Abstract
Objectives
The purpose of this study was to refine an accepted contaminated rat femur defect model to result in an infection rate of approximately 50%. This threshold will allow examination of treatments aimed at reducing infection in open fractures with less risk of type II error.
Methods
Defects were created in the stablised femurs of anaethetised rats, contaminated with Staphylococcus aureus and then debrided and irrigated six hours later. After 14 days, the bone and implants were harvested for separate microbiological analysis. This basic model was developed in several studies by varying the quantity of bacterial inoculation, introducing various doses of systemic antibiotics with and without local antibiotics.
Results
The bacterial inoculation associated with a 50% infection rate was established as 1 × 102 colony forming units (CFU). With an initial bacterial inoculum of 1 × 105 CFU, the dose of systemic antibiotics associated with 50% infection was 5 mg/Kg of cafazolin injected sub-cutaneously every 12 hours, starting at the time of the first debridment and continuing for 72 hours (seven doses). The systemic dose of cafazolin was lowered to 2 mg/Kg when antibiotic polymethyl methacrylate beads were used concurrently with the same amount of bacterial inoculation.
Conclusion
This model of open fracture infection has been further refined with potential for local and systemic antibiotics. This is a versatile model and with the concepts presented herein, it can be modified to evaluate various emerging therapies and concepts for open fractures.
Cite this article: Bone Joint Res 2014;3:187–92.
Article focus
This paper details our institution’s experience with refining a previously described contaminated rat femur defect model to give a reliable 50% infection rate with no antibiotics, systemic antibiotics, or systemic with local antibiotics.
Key messages
Details of a rodent model of a contaminated open fracture are presented.
Specifically, the quantity of bacterial inoculum and the doses of systemic antibiotic (both used singularly and in combination with local antibiotics) to produce a 50% infection rate in this model are defined.
Variables such as bacterial inoculum and dose of systemic antibiotics can be changed to create an environment where different concepts and emerging therapies can be evaluated.
Within the limits of animal welfare and practicality, our results demonstrate the versatility, reproducibility, and functionality of this model to effectively examine clinically relevant interventions.
Strengths and limitations
-
Strength: these studies detail the ability of this model to be adapted to a variety of research questions in the evaluation of treatments of infection in open fractures.
-
Limitation: the open-fracture rat model used in this study mimics clinical practice, but does not re-create the clinical reality of open fractures.
Introduction
Infection following open fracture remains a significant challenge to orthopaedic surgeons.1,2 Clinical studies of factors that affect infection are difficult. Patient variables and heterogeneity of wounds and fractures all risk confounding clinical research findings. To overcome these problems, large numbers of patients need to be recruited, but it can take many years to report their findings.3 Alternatively, animal models of open fractures offer the potential to test concepts and novel treatments in a reproducible, standardised setting. Variables, other than those under investigation, can be controlled and results obtained rapidly. Although there is a compromise between reality and reproducibility, they allow testing of interventions not sanctioned for clinical trials and save research money for the pursuit of only the most promising therapies. Furthermore, there is a versatility in animal models that allows rapid progress in developing themes as well as the direct comparison of multiple treatment modalities. This paper describes our institution’s experience with a rat-contaminated open femur fracture model. This is a versatile, reproducible model for testing interventions in infection of an open fracture.
Materials and Methods
The model used in these studies is based on that first described by Chen, Kidder and Lew4 in 2002. These studies were conducted under a protocol in compliance with the Animal Welfare Act and the implementing Animal Welfare Regulations, and in accordance with the principles of the Guide for the Care and Use of Laboratory Animals.
Surgical procedure
Adult male Sprague-Dawley rats (Harlan Laboratories, Indianapolis, Indiana) weighing between 350 g and 400 g on the day of surgery, were anesthetised with 2% isofluorane, the fur over their right leg shaved, and the skin sterilised with povidone iodine and 70% isopropanol. An incision is then made in the skin originating over the greater trochanter and continuing distally toward the knee, centred over the femoral shaft. The femoral shaft is then exposed and stabilised with a bespoke contoured polyoxymethylene plate, secured with six threaded K-wires inserted under power (Fig. 1). This allows the humane stabilisation of a bony defect and has the effect of introducing an implant to lower the bacterial inoculum required for infection.5 A 6 mm critical defect is then created in the mid-shaft with a reticulating saw (Series 1000, Microaire, Charlottesville, Virginia) with continuous saline irrigation. The defect is then contaminated with 30 mg of sterile bovine collagen soaked with a specified amount of Staphylococcus aureus (S. aureus) in 0.5 ml of saline. The (Xenogen 36) strain of S. aureus used derives from ATCC strain 49525, originally from a septic human patient (Caliper LifeSciences, Santa Clara, California) and has been engineered to emit photons. This strain of bacteria has been accepted by other investigators to be an appropriate choice for modelling musculoskeletal infection.6 The wound is then closed, with continuous nylon sutures to the fascial layer and surgical clips to skin.
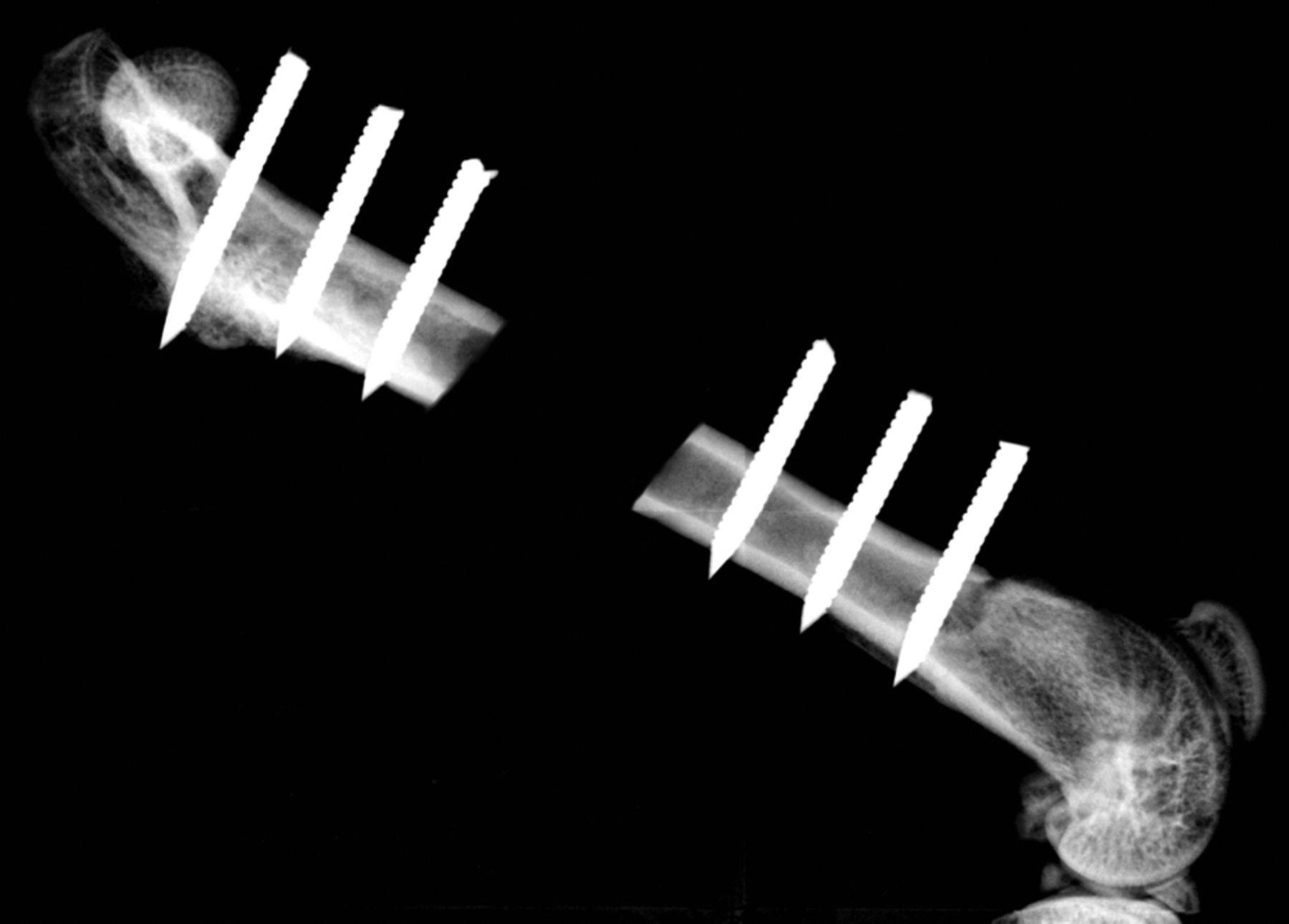
Fig. 1
Radiograph showing a 6 mm defect in a rat femur stabilised by a radiolucent polyoxymethylene plate secured with six threaded 0.9 mm K-wires.
A total of six hours after the original procedure, all animals are re-anesthetised, their wounds are opened, and all the contaminated collagen is meticulously removed with excision of any necrotic or otherwise non-viable soft tissue. The wound is then irrigated with 60 ml of sterile saline delivered at low pressure from a hand-held syringe held 10 cm from the surgical field. At this stage, local treatments, if applicable, were applied and their wounds similarly closed in layers. The animals recovered with appropriate provision of adequate analgesia, food and water, and they were mobilised without restriction of activity. The animals were euthanised 14 days after simulated injury. Under sterile conditions, the wounds were opened through the healed incision with exposure of the bone defect. The femur and hardware were stripped of soft tissue and separated. The bone tissue was snap-frozen in liquid nitrogen and crushed. Bone and hardware samples were then sent separately for standard quantitative microbiological analysis. Briefly, crushed bone samples were homogenised with 10 ml of saline in an agitator. Similarly, hardware specimens were rinsed with 10 ml of saline in an agitator; then aliquots from individual specimens were sequentially diluted and spread onto tryptic-soy-agar plates. After overnight incubation at 37°C, bacterial colonies were counted and recorded, the threshold of detectability was set at 30 colony forming units per gram (CFU/g). Confirmation that the bacterial colonies being counted are from the original contaminating S. aureus is obtained with further imaging using a photon count camera.
Outcome measures
The outcome measures were the presence and quantity of bacteria on the bone and hardware samples, normalised for the mass of the sample processed. For the purposes of this paper, the term ‘infection’ was used to describe an animal on which bacteria were detectable.
Bacterial inoculation
To determine the relationship between initial bacterial inoculation and infection in this model, study groups of ten animals each were inoculated with bacteria at a range of concentrations from 1 × 101 CFU to 1 × 105 CFU, in graduations of single orders of magnitude.
Systemic antibiotics
In animals inoculated with 1 × 105 CFU of S. aureus, sub-cutaneous cefazolin was administered at the time of debridment and at 12 hourly intervals for 3 days, at a total of 7 doses. Surgical and antibiotic treatment was initiated at six hours in study groups of six animals, with a range of antibiotic doses calculated according to an assumed animal weight of 400 g.
Systemic and local antibiotics
Antibiotic polymethylmethacrylate (PMMA) beads were manufactured under sterile conditions using Palacos R (Haraeus, Wehrheim, Germany), arthroplasty cement. 40 g of PMMA co-polymer powder was blended with 2.0 g of vancomycin (Sigma-Aldrich, St Louis, Missouri) and 2.4 g of tobramycin sulphate (Sigma-Aldrich), then mixed with 20 ml of PMMA monomer liquid. A 3 mm mould was then used to create beads weighing approximately 20 mg and containing 3.3% vancomycin and 4% tobramycin sulphate by weight. In this study, all six animals in each study group were contaminated with 1 × 105 CFU of S. aureus and treated with four beads in the wound: two in the bone defect, and two in the surrounding soft tissues; this was the number of beads that reasonably ‘fit’ into the wound. This delivered a dose of 2.5 mg of vancomycin and 2.9 mg of tobramycin into the wound. All of the animals in this work that were surgically treated at six hours, had bacteria recoverable from their wounds 14 days later.
Statistical analysis
The categorical data regarding the presence of bacteria in the bone and on the hardware from study groups were compared using Fisher’s exact test. The quantity of bacteria in the bone and on the hardware for each animal was added up and the log transformed before the mean was found for each group. Pairs of groups can then be directly compared using a Mann–Whitney test, or analysis of variance of results across a range of groups was calculated using a Student Newman–Kuells test.
Results
Bacterial inoculation
Bacteria were not detectable in the 10 male Sprague-Dawley rats that were inoculated with 1 × 101 CFU, while all 10 rats inoculated with 1 × 103 and greater had bacteria detectable on both hardware and bone samples (Fig. 2). In the 10 rats inoculated with 1 × 102, approximately half of the animals had detectable bacteria. This group had a mean bacteria level of several hundred CFU/g while all groups initially inoculated with more bacteria had similar amounts of bacteria in orders of magnitude greater than these levels (Fig. )andTable I).When the model is inoculated with 1 × 102 CFU, bacteria are recoverable from approximately 50% of animals at 14 days.
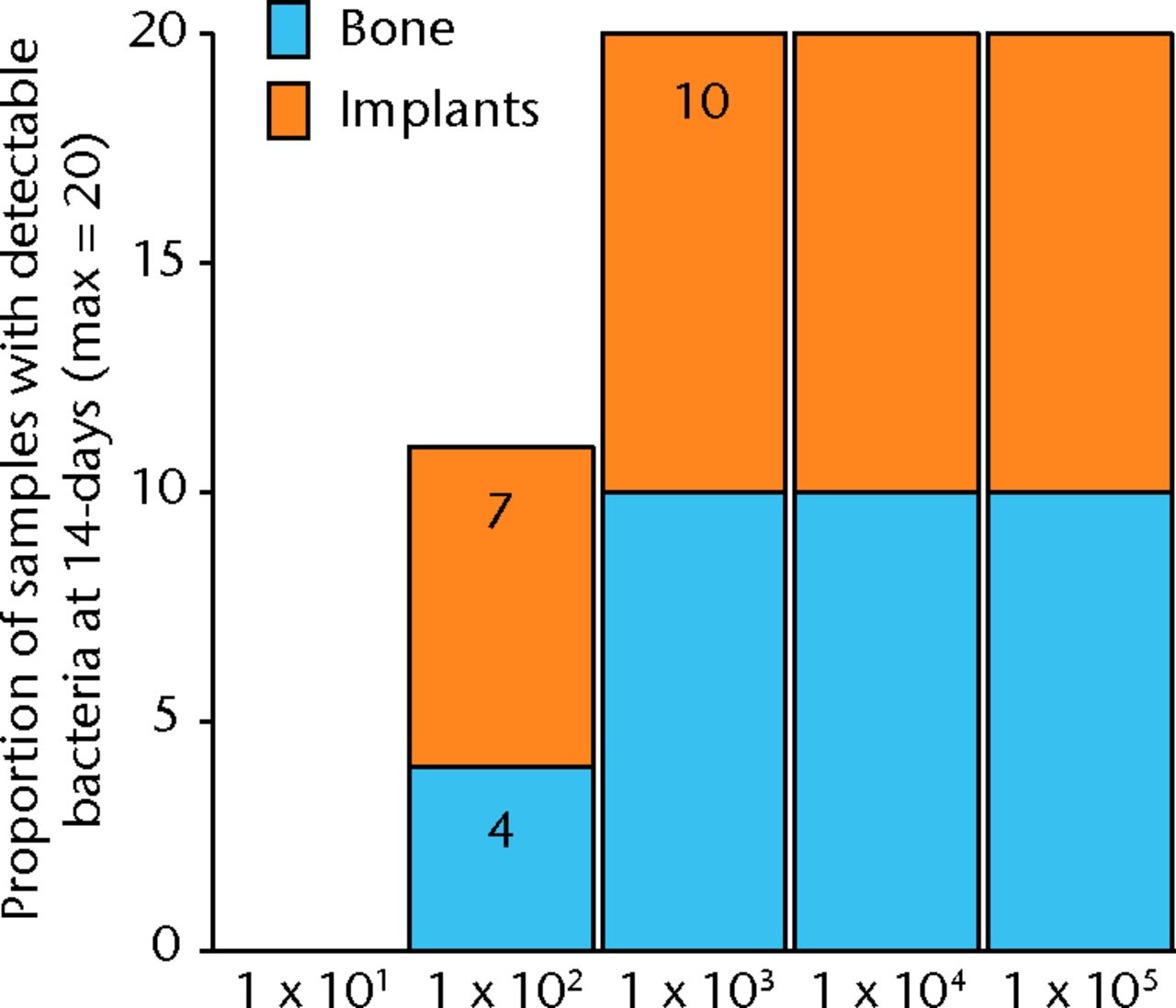
Fig. 2
Graph showing the proportion of bone or implant samples with detectible bacteria in each group of 10 animals (maximum of 20 samples per group) 14 days after inoculation with various quantities of bacteria, given in colony-forming units (CFUs).
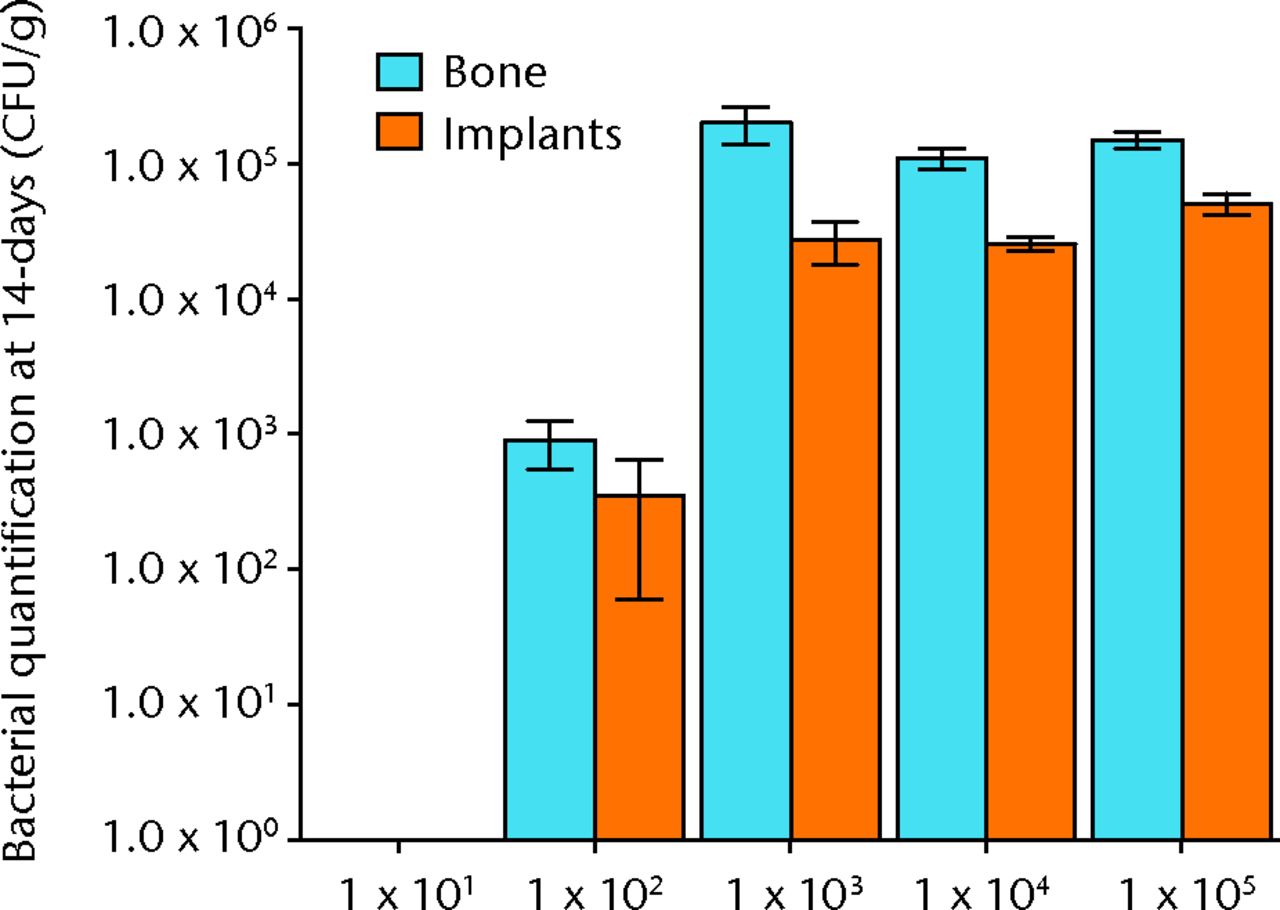
Fig. 3
Graph showing the quantification of bacteria recovered from animals 14 days after inoculation with various quantities of bacteria, given in colony-forming units (CFUs). Error bars show the standard error of the mean.
Table I
Quantification of bacteria recovered from animals 14 days after inoculation with various quantities of bacteria, given in colony forming units (SEM, standard error of the mean)
Quantity of colony-forming units | |||||
---|---|---|---|---|---|
1 × 101 | 1 × 102 | 1 × 103 | 1 × 104 | 1 × 105 | |
Bone mean | 0 | 7.21 × 104 | 7.83 × 105 | 1.11 × 105 | 1.51 × 105 |
Bone SEM | 0 | 4.40 × 104 | 1.35 × 105 | 1.92 × 104 | 1.95 × 104 |
Implant mean | 0 | 9.82 × 104 | 1.65 × 105 | 2.58 × 104 | 5.11 × 104 |
Implant SEM | 0 | 5.10 × 104 | 1.61 × 105 | 3.16 × 103 | 9.11 × 103 |
Systemic antibiotics
All samples in animals treated with 2 mg/Kg were infected, and no animals treated with 10 mg/kg of cefazolin had any bacteria detectible on their bone or hardware. In the study group treated with 5 mg/Kg of cefazolin, approximately half of the animals (6/12) had samples positive for bacteria (Fig. 4). The relationship between bacterial inoculation and subsequent bacterial quantification is shown in (Fig. )andin Table II).
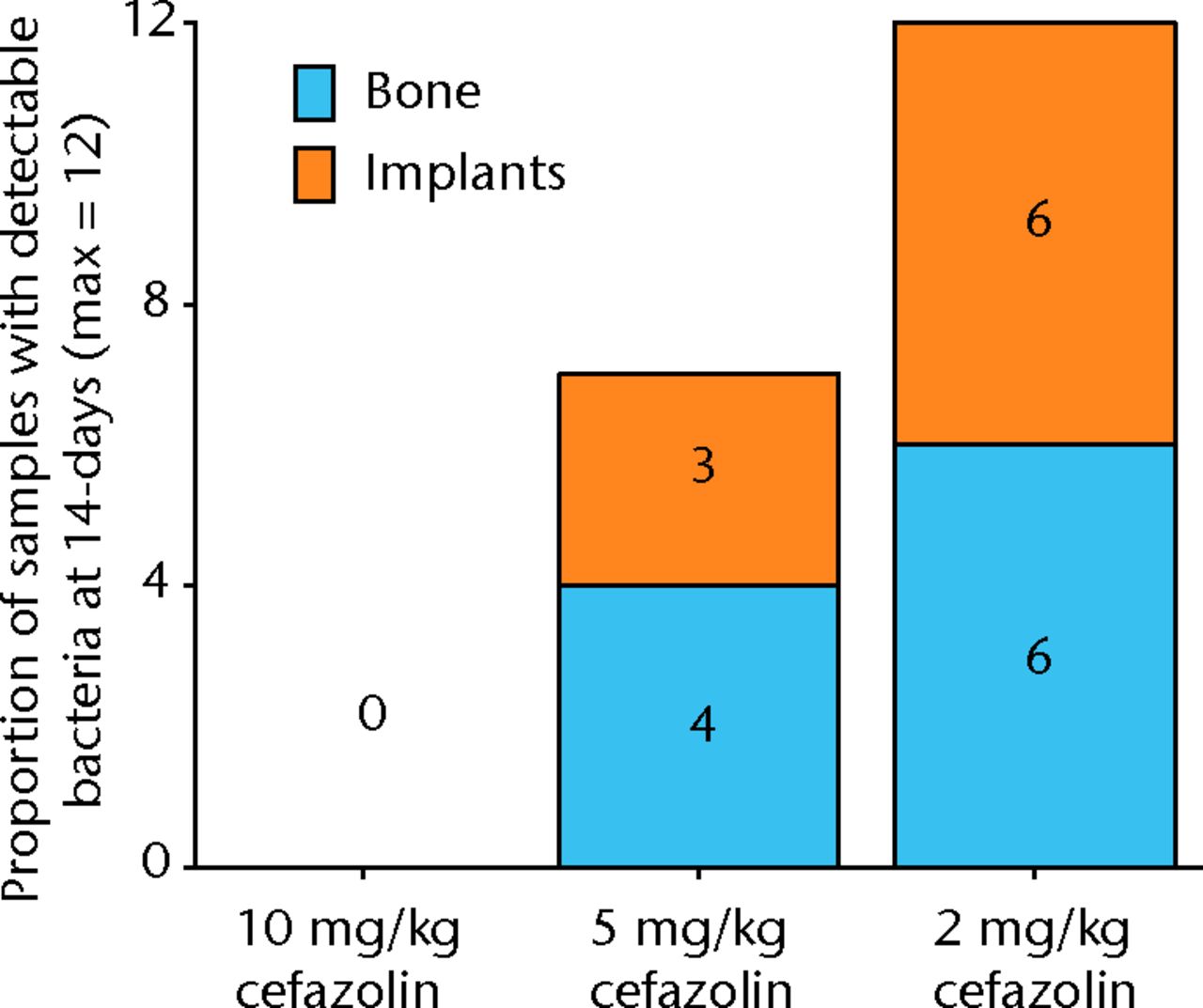
Fig. 4
Graph showing the proportion of samples with detectible bacteria in each group of six animals (maximum of 12 samples per group) 14 days after inoculation with 1 × 105 colony-forming units (CFU) of S. aureus and treatment with various doses of systemic cafazolin for 72 hours.
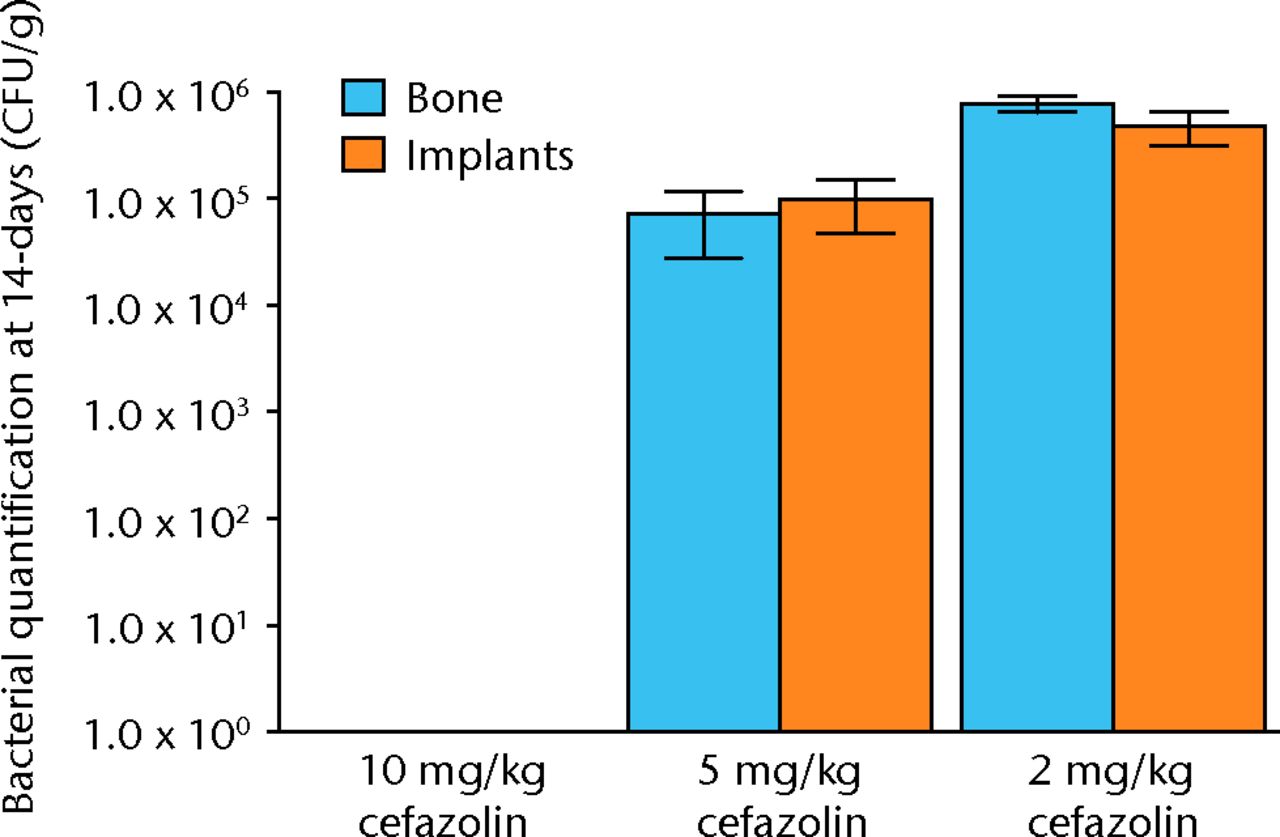
Fig. 5
Graph showing the quantification of bacteria recovered from animals 14 days after inoculation with 1 × 105 colony-forming units (CFU) of S. aureus and treatment with various doses of systemic cafazolin for 72 hours. Error bars show standard error of the mean.
Table II
Quantification of bacteria recovered from animals 14 days after inoculation with 1 × 105 colony-forming units (CFU) of S. aureus and treatment with various doses of systemic cafazolin for 72 hours. Results are given in CFU (SEM, standard error of the mean)
10 mg/Kg cefazolin | 5 mg/Kg cefazolin | 2 mg/Kg cefazolin | |
---|---|---|---|
Bone mean | 0 | 7.21 × 104 | 7.83 × 105 |
Bone SEM | 0 | 4.40 × 104 | 1.35 × 105 |
Implant mean | 0 | 9.82 × 104 | 1.65 × 105 |
Implant SEM | 0 | 5.10 × 104 | 1.61 × 105 |
Systemic and local antibiotics
The results with respect to infection rate are shown in Figure 6. A 50% infection rate was not clearly established in this study but the relationship between systemic antibiotic dosage and infection in the presence of antibiotic beads is shown in Figure 7 and Table III.
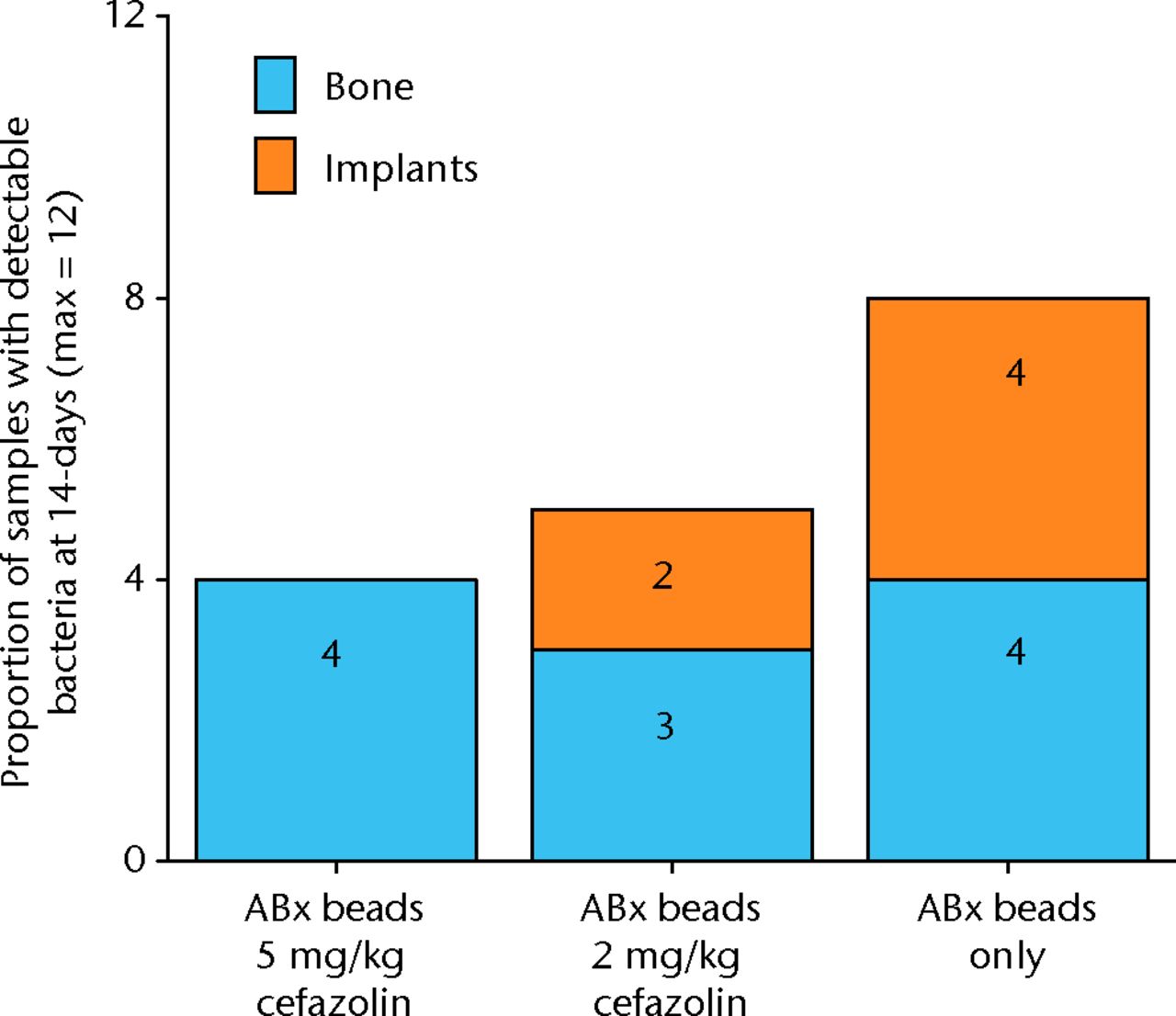
Fig. 6
Graph showing the proportion of samples with detectible bacteria in each group of six animals (maximum of 12 samples per group) 14 days after inoculation with 1 × 105 colony-forming units (CFU) of S. aureus and treatment with antibiotic beads and various doses of systemic cafazolin for 72 hours.
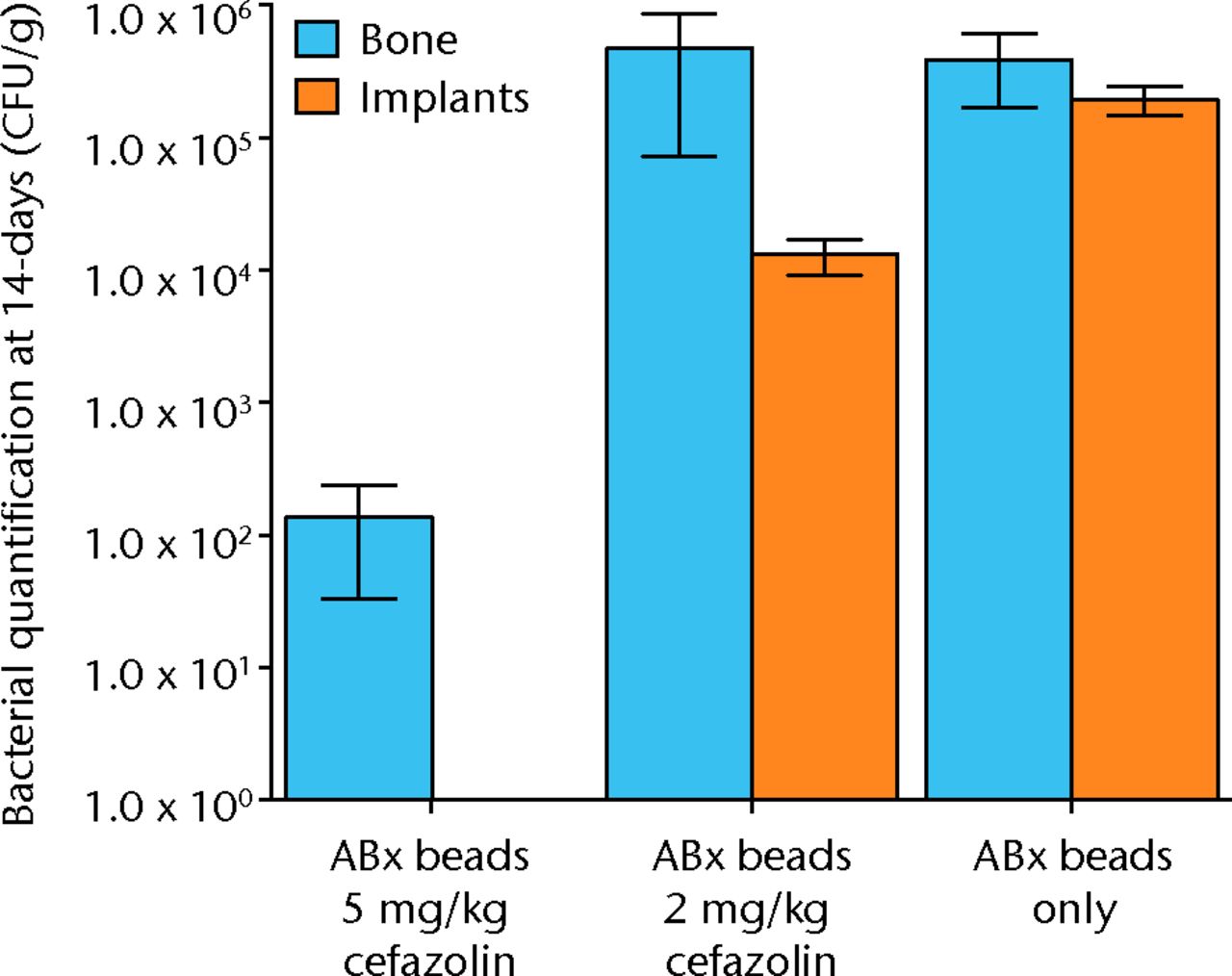
Fig. 7
Graph showing the quantification of bacteria recovered from animals 14 days after inoculation with 1 × 105 colony-forming units (CFU) of S. aureus and treatment with antibiotic beads and various doses of systemic cafazolin for 72 hours. Error bars show standard error of the mean.
Table III
Quantification of bacteria recovered from animals 14 days after inoculation with 1 × 105 colony-forming units (CFU) of S. aureus and treatment with antibiotic beads and various doses of systemic cafazolin for 72 hours. Results are given in CFU; SEM, standard error of the mean.
5 mg/Kg cefazolin antibiotic beads | 2 mg/Kg cefazolin antibiotic beads | Antibiotic beads only | ||
---|---|---|---|---|
Bone mean | 1.36 × 102 | 4.72 × 105 | 3.89 × 105 | |
Bone SEM | 1.03 × 102 | 4.00 × 105 | 2.21 × 105 | |
Implant mean | 0 | 1.32 × 104 | 1.96 × 105 | |
Implant SEM | 0 | 3.98 × 103 | 4.79 × 104 |
Discussion
Bacterial inoculation-relevance
The early experience of this model in our institution7 used an inoculant of 105 CFUs. When this was treated with irrigation and debridement at six hours, robust infection was detectable in all animals, necessitating differentiation in the degree of contamination using bacterial quantification.7 There is a danger that when the bacterial contamination is this stringent, the effect of treatments that produce anything less than a massive effect on bacterial levels will not be detected, leading to a Type II error. Additionally, a treatment that actually exerted a negative influence on infection i.e. brought about an increase in infection, might not be detected. Finally, in an under-contaminated wound where none of the wounds would become infected due to insufficient bacterial inoculation, a positive treatment effect might not be detected. These examples show the importance of avoiding a floor or ceiling effect. The results of this study establish the bacterial inoculation associated with a 50% infection rate therefore refining this model for further studies.8
Systemic relevance of antibiotics
Animals inoculated with 105 CFU of bacteria, and then surgically treated at six hours, had an infection rate of approximately 50% when treated with 5 mg/kg of cafazolin for three days. This allows for the surgical and antibiotic treatment to be initiated at different time points, allowing the effect of timing of treatment to be explored.9 Use of systemic antibiotics at a dosage that still results in 50% infection rates allows treatments that work harmoniously with antibiotics to be tested, once again establishing the ‘tipping point’ for infection and thus avoiding floor or ceiling effects in this modification of the basic model.
Systemic and local relevance of antibiotics
In clinical practice, antibiotics may be delivered locally as well as systemically.10 In fact, this may represent the area of treatment with the most potential for development. The most common local antibiotic vehicle remains antibiotic-loaded PMMA ‘bone cement’ beads.11 This institution has previously published results of the introduction of antibiotic PMMA beads into this open-fracture model.7
This study shows infection outcomes in a context closely mimicking the regular clinical practice of concurrent treatment with systemic and local antibiotics. This permits the model to be used to investigate the relationship between infection and combinations of antibiotics delivered in different forms.12
Interpretation
The sigmoid curve as shown in Figure 8 is a common treatment effect across dose response studies. In this clinical scenario, a low level of contamination is easily managed by the host with no infection; however, at a certain level of contamination, a tipping point is reached, above which clinical infection overwhelms this host immunity. Clinically, we may influence this situation with a therapy that translates to the left of the curve. If it moves the contamination off the steepest part of the curve, it may mean that even a moderate treatment effect creates a sufficiently different infection rate to reach significance (Fig. 8).
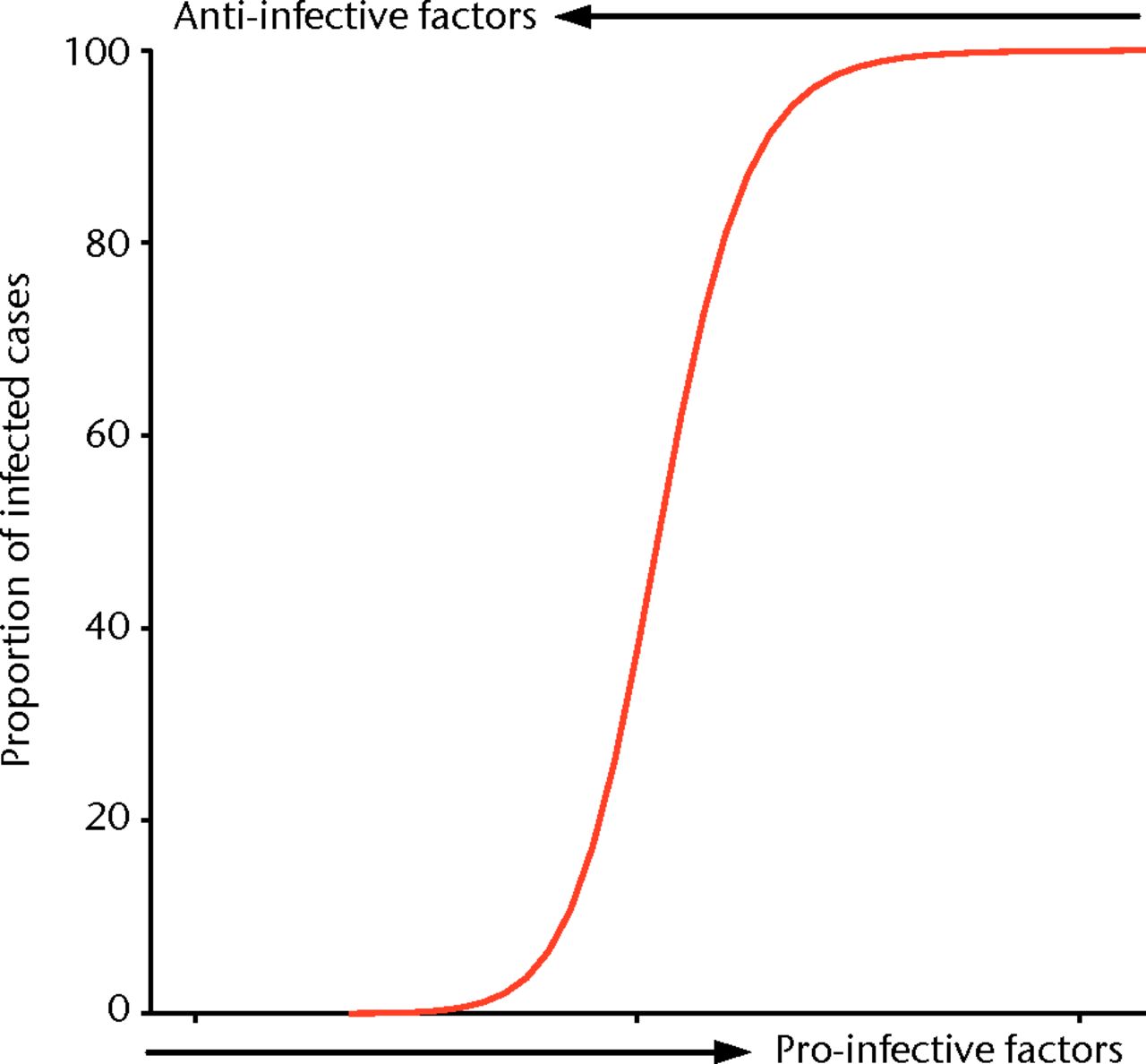
Fig. 8
Idealised curve representing the ‘tipping point’ at which a host’s immune system is unable to eradicate infection and a group of animals in a study group will move from 0% infection to 100% infection, with a small increase in pro-infective factors or a small decrease in anti-infective factors.
In the model, finding this same tipping point of initial contamination for a set control group with a 50% infection rate allows us to maximise the potential for demonstrating an effect, whether positive or negative, of an intervention group. We describe our results when the pro- and anti-infective variables are changed subtly to result in a significant effect on the proportion of animals with bacteria recoverable from their wounds. These results demonstrate this discrete ‘tipping point’ in the balance between pro- and anti-infective factors across multiple studies which test novel products and treatment strategies, and the importance of this to avoid floor or ceiling effects of tested interventions.8,13 Minimising the risk of studies that do not demonstrate efficacy, or those that do not demonstrate the lack thereof, is a waste of investigators time, resources and is unethical in studies using animals. By maximising the potential to establish strategies worth taking further, while minimising the power of these studies, we believe we have shown the ability of this model and these techniques to perform efficient basic science research.
Model flexibility
Although the studies discussed in this paper have focused on a single outcome measure of deep infection, this model can also be used to examine the effect of treatments on fracture healing. If the animals are kept alive for longer than 14 days, then bone healing in the defect can be quantified with micro-CT or histology after euthanasia.14,15
The studies presented here all involved a six-hour delay between contamination and surgical treatment. This delay has been shown to have a large effect on infection.7,13 If there is a longer delay between infection and debridement, this model can be used to investigate a chronic, recalcitrant infection.
This model could be used with a multiple photoluminescent bacteria strain to examine polymicrobial infection, which has yet to be accurately characterised in an animal model.
Study weaknesses
The model used in this study mimics clinical practice but does not re-create the clinical reality of open fractures. Some key differences include the absence of significant soft-tissue damage, a surgically created defect rather than fracture, a single surgical treatment, and immediate fixation and primary closure between injury with contamination and surgical treatment, including debridement and irrigation, which clearly differs from clinical practice. It has been argued that measuring bacterial numbers is a measure of contamination and is not necessarily analogous to infection. We used cafazolin in this model because cefalosporins are a recommended antibiotic option in open fractures,16 and previous experience with cafazolin in similar animal models reduced the model development work required to design this study. We believe that as this model involves an organism known to be sensitive to the antibiotic used; the effects observed should be similar with different combinations of bacteria and antibiotic. However, it is possible that the effects observed might be more pronounced with intravenous administration of antibiotics than with oral ingestion or the subcutaneous route that was used in this model, in order to avoid the morbidity from repeated venupuncture in a small mammal. Within the limits of animal welfare and practicality of a cost-effective model, we believe our results demonstrate the versatility, reproducibility, and functionality of this model to examine effectively multiple and clinically relevant interventions.
1 MacKenzie EJ , BosseMJ, PollakAN, et al.Long-term persistence of disability following severe lower-limb trauma. Results of a seven-year follow-up. J Bone Joint Surg [Am]2005;87-A:1801–1809.CrossrefPubMed Google Scholar
2 Penn-Barwell JG , BennettPM, FriesCA, et al.Severe open tibial fractures in combat trauma: management and preliminary outcomes. Bone Joint J2013;95-B:101–105.CrossrefPubMed Google Scholar
3 Anglen JO . Comparison of soap and antibiotic solutions for irrigation of lower-limb open fracture wounds. A prospective, randomized study. J Bone Joint Surg [Am]2005;87-A:1415–1422.CrossrefPubMed Google Scholar
4 Chen X , KidderLS, LewWD. Osteogenic protein-1 induced bone formation in an infected segmental defect in the rat femur. J Orthop Res2002;20:142–150.CrossrefPubMed Google Scholar
5 Petty W , SpanierS, ShusterJJ, SilverthorneC. The influence of skeletal implants on incidence of infection. Experiments in a canine model. J Bone Joint Surg [Am]1985;67-A:1236–1244.PubMed Google Scholar
6 Pribaz JR , BernthalNM, BilliF, et al.Mouse model of chronic post-arthroplasty infection: noninvasive in vivo bioluminescence imaging to monitor bacterial burden for long-term study. J Orthop Res2012;30:335–340.CrossrefPubMed Google Scholar
7 Brown KV , WalkerJA, CortezDS, MurrayCK, WenkeJC. Earlier debridement and antibiotic administration decrease infection. J Surg Orthop Adv2010;19:18–22.PubMed Google Scholar
8 Penn-Barwell JG , MurrayCK, WenkeJC. Comparison of the antimicrobial effect of chlorhexidine and saline for irrigating a contaminated open fracture model. J Orthop Trauma2012;26:728–732.CrossrefPubMed Google Scholar
9 Penn-Barwell JG , MurrayCK, WenkeJC. Early antibiotics and debridement independently reduce infection in an open fracture model. J Bone Joint Surg [Br]2012;94-B:107–112.CrossrefPubMed Google Scholar
10 Keating JF , BlachutPA, O'BrienPJ, MeekRN, BroekhuyseH. Reamed nailing of open tibial fractures: does the antibiotic bead pouch reduce the deep infection rate?J Orthop Trauma1996;10:298–303.CrossrefPubMed Google Scholar
11 Zalavras CG , PatzakisMJ, HoltomP. Local antibiotic therapy in the treatment of open fractures and osteomyelitis. Clin Orthop Relat Res2004;427:86–93.CrossrefPubMed Google Scholar
12 Penn-Barwell JG, Murray CK, Wenke JC. Local Antibiotic Delivery by a Bioabsorbable Gel is Superior to PMMA Bead Depot at Reducing Infection in an Open Fracture Model. J Orthop Trauma 2013.(Epub). Google Scholar
13 Penn-Barwell JG , MurrayCK, WenkeJC. Early antibiotics and debridement independently reduce infection in an open fracture model. J Bone Joint Surg [Br]2012;94-B:107–112.CrossrefPubMed Google Scholar
14 Li B , BrownKV, WenkeJC, GuelcherSA. Sustained release of vancomycin from polyurethane scaffolds inhibits infection of bone wounds in a rat femoral segmental defect model. J Control Release2010;145:221–230.CrossrefPubMed Google Scholar
15 Guelcher SA , BrownKV, LiB, et al.Dual-purpose bone grafts improve healing and reduce infection. J Orthop Trauma2011;25:477–482.CrossrefPubMed Google Scholar
16 Nanchahal J, Nayagam S, Khan U, et al. Standards for the Management of Open Fractures of the Lower Limb. London: Royal Society of Medicine Press, 2009. Google Scholar
Funding statement:
None declared
Author contributions:
J. G. Penn-Barwell: Study design, Data collection, Data interpretation, Manuscript writing
B. C. C. Rand: Study design, Data collection, Data interpretation, Manuscript writing
K. V. Brown: Data interpretation, Manuscript writing
J. C. Wenke: Study design, Data interpretation, Manuscript writing
ICMJE Conflict of Interest:
None declared
©2014 The British Editorial Society of Bone & Joint Surgery. This is an open-access article distributed under the terms of the Creative Commons Attributions licence, which permits unrestricted use, distribution, and reproduction in any medium, but not for commercial gain, provided the original author and source are credited.