18:127–133. Google Scholar
19 Barker AT , DixonRA. Pulsed electromagnetic fields. J Bone Joint Surg [Br]1991;73-B:352–354.CrossrefPubMed Google Scholar
20 Sharrard WJ . A double-blind trial of pulsed electromagnetic fields for delayed union of tibial fractures. J Bone Joint Surg [Br]1990;72-B:347–355.CrossrefPubMed Google Scholar
21 Simonis RB , ParnellEJ, RayPS, PeacockJL. Electrical treatment of tibial non-union: a prospective, randomised, double-blind trial. Injury2003;34:357–362.CrossrefPubMed Google Scholar
22 Griffin XL, Costa ML, Parsons N, Smith N. Electromagnetic field stimulation for treating delayed union or non-union of long bone fractures in adults. Cochrane Database Syst Rev 2011;4:CD008471. Google Scholar
23 Tabrah F , HoffmeierM, GilbertF Jr, BatkinS, BassettCA. Bone density changes in osteoporosis-prone women exposed to pulsed electromagnetic fields (PEMFs). J Bone Miner Res1990;5:437–442.CrossrefPubMed Google Scholar
24 Chang K , ChangWH. Pulsed electromagnetic fields prevent osteoporosis in an ovariectomized female rat model: a prostaglandin E2-associated process. Bioelectromagnetics2003;24:189–198.CrossrefPubMed Google Scholar
25 Jing D , ShenG, HuangJ, et al.Circadian rhythm affects the preventive role of pulsed electromagnetic fields on ovariectomy-induced osteoporosis in rats. Bone2010;46:487–495.CrossrefPubMed Google Scholar
26 Sert C , MustafaD, DüzMZ, AkşenF, KayaA. The preventive effect on bone loss of 50-Hz, 1-mT electromagnetic field in ovariectomized rats. J Bone Miner Metab2002;20:345–349.CrossrefPubMed Google Scholar
27 van der Jagt OP , van der LindenJC, WaarsingJH, VerhaarJA, WeinansH. Systemic treatment with pulsed electromagnetic fields do not affect bone microarchitecture in osteoporotic rats. Int Orthop2012;36:1501–1506. Google Scholar
28 Kalu DN . The ovariectomized rat model of postmenopausal bone loss. Bone Miner1991;15:175–191.CrossrefPubMed Google Scholar
29 van der Jagt OP , van der LindenJC, SchadenW, et al.Unfocused extracorporeal shock wave therapy as potential treatment for osteoporosis. J Orthop Res2009;27:1528–1533.CrossrefPubMed Google Scholar
30 No authors listed. Erasmus MC: Erasmus Orthopaedic Research Laboratory, 2014. http://www.erasmusmc.nl/47460/386156/Downloads (date last accessed 11 March 2014). Google Scholar
31 Waarsing JH , DayJS, WeinansH. An improved segmentation method for in vivo microCT imaging. J Bone Miner Res2004;19:1640–1650.CrossrefPubMed Google Scholar
32 Huang LQ , HeHC, HeCQ, ChenJ, YangL. Clinical update of pulsed electromagnetic fields on osteoporosis. Chin Med J (Engl)2008;121:2095–2099.PubMed Google Scholar
Funding statement:
J. C. van der Linden reports receipt of a personal Veni grant from the Dutch Technology Foundation STW (RPG 6294) which is related to this article.
Author contributions:
O. P. van der Jagt: Study design, Performed surgeries, Data collection and analysis, Writing the paper
J. C. van der Linden: Study design, Data analysis, Writing the paper
J. H. Waarsing: Study design, Data analysis, Writing the paper
J. A. N. Verhaar: Study design, Writing the paper
H. Weinans: Study design, Writing the paper
ICMJE Conflict of Interest:
None declared
©2014 The British Editorial Society of Bone & Joint Surgery. This is an open-access article distributed under the terms of the Creative Commons Attributions licence, which permits unrestricted use, distribution, and reproduction in any medium, but not for commercial gain, provided the original author and source are credited.
Supplementary material. A photograph showing a rat hanging in a custom-made harness is available with the online version of this paper at www.bjr.boneandjoint.org.uk.