Abstract
The treatment of osteochondral lesions and osteoarthritis remains an ongoing clinical challenge in orthopaedics. This review examines the current research in the fields of cartilage regeneration, osteochondral defect treatment, and biological joint resurfacing, and reports on the results of clinical and pre-clinical studies. We also report on novel treatment strategies and discuss their potential promise or pitfalls. Current focus involves the use of a scaffold providing mechanical support with the addition of chondrocytes or mesenchymal stem cells (MSCs), or the use of cell homing to differentiate the organism’s own endogenous cell sources into cartilage. This method is usually performed with scaffolds that have been coated with a chemotactic agent or with structures that support the sustained release of growth factors or other chondroinductive agents. We also discuss unique methods and designs for cell homing and scaffold production, and improvements in biological joint resurfacing. There have been a number of exciting new studies and techniques developed that aim to repair or restore osteochondral lesions and to treat larger defects or the entire articular surface. The concept of a biological total joint replacement appears to have much potential.
Cite this article: Bone Joint Res 2013;2:193–9.
Articular cartilage is an important and specialised tissue that increases joint congruence, protects the subchondral bone from high stresses and reduces friction at the edge of long bones.1,2 The function of articular cartilage as a load-bearing tissue relies upon the maintenance and integrity of its extra-cellular matrix (ECM) and its arrangement of molecular components.3 The finely tuned dynamic equilibrium of healthy articular cartilage is maintained and tightly controlled by anabolic growth factors, cytokines, matrix metalloproteinases (MMPs) and tissue inhibitors of metalloproteinases (TIMPs). Uncoupling of the balance between synthesis and degradation leads to a slow progressive loss of the macromolecular components from the ECM and the eventual degradation of the articular cartilage tissue as typified by osteoarthritis (OA).4-6 The susceptibility of articular cartilage to progression towards OA is a consequence of its avascular nature and low capacity for self-repair following injury.7 The treatment of osteoarthritis and focal cartilage defects remains a challenging topic within the field of orthopaedics.
Osteochondral lesions, which involve both the articular cartilage and the subchondral bone, typically lead to the development of fibrocartilage that has different biomechanical properties from the native hyaline cartilage and does not protect the subchondral bone from further degeneration.8 Left untreated, osteochondritis dissecans (OCD) lesions can lead to the development of OA at an early age, resulting in progressive pain and disability.9 Currently available treatments for OCD lesions include debridement, microfracture chondroplasty, autogenous osteochondral transplantation (AOT)/mosaicplasty, and autologous chondrocyte implantation (ACI).
Microfracture chondroplasty consists of the penetration of the subchondral bone with the subsequent release of progenitor cells from the bone marrow cavity into the defect.10 Morphologically the progenitor cells resemble chondrocytes,11 but produce a fibrocartilaginous cartilage generally acknowledged to be mechanically and biochemically inferior to native hyaline cartilage tissue.12 AOT involves the transfer of autologous osteochondral grafts. Cylindrical plugs of hyaline cartilage are removed with underlying subchondral bone from an unaffected area and implanted into the chondral defect.10 Similarly, mosaicplasty involves the placement of multiple smaller autologous ostechondral plugs into a chondral defect, yielding a mosaic-like pattern. Medium-term results are variable but have shown success. Ollat et al13 investigated 142 patients receiving mosaicplasty for OCD lesions (mean size 2.29 cm2). At a mean follow-up of eight years, they reported a rate of patient satisfaction of 81.8% with significant improvements in subjective scores from baseline.13 Smaller defects and a shorter delay to surgery were found to be good prognostic factors. AOT/mosaicplasty is a single-stage procedure (Fig. 1), although there is potential donor site morbidity due to the harvesting of the osteochondral plug(s).
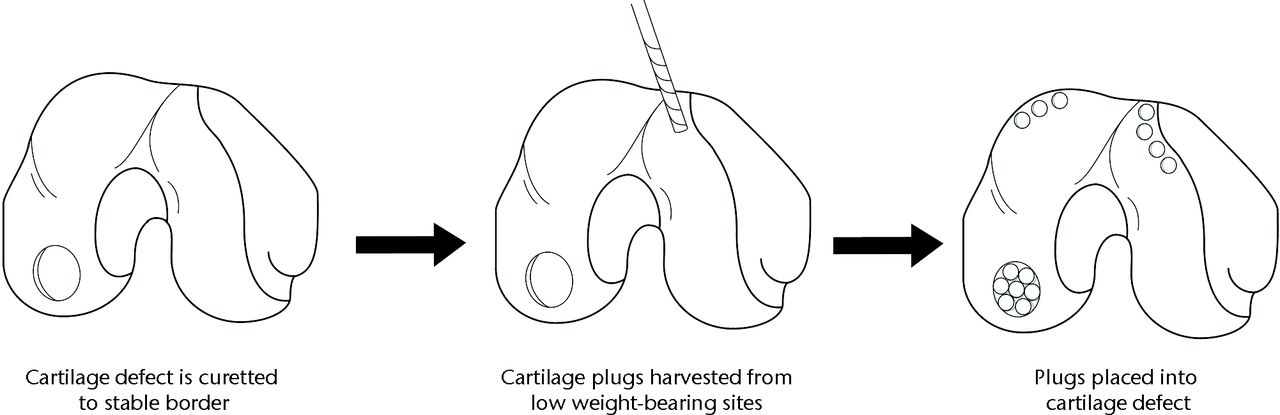
Fig. 1
Schematic of the autogenous osteochondral transplantation (AOT) procedure.
ACI comprises the isolation of chondrocytes from a small cartilage piece harvested from a low weight-bearing area of the knee joint, the expansion of the chondrocytes in vitro for two to three weeks, followed by their transplantation into the chondral defect with a covering periosteal patch (Fig. 2).10 This technique has been found to form new type II hyaline cartilage.14 A variation of the procedure involves the use of a matrix (matrix autologous chondrocyte implantation (M-ACI)). In this method, a chondrocyte-seeded biodegradable collagen matrix is implanted onto the defect site without the use of a periosteal flap.15,16 At five years after M-ACI, patients were found to have complete integration with surrounding native cartilage on MRI and had improvement in the Lysholm score17 and visual analogue scale (VAS) for pain, but no difference in Tegner18 activity levels.19 Ten-year results after ACI showed cartilage filling of > 50% of the initial defect in nine of 12 patients with moderate degenerative changes of the knee but with improved functional scores.20 ACI and M-ACI have demonstrated acceptable medium-term results but require complex manufacturing practices, are not necessarily cost-effective, and involve exposing the patient to multiple procedures for the harvest, culture, and subsequent implantation of cells.12
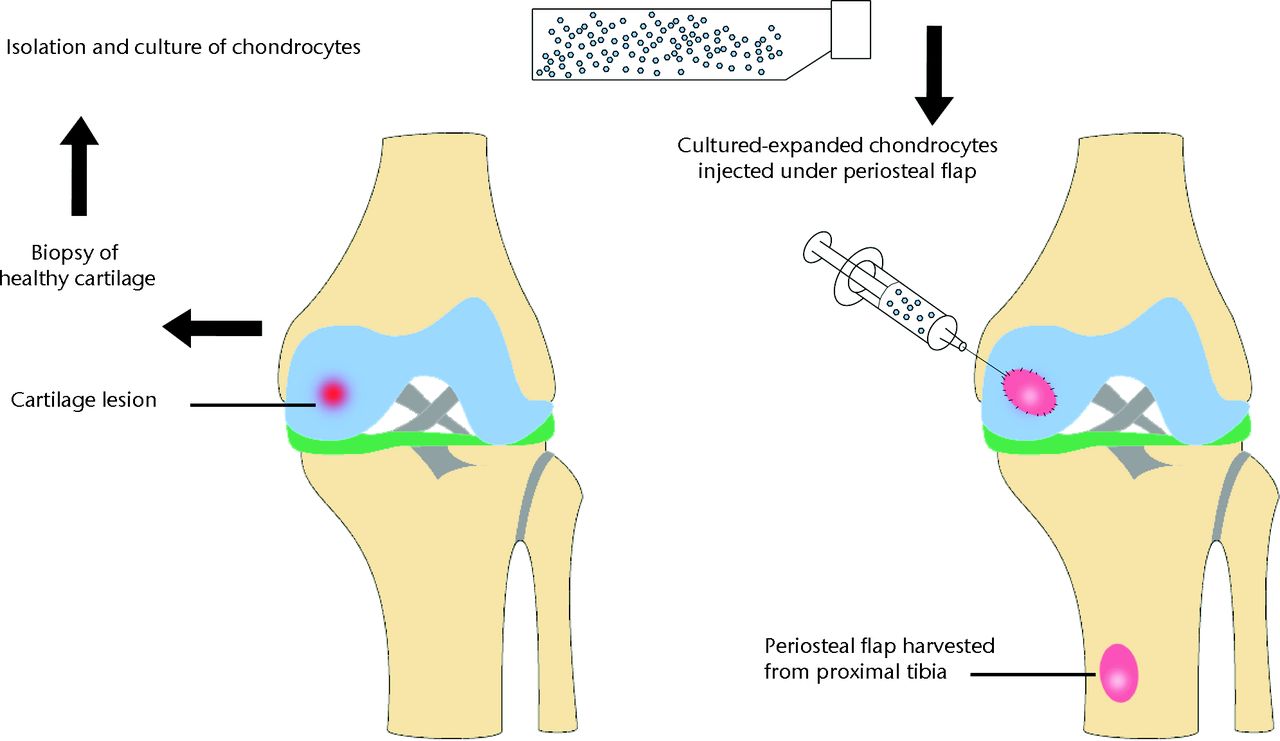
Fig. 2
Diagram of the autologous chondrocyte implantation (ACI) procedure. A sample of healthy cartilage is isolated and then expanded in vitro over two to three weeks. The chondrocytes are then implanted into the defect and covered with a periosteal patch.
Current treatments for OA treat the symptoms of the disease, and include conservative measures such as physical therapy, weight loss, non-steroidal anti-inflammatory drugs (NSAIDs), injections of corticosteroid or hyaluronic acid (HA), and total joint arthroplasty for end-stage OA. There are currently no available medical or surgical treatments that are curative or result in the repair or restoration of the damaged articular cartilage surface. Treatment strategies for degenerative articular cartilage disease and osteochondral defects remain a challenge. There have been a number of exciting new studies and techniques developed that aim to repair or restore OCD lesions and to treat larger defects or the entire articular surface. The aim of this review is to examine current research in the fields of cartilage regeneration, OCD treatment, and biological joint resurfacing, and report on the results of clinical and pre-clinical studies. We also aim to report on novel treatment strategies and their potential promise or pitfalls.
We searched MEDLINE, OVID, and PubMed (January 2010 to current) using the terms ‘cartilage’ and ‘mesenchymal stem cells’ with ‘regeneration’, ‘repair’, and ‘engineering’. We also searched using the terms ‘biologic joint resurfacing’. We focused on publications within the last three years, but did not exclude commonly referenced or highly regarded older publications. We also included publications from within the last five years if they were judged to be relevant. The search process was not limited to English-language articles. We also searched the reference lists of articles identified by our search strategy and selected those we deemed pertinent. Several review articles were included because they provided comprehensive and thorough reviews of the subject matter. The reference list was modified during the peer-review process on the basis of comments from reviewers. The general trend of current research involves the use of a scaffold or structure providing mechanical support with the addition of chondrocytes or mesenchymal stem cells (MSCs), or by using cell homing to differentiate endogenous cell sources into cartilage. This method is usually performed with scaffolds that have been coated with a chemotactic agent and relies on the organisms own endogenous cells to form new cartilage.
A phase II trial comparing M-ACI grown under bioreactor conditions with the microfracture technique showed significantly improved clinical outcomes in the M-ACI group at 26-month follow-up.21 The treatment of larger cartilage lesions has remained especially difficult. Vijayan et al22reported good to excellent clinical outcomes in 12 of 14 patients with large OCD lesions (mean size 7.2 cm2) using two M-ACI membranes with impaction grafting of the subchondral bone at a mean follow-up of five years.
An allograft adult cartilage product that was dessicated and micronised was placed into OCD lesions in rabbit and baboon models after microfracture, resulting in the formation of type II cartilage.23,24 A juvenile allograft cartilage product has been developed that is being used to fill focal OCD lesions, especially as an adjunct to microfracture.25 Recently, sphingosine- and SDF-1-seeded polymer scaffolds were placed in a chondral defect in rat knees after microfracture and were found to produce hyaline cartilage.26 The sphingosine-seeded scaffolds and the SDF-1-seeded scaffolds each resulted in the filling of the OCD lesion with type II collagen, as well as the scaffolds seeded with both sphingosine and SDF-1. However, the combination of sphingosine and SDF-1 did not show greater cartilage regeneration than either sphingosine or SDF-1 alone.26
Another area of interest involves the use of mesenchymal stem cells that can be seeded onto scaffolds, used as surgical adjuncts, or used as stand alone treatments. MSCs have a capacity for self-renewal, self-maintenance, and a potential for differentiation into cells forming multiple mesodermal tissues including chondrocytes.27 Additionally, they can migrate toward injured tissues (homing/trafficking) where they display trophic effects. Nejadnik et al28 compared bone marrow-derived MSC (BMSC) implantation with traditional ACI in 72 patients and demonstrated no difference in clinical and subjective outcomes at 24 months follow-up. The BMSC implantation was advantageous because it only required one surgical procedure, with less donor morbidity and lower costs. The bone marrow aspirate was taken using local anaesthesia, the BMSCs were then cultured for four to five weeks and implanted underneath a periosteal patch in the same method as ACI. BMSCs on a platelet fibrin glue scaffold were used to treat OCD lesions in five patients, with three showing complete defect filling and complete surface congruity with native cartilage on MRI at 12 months. The remaining two patients had incomplete congruity.29 In 2011 Kasemkijwattana et al30 reported good defect filling and incorporation with the adjacent cartilage, and good repair tissue stiffness with the use of cultured BMSCs on a collagen scaffold in two patients with knee OA, and demonstrated significant clinical improvement. In another study, six patients with knee OA were given a single injection of BMSCs and showed improvements in pain and function at up to six months.31 MRI showed an increase in cartilage thickness and a decrease in the size of subchondral bone oedema in three patients (50%).31 Recently, BMSCs were seeded into a hydrogel scaffold and used to treat chondral defects in a porcine model.32 Defect filling of 99% was found at four months with 84% hyaline-like cartilage present.32
Bone marrow concentrate (BMC) has also been used to treat cartilage defects. OCD lesions in an equine model were treated with BMC injection and microfracture and demonstrated improved histological appearance and cartilage thickness than with microfracture alone.33 Giannini et al34 used BMC on a hyaluronic acid membrane to treat osteochondral lesions of the talar dome. They demonstrated similar clinical outcomes to ACI in 81 patients with the formation of hyaline like cartilage on histology on biopsy during second-look arthroscopy at one year. In another study, BMC was combined with a collagen matrix and used to treat OCD lesions of the knee in 15 patients.35 Hyaline-like cartilage was formed on biopsy and patients had improved subjective scores at two-year follow-up. A similar technique was used by Gigante et al36 in five patients with medial femoral condyle lesions. All patients had near-normal arthroscopic appearance but evidence of hyaline like cartilage was only found in one case.
Adipose-derived stem cells (ADSCs) combined with various scaffolds have shown promising results in the treatment of OCD lesions in rabbits.37-39 The injection of concentrated ADSCs together with HA, dexamethasone, and PRP in two patients resulted in improved pain and functional status at three months with MRI showing increased cartilage thickness.40 Koh and Choi41 described the use of concentrated ADSCs isolated from the infrapatellar fat pad for the treatment of knee OA in a case–control study in 25 patients. After debridement, patients received an injection of concentrated ADSCs and PRP with another two injections of PRP weekly. The control group received only debridement and the PRP injections. No major adverse events were reported using ADSCs. Clinical results at 16 months were not significantly different between the two groups, although the study group tended to have a greater degree of improvement.
Synovial-derived mesenchymal stem cells (SMSCs) are another possible source of stem cells for cartilage restoration because they display greater chondrogenic and less osteogenic potential than MSCs derived from bone marrow or periosteum,42 but only preclinical trials are available currently. A collagen/synovial cultured MSC construct with a periosteum flap was used in rabbits to treat full-thickness knee articular cartilage defects, with improved histological scores and integration with the native cartilage evidenced. Shimomura et al43 reported on a novel scaffold-free three-dimensional tissue-engineered construct that was derived from SMSCs and extracellular matrix synthesised by the cells after culturing in vitro. The construct was implanted in pigs with chondral lesions and resulted in significant tissue restoration with repair tissue noted to be similar to normal appearing cartilage. In 2011, a unique mechanism involving the use of magnetic-labelled SMSCs that were injected into rat knees in which a magnetic implant had been placed at the bottom of the chondral lesion was used.44 Improved histological scores were seen at 12 weeks with complete regeneration of articular cartilage.44
There are currently no clinical comparative studies focusing on different MSC sources and the optimal MSC source has not yet been identified. Additionally, the potential risks of MSC use have likely not been fully elucidated. However, MSCs do represent a promising approach for cartilage regeneration and for treating articular cartilage defects.
Saw et al45 developed a novel treatment approach and performed a randomised controlled trial in 50 patients comparing articular injections of autologous peripheral blood stem cells in addition to microfracture and hyaluronic acid injections versus microfracture and hyaluronic acid injections alone. At 18 months there was improved MRI morphology and histology scores, but no difference in IKDC knee scores.
Various new strategies are being developed with the aim of scaling up the size of engineered constructs that are currently more suitable for the treatment of smaller focal defects. Producing full-size engineered articular layers has been difficult due to the competing effects of nutrient transport and consumption.46 Several promising new strategies have been introduced. Lipid shelled microbubbles or microtubes have been used as porogens in hydrogel scaffolds and have been found to provide less resistance to solute diffusion and improved mechanical properties.47-49 Applied deformational loading has been used to influence fibre orientation during engineered cartilage production in order to achieve fibre alignment similar to that of native cartilage.50 Additionally, bioreactors that apply compression or rotation during the cartilage engineering process51-53 and different perfusion devices54-56 have been demonstrated. A new polymerised gel with a favourable biologic profile has been created. This polymerised gel could be used for the purpose of targeted drug delivery or targeted and sustained release of TIMPs.57
Recently, a polyester-polyurethane scaffold was developed that has significant porosity with a void content > 95%.58 The scaffold is formed from diphenylmethane diisocyanate (MDI) and polycaprolactone (PCL) and polyglycolic acid (PGA). A polymerisation reaction between MDI and PCL/PGA is performed simultaneously with a blowing reaction that produces water and CO2, which results in a foam. The foam is then thermally reticulated to form a very porous structure. Different amounts of crosslinking resulted in the creation of scaffolds with degradation times ranging from four to 16 months. The degradation time of the scaffold can be controlled specifically by changing the amount of crosslinking during the manufacturing process. This attribute of the scaffold lends it to having many possible applications, as well as the increased porosity allowing for use in larger chondral defects. In vitro studies demonstrated the formation of cartilage when the scaffold was seeded directly with chondrocytes and when the scaffold was coated with SDF-1 and seeded with human BMSCs.
Focus has been placed on engineering hydrogels as cartilage substitutes. Hydrogels are biphasic materials that have properties similar to articular cartilage, and could support the growth and differentiation of cells into new cartilage. Hydrogels based on κ-carrageenan (κC), a thermoreversible natural-origin polymer, were combined with human ADSCs.59 The hydrogels were found to encapsulate the ADSCs and to support the growth and differentiation of cartilage in vitro. Recently, a biodegradable oligo(poly(ethylene glycol)fumarate) (OPF) composite hydrogel was used in a rabbit osteochondral defect model.60 This hydrogel was implanted with gelatin microparticles containing IGF-1, TGFβ-3, or a combination of the two. The gelatin microparticles support the sustained release of their contents, which can include a variety of growth factors or chemotactic agents. All groups that utilised hydrogels with incorporated growth factors had significant improvements in cartilage morphology and histological scoring after 12 weeks. In another study, hydrogels with TGFβ-3 releasing microspheres were seeded with progenitor cells derived from the infrapatellar fat-pad of the knee and cultured for 21 days.61 The hydrogels were made from fibrin, agarose, or gellan gum. All of the hydrogels with the incorporated TGFβ-3 were found to form significant amounts of cartilage. However, the fibrin hydrogels were found to be the least chondroinductive, and the gellan gum hydrogel had the most abundant cartilage production but the phenotype was more fibrocartilaginous.
A study of interest by Ousema et al62 reported on a uniquely prepared 3D woven poly-caprolactone scaffold seeded with BMSCs. Collagen and proteoglycan content and biomechanical properties were followed for eight weeks. The BMSC seeded scaffolds were divided into three groups. One group was placed in a solution with interleukin-1 (IL-1) medium at a concentration similar to that found in the synovial fluid of patients with OA, for a period of eight weeks. The second group was placed in a chondrogenic TGFβ-3 medium for eight weeks. The third group was placed in the chondrogenic medium for four weeks and then transferred to an IL-1 medium for the next four weeks. The scaffolds placed in the chondrogenic medium for eight weeks were found to have a Young’s modulus equal to that of native hyaline cartilage. The group placed in the IL-1 medium for eight weeks had a significantly lower Young’s modulus. The scaffolds that were initially placed in chondrogenic medium and then transferred to IL-1 medium had a significantly lower collagen and proteoglycan content than the scaffolds cultured in the chondrogenic medium for the full eight weeks, and had a slightly lower Young’s modulus but the difference was not significant. This study highlights the deleterious effects that IL-1 can have on the formation of cartilage and provides some insight as to why in vivo results in patients with inflammatory synovial environments with IL-1 may not necessarily show the same results as in vitro studies.
A novel microscale three-dimensional (3D) weaving technique was used to create a woven scaffold structure that was then consolidated with a chondrocyte-hydrogel mixture.63 The construct was shown to possess compressive, tensile, and shear properties of the same order of magnitude as native articular cartilage. The unique production technique can be used to create 3D scaffold-cartilage constructs that mimic the contouring and shape of the normal joint. These structures could be potentially created and individualised to each patient based on their own specific anatomy, and could potentially be used to replace an entire articular surface. Additionally, the structures could support load bearing immediately while also providing the biological support for cartilage regeneration. However, the 3D woven scaffold structure has only been studied in vitro currently.
Lee et al64 reported the results of the biological replacement of the entire articular surface of humeral head in a rabbit model. The native humeral head was removed and a complete humeral head hemiarthroplasty scaffold was fabricated from poly-ε-caprolactone and hydroxyapatite by photolithography. The scaffold was loaded with TGFβ-3 and implanted in the same fashion as a prosthetic hemiarthroplasty is implanted. At four months the TGFβ-3 scaffold was fully covered with hyaline cartilage, with compressive and shear properties similar to native cartilage. The regenerated cartilage was avascular and integrated with regenerated subchondral bone. The non-TGFβ-3 scaffold had isolated cartilage formation with significantly less thickness and density than the TGFβ-3 scaffold.64 This novel technique is noteworthy for a variety of different reasons. First, the entire articular surface of the humeral head was replaced with new hyaline cartilage formed from the organism’s own cell sources. This technique could be employed to treat large OCD lesions as well as for resurfacing a more widely and diffusely degenerative joint. Theoretically a biological joint replacement would not be subject to the wear of prosthetic implants or the risk of aseptic loosening. A number of questions arise: could the glenoid be replaced biologically as well? Can other joints be replaced, and how long could such a biological joint replacement last? Could this technique be used efficiently in humans in a joint that is deformed from its normal appearance, and would older patients make new cartilage on such a scaffold? The study by Lee et al,64 in which the entire surface of a joint was replaced using cell-homing and endogenous stem cell recruitment, highlights a promising technique that has substantial potential for application in the field of orthopaedics.
This systematic review discusses many new and exciting improvements in the field of cartilage regeneration and biological joint resurfacing. This work has been performed with the aim of replacing and regenerating larger chondral defects and even entire articular surfaces. The idea of a biological total joint replacement appears to have much potential. An integrated approach to cartilage regeneration will likely be a focus for the future (Fig. 3). We envision a treatment strategy for OCD lesions or focal OA that may involve microfracture with morphogenic or cellular augmentation, more practical and available osteochondral allografting, and possible implantation of a scaffold seeded with MSCs or chemotactic agents. The use of a targeted drug delivery system containing TIMPs or other molecules to induce an environment favourable to hyaline cartilage regeneration with the possible addition of a systemic agent may also play a role. The future of biological resurfacing procedures will likely involve a single stage point of service approach that is both cost-effective and minimally invasive.65
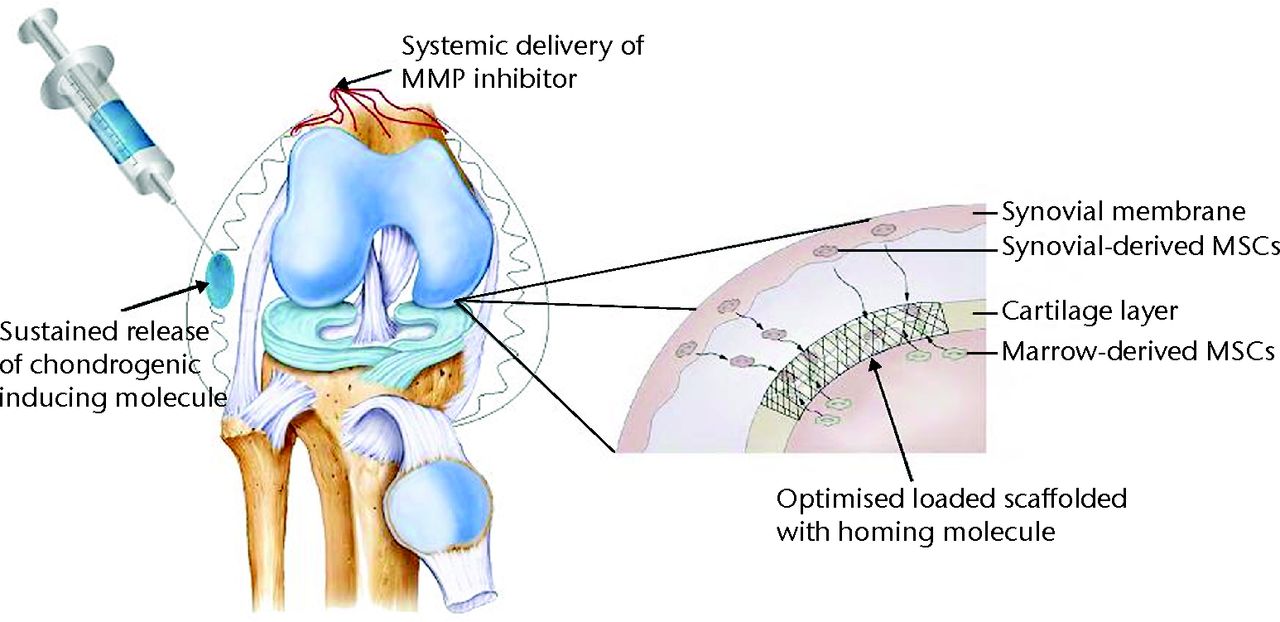
Fig. 3
Diagram showing an example of an integrated approach to cartilage regeneration combining a scaffold structure coated with a homing molecule with local and systemic chemotactic and chondrogenic agents (MMP, matrix metalloproteinase; MSC, mesenchymal stem cell).
The authors would like to thank John Schwartz for his help in drawing the figures contained in this review.
1 Mano JF , ReisRL. Osteochondral defects: present situation and tissue engineering approaches. J Tissue Eng Regen Med2007;1:261–273.CrossrefPubMed Google Scholar
2 Swieszkowski W , TuanBH, KurzydlowskiKJ, HutmacherDW. Repair and regeneration of osteochondral defects in the articular joints. Biomol Eng2007;24:489–495.CrossrefPubMed Google Scholar
3 Oldershaw RA . Cell source for the regeneration of articular cartilage: the past, the horizon and the future. Int J Exp Pathol2012;93:389–400. Google Scholar
4 Goldring MB , OteroM, PlumbDA, et al.Roles of inflammatory and anabolic cytokines in cartilage metabolism: signals and multiple effectors converge upon MMP-13 regulation in osteoarthritis. Eur Cell Mater2011;21:202–220.CrossrefPubMed Google Scholar
5 Leong DJ , HardinJA, CobelliNJ, SunHB. Mechanotransduction and cartilage integrity. Ann N Y Acad Sci2011;1240:32–37.CrossrefPubMed Google Scholar
6 Rosa SC , RufinoAT, JudasFM, et al.Role of glucose as a modulator of anabolic and catabolic gene expression in normal and osteoarthritic human chondrocytes. J Cell Biochem2011;112:2813–2824.CrossrefPubMed Google Scholar
7 Ito S , SatoM, YamatoM, et al.Repair of articular cartilage defect with layered chondrocyte sheets and cultured synovial cells. Biomaterials2012;33:5278–5286.CrossrefPubMed Google Scholar
8 Ochi M , UchioY, TobitaM, KuriwakaM. Current concepts in tissue engineering technique for repair of cartilage defect. Artif Organs2001;25:172–179.CrossrefPubMed Google Scholar
9 Detterline AJ , GoldsteinJL, RueJP, BachBR. Evaluation and treatment of osteochondritis dissecans lesions of the knee. J Knee Surg2008;21:106–115.CrossrefPubMed Google Scholar
10 Panseri S , RussoA, CunhaC, et al.Osteochondral tissue engineering approaches for articular cartilage and subchondral bone regeneration. Knee Surg Sports Traumatol Arthrosc2012;20:1182–1191.CrossrefPubMed Google Scholar
11 Mithoefer K , WilliamsRJ, WarrenRF, et al.The microfracture technique for the treatment of articular cartilage lesions in the knee: a prospective cohort study. J Bone Joint Surg [Am]2005;87-A:1911–1920. Google Scholar
12 Grande DA , SgaglioneNA. Self-directed articular resurfacing: a new paradigm?Nat Rev Rheumatol2010;6:677–678. Google Scholar
13 Ollat D , LebelB, ThaunatM, et al.Mosaic osteochondral transplantations in the knee joint, midterm results of the SFA multicenter study. Orthop Traumatol Surg Res2011;97(Suppl):S160–S166.CrossrefPubMed Google Scholar
14 Vasiliadis H , WasiakJ, SalantiG. Autologous chondrocyte implantation for the treatment of cartilage lesions of the knee: a systematic review of randomized studies. Knee Surg Sports Traumatol Arthrosc2010;18:1645–1655.CrossrefPubMed Google Scholar
15 Kon E , VerdonkP, CondelloV, et al.Matrix-assisted autologous chondrocyte transplantation for the repair of cartilage defects of the knee: systematic clinical data review and study quality analysis. Am J Sports Med2009;37(Suppl 1):156S–166S.CrossrefPubMed Google Scholar
16 Kon E , FilardoG, Di MatteoB, PerdisaF, MarcacciM. Matrix assisted autologous chondrocyte transplantation for cartilage treatment: a systematic review. Bone Joint Res2013;2:18–25.CrossrefPubMed Google Scholar
17 Lysholm J , GillquistJ. Evaluation of knee ligament surgery results with special emphasis on use of a scoring scale. Am J Sports Med 1982;3:150–154.CrossrefPubMed Google Scholar
18 Tegner Y , LysholmJ. Rating systems in the evaluation of knee ligament injuries. Clin Orthop Relat Res 1985;198:43–49.PubMed Google Scholar
19 Ventura A , MemeoA, BorgoE, et al.Repair of osteochondral lesions in the knee by chondrocyte implantation using the MACI technique. Knee Surg Sports Traumatol Arthrosc2012;20:121–126. Google Scholar
20 Pelissier A, Boyer P, Boussetta Y, et al. Satisfactory long-term MRI after autologous chondrocyte implantation at the knee. Knee Surg Sports Traumatol Arthrosc 2013:Epub. Google Scholar
21 Crawford DC , DeBerardinoTM, WilliamsRJ. NeoCart, an autologous cartilage tissue implant, compared with microfracture for treatment of distal femoral cartilage lesions: an FDA phase-II prospective, randomized clinical trial after two years. J Bone Joint Surg [Am]2012;94-A:979–989.CrossrefPubMed Google Scholar
22 Vijayan S , BartlettW, BentleyG, et al.Autologous chondrocyte implantation for osteochondral lesions in the knee using a bilayer collagen membrane and bone graft: a two- to eight-year follow-up study. J Bone Joint Surg [Br]2012;94-B:488–492.CrossrefPubMed Google Scholar
23 Chadha N, Dang A, Sampson E, et al. Porous cartilage-derived matrix scaffolds for repair of articular cartilage defects. Poster 0735. Procs ORS 2012, San Francisco, California. Google Scholar
24 Malinin T. Induction of regeneration of articular cartilage defects by freeze dried particulate cartilage allografts. Procs ICRS 2009, Miami, Florida. Google Scholar
25 Kruse DL , NgA, PadenM, et al.Arthroscopic De Novo NT juvenile allograft cartilage implantation in the talus: a case presentation. J Foot Ankle Surg2012;51:218–221. Google Scholar
26 Chinitz N, Grande D, Razzano P, et al. Use of homing molecules as an adjunct in articular cartilage repair. American Academy of Orthopaedic Surgeons 2013: Poster presentation. Google Scholar
27 Filardo G, Madry H, Jelic M, et al. Mesenchymal stem cells for the treatment of cartilage lesions: from preclinical findings to clinical application in orthopaedics. Knee Surg Sports Traumatol Arthrosc 2013:Epub. Google Scholar
28 Nejadnik H , HuiJH, Feng ChoongEP, TaiBC, LeeEH. Autologous bone marrow-derived mesenchymal stem cells versus autologous chondrocyte implantation: an observational cohort study. Am J Sports Med2010;38:1110–1116.CrossrefPubMed Google Scholar
29 Haleem AM , SingergyAA, SabryD, et al.The clinical use of human culture-expanded autologous bone marrow mesenchymal stem cells transplanted on platelet-rich fibrin glue in the treatment of articular cartilage defects: a pilot study and preliminary results. Cartilage2010;1:253–261.CrossrefPubMed Google Scholar
30 Kasemkijwattana C , HongengS, KesprayuraS, et al.Autologous bone marrow mesenchymal stem cells implantation for cartilage defects: two cases report. J Med Assoc Thai2011;94:395–400.PubMed Google Scholar
31 Emadedin M , AghdamiN, TaghiyarL, et al.Intraarticular injection of autologous mesenchymal stem cells in six patients with knee osteoarthritis. Arch Iran Med2012;15:422–428. Google Scholar
32 Lim CT , RenXF, AfizahMH, et al.Repair of osteochondral defects with rehydrated freeze-dried oligo(poly(ethylene glycol) fumarate) hydrogels seeded with bone marrow mesenchymal stem cells in a porcine model. Tissue Eng Part A2013;19:1852–1861. Google Scholar
33 Fortier LA , PotterHG, RickeyEJ, et al.Concentrated bone marrow aspirate improves full-thickness cartilage repair compared with microfracture in the equine model. J Bone Joint Surg [Am]2010;92-A:1927–1937.CrossrefPubMed Google Scholar
34 Giannini S , BudaR, CavalloM, et al.Cartilage repair evolution in post-traumatic osteochondral lesions of the talus: from open field autologous chondrocyte to bone-marrow-derived cells transplantation. Injury2010;41:1196–1203.CrossrefPubMed Google Scholar
35 Gobbi A , KarnatzikosG, ScottiC, et al.One-step cartilage repair with bone marrow aspirate concentrated cells and collagen matrix in full-thickness knee cartilage lesions: results at 2-year follow-up. Cartilage2011;2:286–299.CrossrefPubMed Google Scholar
36 Gigante A , CalcagnoS, CecconiS, et al.Use of collagen scaffold and autologous bone marrow concentrate as a one-step cartilage repair in the knee: histological results of second-look biopsies at 1 year follow-up. Int J Immunopathol Pharmacol2011;24(Suppl 2):69–72.CrossrefPubMed Google Scholar
37 McIlwraith CW , FrisbieDD, RodkeyWG, et al.Evaluation of intraarticular mesenchymal stem cells to augment healing of microfractured chondral defects. Arthroscopy2011;27:1552–1561. Google Scholar
38 Dragoo JL , CarlsonG, McCormickF, et al.Healing full-thickness cartilage defects using adipose-derived stem cells. Tissue Eng2007;13:1615–1621.CrossrefPubMed Google Scholar
39 Im GI , LeeJH. Repair of osteochondral defects with adipose stem cells and a dual growth factor-releasing scaffold in rabbits. J Biomed Mater Res B Appl Biomater2010;92:552–560.CrossrefPubMed Google Scholar
40 Pak J . Regeneration of human bones in hip osteonecrosis and human cartilage in knee osteoarthritis with autologous adipose-tissue-derived stem cells: a case series. J Med Case Rep2011;5:296.CrossrefPubMed Google Scholar
41 Koh YG , ChoiYJ. Infrapatellar fat pad-derived mesenchymal stem cell therapy for knee osteoarthritis. Knee2012;19:902–907.CrossrefPubMed Google Scholar
42 De Bari C , Dell’AccioF, KarystinouA, et al.A biomarker-based mathematical model to predict bone-forming potency of human synovial and periosteal mesenchymal stem cells. Arthritis Rheum2008;58:240–250.CrossrefPubMed Google Scholar
43 Shimomura K , AndoW, TateishiK, et al.The influence of skeletal maturity on allogenic synovial mesenchymal stem cell-based repair of cartilage in a large animal model. Biomaterials2010;31:8004–8011.CrossrefPubMed Google Scholar
44 Hori J , DeieM, KobayashiT, et al.Articular cartilage repair using an intra-articular magnet and synovium-derived cells. J Orthop Res2011;29:531–538.CrossrefPubMed Google Scholar
45 Saw KY , AnzA, Siew-Yoke JeeC, et al.Articular cartilage regeneration with autologous peripheral blood stem cells versus hyaluronic acid: a randomized controlled trial. Arthroscopy2013;29:684–694.CrossrefPubMed Google Scholar
46 O’Connell GD , LimaEG, BianL, et al.Toward engineering a biological joint replacement. J Knee Surg2012;25:187–196.CrossrefPubMed Google Scholar
47 O’Connell GD, Gollnick C, Ateshian GA, et al. Beneficial effects of chondroitinase ABC release from lipid microtubes encapsulated in chondrocytes-seeded hydrogel constructs. Procs ASME2011 Summer Bioengineering Conference, Farmington, 2011. Google Scholar
48 Wang X , WenkE, ZhangX, et al.Growth factor gradients via microsphere delivery in biopolymer scaffolds for osteochondral tissue engineering. J Control Release2009;134:81–90.CrossrefPubMed Google Scholar
49 Durney KM, Sirsi SR, Nover A, et al. Microbubbles improve depth-dependent mechanical properties of cartilage tissue engineered constructs. Orthopaedic Research Society 2011; Long Beach, CA. Google Scholar
50 Kelly TA , NgKW, WangCC, AteshianGA, HungCT. Spatial and temporal development of chondrocyte-seeded agarose constructs in free-swelling and dynamically loaded cultures. J Biomech2006;39:1489–1497.CrossrefPubMed Google Scholar
51 Mauck RL , SoltzMA, WangCC, et al.Functional tissue engineering of articular cartilage through dynamic loading of chondrocyte-seeded agarose gels. J Biomech Eng2000;122:252–260.CrossrefPubMed Google Scholar
52 Huang AH , FarrellMJ, KimM, MauckRL. Long-term dynamic loading improves the mechanical properties of chondrogenic mesenchymal stem cell-laden hydrogel. Eur Cell Mater2010;19:72–85.CrossrefPubMed Google Scholar
53 Ng KW , MauckRL, WangCC, et al.Duty cycle of deformational loading influences the growth of engineered articular cartilage. Cell Mol Bioeng2009;2:386–394.CrossrefPubMed Google Scholar
54 Raimondi MT , CandianiG, CabrasM, et al.Engineered cartilage constructs subject to very low regimens of interstitial perfusion. Biorheology2008;45:471–478.PubMed Google Scholar
55 Seidel JO , PeiM, GrayML, et al.Long-term culture of tissue engineered cartilage in a perfused chamber with mechanical stimulation. Biorheology2004;41:445–448.PubMed Google Scholar
56 Klein TJ , SahRL. Modulation of depth-dependent properties in tissue-engineered cartilage with a semi-permeable membrane and perfusion: a continuum model of matrix metabolism and transport. Biomech Model Mechanobiol2007;6:21–32.CrossrefPubMed Google Scholar
57 Wu J , ChuCC. Block copolymer of poly(ester amide) and polyesters: synthesis, characterization, and in vitro cellular response. Acta Biomater2012;8:4314–4323.CrossrefPubMed Google Scholar
58 Datta A, Lavelle L, Andrade K, et al. Novel biointegrated cross-linked degradable polyurethane scaffold matrix. Procs Society for Biomaterials, Boston, April 2013. Google Scholar
59 Popa E , ReisR, GomesM. Chondrogenic phenotype of different cells encapsulated in κ-carrageenan hydrogels for cartilage regeneration strategies. Biotechnol Appl Biochem2012;59:132–141.CrossrefPubMed Google Scholar
60 Kim K , LamJ, LuS, et al.Osteochondral tissue regeneration using a bilayered composite hydrogel with modulating dual growth factor release kinetics in a rabbit model. J Control Release2013;168:166–178.CrossrefPubMed Google Scholar
61 Ahearne M , KellyDJ. A comparison of fibrin, agarose and gellan gum hydrogels as carriers of stem cells and growth factor delivery microspheres for cartilage regeneration. Biomed Mater2013;8:035004.CrossrefPubMed Google Scholar
62 Ousema PH , MoutosFT, EstesBT, et al.The inhibition by interleukin 1 of MSC chondrogenesis and the development of biomechanical properties in biomimetic 3D woven PCL scaffolds. Biomaterials2012;33:8967–8974.CrossrefPubMed Google Scholar
63 Moutos FT , FreedLE, GuilakF. A biomimetic three-dimensional woven composite scaffold for functional tissue engineering of cartilage. Nat Mater2007;6:162–167.CrossrefPubMed Google Scholar
64 Lee CH , CookJL, MendelsonA, et al.Regeneration of the articular surface of the rabbit synovial joint by cell homing: a proof of concept study. Lancet2010;376:440–448.CrossrefPubMed Google Scholar
65 Nooeaid P , SalihV, BeierJP, BoccacciniAR. Osteochondral tissue engineering: scaffolds, stem cells and applications. J Cell Mol Med2012;16:2247–2270.CrossrefPubMed Google Scholar
Funding statement:
Funding was received from the Department of Orthopaedic Surgery, North Shore University/Long Island Jewish Medical Center.
Author contributions:
K. R. Myers: Lead author, Literature review
N. A. Sgaglione: Literature review, Editing of manuscript
D. A. Grande: Literature review, Editing of manuscript
ICMJE Conflict of Interest:
None declared
©2013 The British Editorial Society of Bone & Joint Surgery. This is an open-access article distributed under the terms of the Creative Commons Attributions licence, which permits unrestricted use, distribution, and reproduction in any medium, but not for commercial gain, provided the original author and source are credited.