Abstract
Objectives
We aimed to further evaluate the biomechanical characteristics of two locking screws versus three standard bicortical screws in synthetic models of normal and osteoporotic bone.
Methods
Synthetic tubular bone models representing normal bone density and osteoporotic bone density were used. Artificial fracture gaps of 1 cm were created in each specimen before fixation with one of two constructs: 1) two locking screws using a five-hole locking compression plate (LCP) plate; or 2) three non-locking screws with a seven-hole LCP plate across each side of the fracture gap. The stiffness, maximum displacement, mode of failure and number of cycles to failure were recorded under progressive cyclic torsional and eccentric axial loading.
Results
Locking plates in normal bone survived 10% fewer cycles to failure during cyclic axial loading, but there was no significant difference in maximum displacement or failure load. Locking plates in osteoporotic bone showed less displacement (p = 0.02), but no significant difference in number of cycles to failure or failure load during cyclic axial loading (p = 0.46 and p = 0.25, respectively). Locking plates in normal bone had lower stiffness and torque during torsion testing (both p = 0.03), but there was no significant difference in rotation (angular displacement) (p = 0.84). Locking plates in osteoporotic bone showed lower torque and rotation (p = 0.008), but there was no significant difference in stiffness during torsion testing (p = 0.69).
Conclusions
The mechanical performance of locking plate constructs, using only two screws, is comparable to three non-locking screw constructs in osteoporotic bone. Normal bone loaded with either an axial or torsional moment showed slightly better performance with the non-locking construct.
Article focus
To further elucidate biomechanical standard guidelines for diaphyseal or metaphyseal fracture fixation with non-locking screws and locking screws
To investigate the clinical dilemma faced when difficulties such as limited length for fixation in juxta-articular fractures or fractures with too short a metaphyseal fragment are encountered
To specifically determine whether two locking screws, with minimal spacing, would provide a similar mechanical performance to the more traditional configuration of three non-locking bicortical screws with minimal spacing
Key messages
The biomechanical capabilities of a two-screw locked construct is comparable to those of a three-screw non-locked construct in osteoporotic bone, thus providing adequate fixation of short metaphyseal segments in juxta-articular fractures
Strengths and limitations
The main strength is the biomechanical comparison made between non-locking and locking screws in both normal density bone substrate and osteoporotic bone substrate, mimicking important clinical scenarios
A major limitation of the study included differing plate lengths of the respective constructs and the relatively small sample size
Introduction
Locked plating techniques are increasingly being used for fracture fixation, but there is limited fundamental research in the form of biomechanical comparison studies to guide their usage.1,2 They may provide improved fixation in osteoporotic bone and superior bridging of severely comminuted fractures.3 Additionally, locking screws may be used as a fixed angle device for short metaphyseal fragments and juxta-articular shaft fractures, in which anatomic constraints limit the uses of compression plating.3 We are aware of no available data regarding the fixation strength of locking screws when used in those clinical scenarios that include limited length for fixation. The biomechanical comparison of plating strategies differing in the numbers of non-locking versus locking screws across a fracture gap has not been investigated. This concept becomes clinically significant in the setting of short metaphyseal fracture fragments, when only a shorter plate segment with two screws will fit based on anatomic or soft-tissue constraints.
The suggested benefits of locking plate technology include the improved biology of fracture healing and improved biomechanics of fracture fixation.4 Reduced disruption of the soft-tissue envelope and periosteal vascular supply are achieved by locked plating, via minimally invasive plating techniques, as well as by the ability to achieve stable constructs without relying on friction at the plate bone interface.3,5-11 From a mechanical standpoint, locking plates behave differently in comparison to conventional constructs. Without the motion at each individual screw-plate junction that occurs in non-locked constructs, locking constructs can be modeled as single beam constructs, which are significantly more stable.6,11 The type of loading seen by the bone is also significantly different for non-locked compared with locked constructs. Non-locked screws generate shear stress at the bone-screw interface upon axial loading, and the axial force is countered by a frictional force between the plate and bone. The frictional force generated leads to the overall stability of the construct.3 Locking constructs, however, subject the bone-screw interface to compressive forces, by converting shear stress into compression. Since bone is much stronger in compression, this creates another mechanical advantage for locking plates, which may be particularly useful for low-density bone where screw cut out is a problematic failure mode.4,10,12-14
Currently, there are no accepted biomechanical standard guidelines for diaphyseal or metaphyseal fixation with non-locking screws. The number of screws required for adequate fixation may depend on several factors, including the pattern of fracture, type of stability required and bone quality.1,15 The working length of a plate and screw construct is paramount to stability. This length can accurately be defined as the distance between the fracture side and the innermost screw.2 Previous studies have demonstrated that reduction of this distance will decrease strain at the fracture and thereby improve stability of the fixation construct.15 Furthermore, Törnkvist et al1 were able to demonstrate that wider spacing of screws, with maintenance of the working length, was able to procure more stability in terms of bending strength for plate and screw constructs. Likewise, there is limited data guiding the use of locking screws in terms of number and spacing.7,16,17 Theoretically, superior fixation will achieve adequate fixation strength with fewer screws. Clinically, surgeons are often faced with limited length for fixation in juxta-articular fractures or fractures with short a metaphyseal fragment. The purpose of this investigation was to determine whether two locking screws, with minimal spacing, would provide a similar mechanical performance to the more traditional configuration of three non-locking bicortical screws with minimal spacing. Furthermore, testing of this objective was carried out in bone substrates of differing porosities (mimicking normal and osteoporotic bone). The null hypothesis was that no significant differences would be observed in axial bending and torsional loading properties between the locked and non-locked constructs.
Materials and Methods
Synthetic foam bone cylinders (Pacific Research Laboratories, Vashon, Washington) were used to simulate normal and osteoporotic bone. The synthetic models were 20 mm in diameter, and consisted of a polyurethane foam-filled cylinder with a 2 mm thick epoxy shell. Standard models of two densities were used: an approximate density of 1.64 g/cm3 to simulate normal bone, and an approximate density of 0.5 g/cm3 to simulate osteoporotic bone. Density was the only difference between the two bone substituting materials. A cylinder length of 10 cm was used on both sides of the simulated fracture gap of 1 cm. Polyurethane foam was chosen to minimise the overall variability of bone substrate, as the purpose of this experiment was to compare the stability of different fixation methods.18 Previous studies have used foam models in order to eliminate the structural variability in human bone.19-21 Two constructs were used, a locking screw construct with two locking screws on either side of the fracture (5-hole LCP Combi plate; Synthes, Paoli, Pennsylvania) and a non-locking screw construct with three screws on each side (7-hole LCP Combi plate; Synthes). Both implants were manufactured from steel. In order to replicate intra-operative procedures and achieve reproducibility, locking screws were torqued to 1.5 Nm using a self-limiting torque screwdriver. Non-locking screws were hand-tightened by a single surgeon (TL), and purchase was judged according to the surgeon to gain as much friction as possible between the plate and bone. This protocol was consistent with previously published data comparing locking and non-locking fixation constructs in a sawbones model.15,22-24 The aim was to investigate a relatively shorter plate with fewer screws compared with a more traditional plate fixation with maximum screw purchase, while still maintaining a uniform working length between constructs.2,25
Two tests were undertaken to assess fatigue characteristics: torsional load and eccentric axial moment (axial + bending) load. These tests were undertaken using an MTS Bionix 858 load frame (MTS Systems, Eden Prairie, Minnesota). In order to test five samples for each individual model configuration of density (1.64 g/cm3versus 0.5 g/cm3), construct (locking versus non-locking) and specific test (torsional versus eccentric bending), a total of 40 samples were used (Fig. 1). This sample size was chosen to reflect previously reported data regarding ex vivo biomechanical comparisons of different fixation constructs.15,22-24 Photographs were taken of each sample showing the plate and the end of the screws, in order to document plate alignment and the initial 1 cm gap. Pilot destructive tests for each group were performed to determine the initial cyclical load to failure within a feasible number of cycles. Using this data, a pre-load of 100 N was selected; this load was within the elastic portion of the stress curve generated by pilot testing. A major objective of the study was to investigate the biomechanical properties of two separate constructs, in both eccentric axial bending and torsion, and the two different test formats were designed to test two different modes of failure.26,27
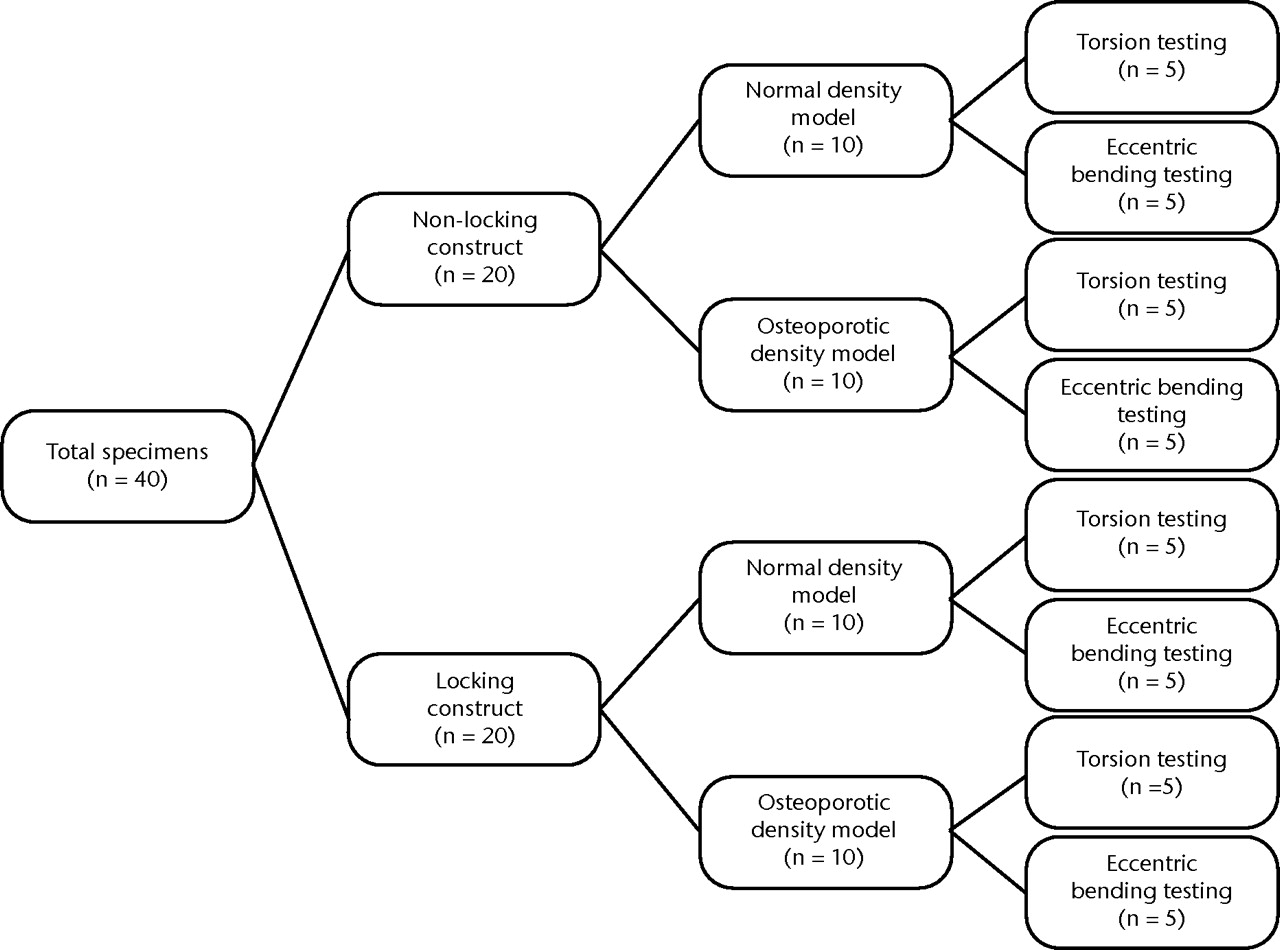
Fig. 1
Schematic illustration of the distribution of specimens for testing.
Eccentric axial loading
Eccentric test specimens were loaded in bending fixtures under load control and pre-loaded to 100 N. Progressive cyclic load testing was performed in 10 cycle blocks, at a rate of 0.1 Hz, in load-control starting at 150 N with a 10 second rest period after each 10 cycle block. The upper load was increased by 50 N after each 10 cycle block until failure, while the lower load remained 100 N throughout the test (Fig. 2). Thus, allowing appropriate qualification of which construct would display superior biomechanical properties during fatigue model testing. The tests were run until failure (failure of bone at the plate/screw interface, screw pull-out, or failure of bone outside of the plate) or until the plate bent enough for the synthetic bone cylinders to touch. Load (N), displacement (mm), number of cycles and time (s) were assessed using MTS software. The mode of failure, maximum displacement, number of cycles to failure, load at failure, and maximum load were recorded. Load versus time and displacement versus time graphs were created from the data.
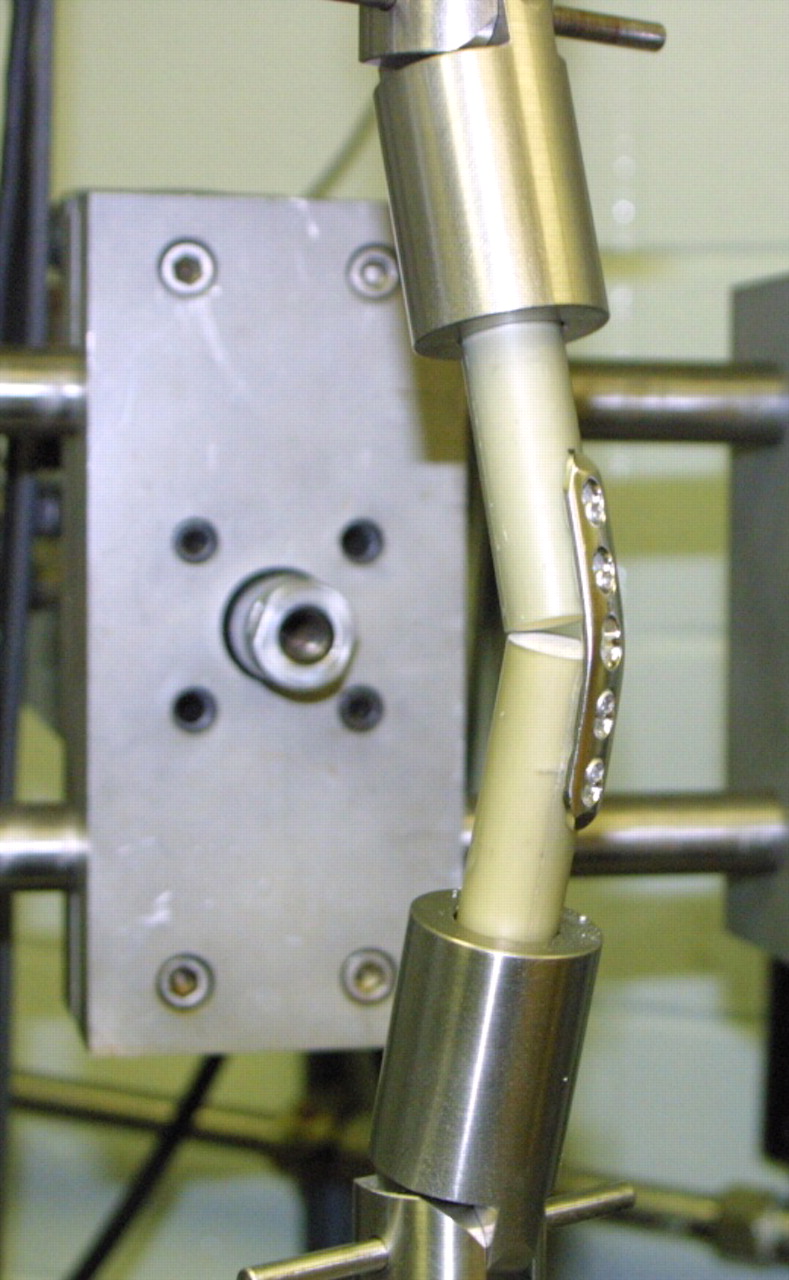
Fig. 2
Photograph showing eccentric bending of the five-hole plate fixed with locking screws. Constructs were tested cyclically until failure with the MTS Bionix frame.
Torsional testing
Torsional test samples were loaded into an alignment fixture and lowered into a testing pot, in load control, and cemented using Whip-mix Snap-Stone (Whip-mix Corporation, Louisville, Kentucky). A 3/8 inch hole was drilled through the end of each normal bone cylinder perpendicular to the plate to allow the stone to enter into the cavity and prevent slipping during testing. After curing, the bottom pot was attached to the upper fixture and lowered with load control into the other testing pot (Fig. 3). The sample was pre-loaded to 100 N and cemented. Torsional testing was performed in rotation control at 10°/min under a constant axial load of 100 N until failure or a machine limit of 100°, and tested in a static cyclic fashion. Failure was determined to be either breaking of the synthetic bone cylinders or pulling out of the screws. Torque (Nmm), rotation (°), time (s), and load (N) was collected using MTS software. Failure mode, maximum torque, maximum rotation, 2°-offset torque, 2°-offset rotation and modulus were calculated and reported. In this way, the calculated modulus is an accurate measure of the construct’s stiffness as a result of the torsional load being a uniaxial stress. Torsion versus rotation graphs were created for each sample.
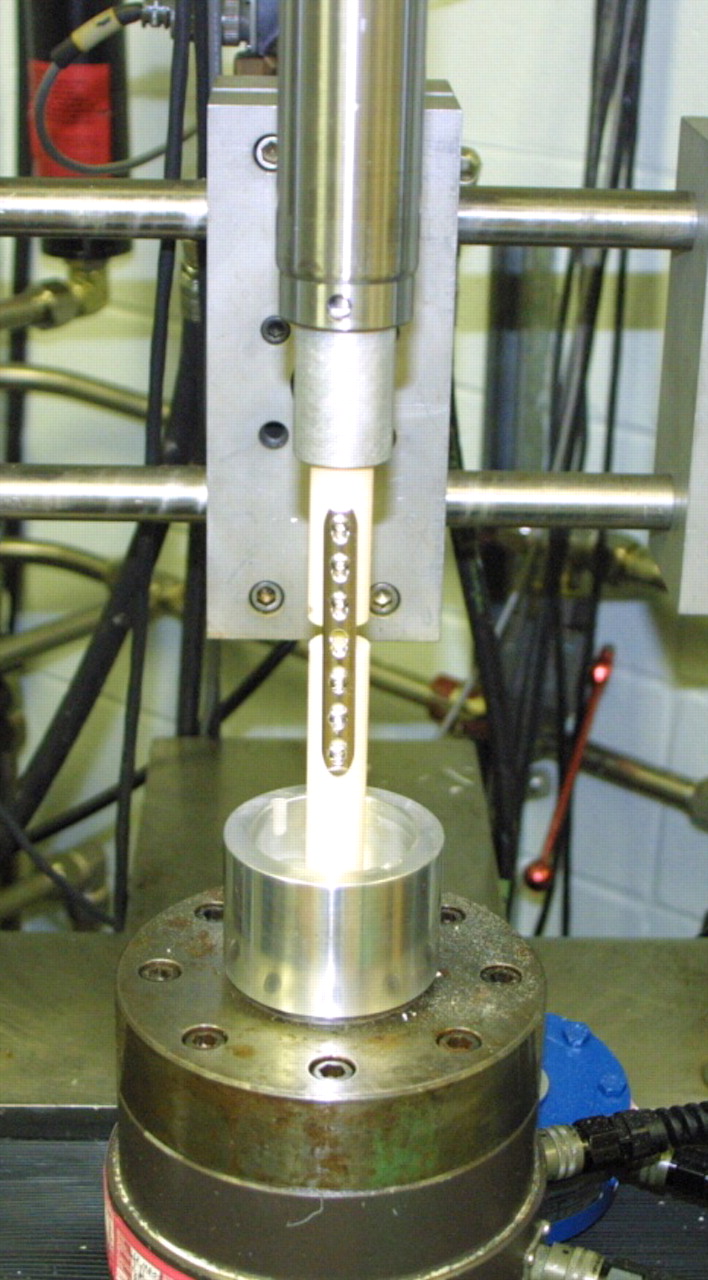
Fig. 3
Photograph showing the pre-test setup of the torsion testing model. The construct was secured with an alignment fixture and cemented into a potting apparatus.
Statistical analysis
The non-parametric Wilcoxon test was used to compare results between the non-locking and locking constructs. A two-sided p-value of 0.05 was used to determine if any significant difference was present between the two groups for any of the measured variables.
Results
Eccentric axial loading
In the normal density bone model, the locking construct withstood a median of 91 cycles (sd 7) to failure, whereas the non-locking construct failed after a median of 105 cycles (sd 3), a difference that reached statistical significance (p = 0.02). However, there were no significant differences between the locking and non-locking constructs in terms of median maximal displacement (3.99 mm (sd 1.4) versus 2.40 mm (sd 0.5), respectively; p = 0.22) or maximal load at failure (641 N (sd 22) versus 626 N (sd 40), respectively; p = 1) (Table I).
Table I
Results of eccentric bending parameters for the locking and non-locking constructs
Median measure (sd) | Locking construct | Non-locking construct | p-value* |
---|---|---|---|
Normal model (1.64 g/cm3) | |||
Maximal displacement (mm) | 3.99 (1.4) | 2.40 (0.5) | 0.22 |
Number of cycles | 91 (7) | 105 (3) | 0.02 |
Load (N) | 619 (110) | 550 (73) | 1 |
Maximal load (N) | 641 (22) | 626 (40) | 1 |
Osteoporotic model (0.5 g/cm3) | |||
Maximal displacement (mm) | 1.44 (0.2) | 1.88 (0.4) | 0.02 |
Number of cycles | 51 (7) | 52 (1.7) | 0.46 |
Load (N) | 303 (57) | 325 (9) | 0.25 |
Maximal load (N) | 306 (55) | 326 (10) | 0.69 |
-
* Wilcoxon exact test
Eccentric axial loading in the osteoporotic bone model revealed a statistically significant difference between the locking and non-locking constructs in terms of maximal displacement, with the locking plate displaying less displacement (1.44 mm (sd 0.2) versus 1.88 mm (sd 0.4); p = 0.02). There were no significant differences between the locking and non-locking constructs in terms of median cycles to failure (51 (sd 7) versus 52 (sd 1.7), respectively; p = 0.46) or maximal load (306 N (sd 55) versus 326 N (sd 10), respectively; p = 0.69) (Table I).
Torsion testing
Torsional testing of the different constructs, in the normal bone model, demonstrated the locking plate to have both a significantly lower stiffness (modulus) and ability to withstand torque (both p = 0.03). The overall stiffness of the locking plate obtained a median value of 370.9 GPa (sd 55) with an ability to withstand torque of 9044 Nmm (sd 715), in contrast to the stiffer non-locking construct values of 461.7 GPa (sd 9) and 10 632 Nmm (sd 728). Rotation, and thus angular displacement, was similar in both constructs during torsional testing in the normal bone model (Table II).
Table II
Results of torsion testing parameters for the locking and non-locking constructs
Median measure (sd) | Locking construct | Non-locking construct | p-value* |
---|---|---|---|
Normal model (1.64 g/cm3) | |||
Rotation 2° offset (°) | 22.37 (3) | 23.83 (3.4) | 0.84 |
Torque 2° offset (Nmm) | 9044 (715) | 10 632 (728) | 0.03 |
Rotation at maximum torque (°) | 45.07 (6.7) | 43.44 (12.7) | 0.84 |
Maximum torque (Nmm) | 12 115 (486) | 13 310 (1123) | 0.06 |
Modulus (GPa) | 370.9 (55) | 461.7 (39) | 0.03 |
Osteoporotic model (0.5 g/cm3) | |||
Rotation 2° offset (°) | 18.98 (3) | 14.78 (2.85) | 0.10 |
Torque 2° offset (Nmm) | 2223 (345) | 1688 (212) | 0.10 |
Rotation at maximum torque (°) | 49.98 (5.7) | 58.24 (6) | 0.008 |
Maximum torque (Nmm) | 3463 (1800) | 4507 (327) | 0.15 |
Modulus (GPa) | 130.7 (4.3) | 143.2 (16) | 0.69 |
-
* Wilcoxon exact test
In the osteoporotic bone model, there were differences in the respective constructs ability to withstand torque, demonstrated in rotation at maximal torque (p = 0.008). Specifically, the locking construct displayed a significantly superior ability to withstand torque. However, during torsion testing in the osteoporotic bone model both the locking plate construct and the non-locking plate construct demonstrated statistically similar stiffness (130.7 GPa (sd 4.3) versus 143.2 GPa (sd 16), respectively; p = 0.69) (Table II).
Mode of failure
The mode of failure of each specimen tested was photographed, recorded and categorised. The four categories included: 1) plate bending, 2) bone failure at the screw, 3) screw pull-out, and 4) failure of bone outside of the plate (edges of the fracture gap in contact). During axial bending both constructs failed an equal number of times, when either the plate deformed or the bone failed at the screw. Failure during torsional testing was also symmetric. Both constructs failed an equal number of times when the bone failed outside of the plate, and failure as a result of screw pull-out was comparable for both constructs (Table III).
Table III
Modes of failure for the 40 samples by test, construct and density (L, locking construct; NL, non-locking construct)
Eccentric bending test | Torsion test | ||||||||||
---|---|---|---|---|---|---|---|---|---|---|---|
Normal | Osteoporotic | Normal | Osteoporotic | ||||||||
Mode of failure | L | NL | L | NL | L | NL | L | NL | |||
Plate bent | 5 | 5 | |||||||||
Bone failed at screw | 5 | 5 | |||||||||
Screw pull-out | 2 | 3 | |||||||||
Bone failed outside of plate | 3 | 2 | 5 | 5 |
Discussion
The data presented in this study indicates that the initial null hypothesis may be rejected, as there were definable and significant biomechanical differences between the locking and non-locking constructs. More specifically, the biomechanical performance of two locking screws across each side of a fracture gap in the normal bone model is not comparable to the performance of three non-locking screws. Key differences in performance were noted between constructs in both cyclic eccentric axial bending and torsional testing. In the normal density bone model, the non-locking construct displayed biomechanically superior results when compared with the locking construct, surviving more cycles until failure (eccentric loading), displaying a stiffer modulus and demonstrating a superior ability to withstand torque. However, in the osteoporotic bone model, the locking construct displayed superior biomechanical results. In this model, after eccentric axial bending, the locking construct exhibited less displacement of the fracture gap and demonstrated a superior ability to endure torque during torsional testing.
However, the respective constructs did not reveal completely disparate properties. In fact, in the normal bone model the differing constructs displayed similar endurance of maximal loads and displacement of the fracture gap during eccentric axial bending. Similarly, in the osteoporotic bone model there were no significant differences between the constructs regarding cycles to failure, maximal load or overall stiffness. Therefore, the two constructs were relatively comparable in the osteoporotic bone model. Moreover, the constructs demonstrated very similar modes of failure throughout the testing process.
Previously reported data, directly comparing non-locking constructs with locking constructs, has shown mixed results. Less invasive stabilisation system (LISS) plate constructs were among the first to be tested for biomechanical properties. Gösling et al24 compared proximal tibial LISS plates with conventional double plating using cadaveric tibiae axially loaded to 400 N, 800 N, 1200 N, and 1600 N for five cycles at each load. Plastic vertical subsidence demonstrated no significant difference between the two constructs.24 In a similar manner, Peindl et al22 axially loaded synthetic tibiae and found that conventional double plating constructs were much stiffer than a lateral proximal tibial locking plate, a peri-articular plate or an external fixator construct, but there was no significant difference between the latter three.
Other studies have focused on the biomechanical properties of locked plating constructs with regard to screw configuration. These studies have also investigated biomechanical differences between locking plate constructs and non-locking plate constructs, but have mainly centered on fixation of diaphyseal fractures. Ehmke28 used foam models with a 1 cm fracture gap fixed with a 10-hole LCP using three non-locked, three locked unicortical, or three locked bicortical screws tested in torsion, axial compression, and four-point bending under progressive dynamic loading. Failure was primarily due to fracture through the foam. However, the clinical importance of this study may be limited by the loading design, which did not replicate the observed clinical failure mode of pullout due to fatigue.28 A cadaver radius model was used by Gardner et al29 to study locking versus non-locking behavior in AP bending, mediolateral bending and torsion. Their results showed significantly more torsion cycles to failure in the locking group and less energy absorbed in the AP bending, but all of the other tested outcomes showed no difference.29 Fulkerson et al18 reported results of testing foam models in cyclic axial loading followed by cyclic cantilever loading, and showed significantly more cycles to failure in bicortical locked constructs compared with unicortical locked or bicortical unlocked constructs.
Still, biomechanical studies investigating the basic properties of locking plates are very limited in number. Stoffel et al17 tested locking plate constructs using foam cylinders and large fragment locking plates. The effect of bridging length and number of screws placed in the construct were tested by measuring axial stiffness and torsional rigidity, as well as by testing to fatigue failure. Since axial stiffness was mainly influenced by plate length and torsional rigidity was mainly influenced by number of screws, the authors recommend that fractures of the lower extremity, where axial forces predominate, can be treated with two or three screws on each side of the fracture.17 Upper extremity fractures, where rotational forces predominate, should be treated with three to four screws on each side of the fracture.17
There are several limitations of the current study. One limitation common to all biomechanical studies is the inability to incorporate the effect of fracture biology in vivo. The effects of fatigue are likely to be dampened by the ongoing healing process. However, a synthetic model was chosen to limit the variability encountered within human cadaver models. Furthermore, the purpose of this study was to directly investigate the mechanical stability of differing constructs, and differences in bone quality would have raised a substantial confounding variable.18,30,31 Secondly, this study is also limited by a small sample size. Study design and sample size allocation was modelled after previously published biomechanical data comparing locking and non-locking constructs. These studies had used samples of a similar size.13,15,22,24,29 In addition, a post-hoc statistical analysis of our data was performed using the non-parametric Wilcoxon test. We were able then to assess for significance by using a cut-off p-value of less than 0.05 to determine if our measured variables differed in a statistically significant fashion. Final limitations of the study are design of the constructs and construct testing. Non-locking screws were tightened by hand at the discretion of the surgeon without the aid of a torque screwdriver; uniformity was maintained through a single surgeon.15,22,23 Plate lengths of the two constructs also differed. The lengths were chosen to mimic clinical scenarios in which the respective number of minimally spaced screws would be used in conjunction with either a 7- or 5-hole plate. Although the lengths of the plates were not uniform, a uniform working length was maintained, and an appropriate comparison between constructs can still be made.25 Biomechanical properties were tested in a somewhat dissimilar fashion, progressive axial bending and static torsion. However, two different testing format designs were required to separately investigate the two different modes of failure in the respective constructs. Future studies are needed to control for these limitations, and should include the testing of hybrid plate designs and newer plate designs intended to accommodate for short metaphyseal fracture fragments.
In summary, this study may suggest that the biomechanical capabilities of a two-screw locked construct is comparable to a three-screw non-locked construct in osteoporotic bone, thus providing adequate fixation of short metaphyseal segments in juxta-articular fractures. These results may suggest that when surgeons are faced with the clinical dilemma of anatomic restriction preventing standard fixation, the biomechanical properties of two locking screws alone are equivalent to the performance of three non-locking screws in osteoporotic bone. Normal bone loaded in both torsion and axial bending, however, showed slightly better performance with the non-locking construct. These results are not intended to be used in isolation but rather as part of the growing body of research into locking plate constructs. Accepted indications for the use of locking plate fixation include: 1) fractures of osteoporotic bone; 2) highly comminuted fractures, especially those involving diaphyseal and metaphyseal bone; and 3) metaphyseal and intra-articular fractures such as proximal humerus and distal radius fractures.10,32
Based on this study, osteoporotic fractures that are restrained by limited space available for fixation can be adequately fixated with two locking screws opposite each side of the fracture gap. However, in bone of normal physiologic density, three non-locked bicortical screws, on either side of the fracture gap, should remain the standard of care.
1 Törnkvist H , HearnTC, SchatzkerJ. The strength of plate fixation in relation to the number and spacing of bone screws. J Orthop Trauma1996;10:204–208.CrossrefPubMed Google Scholar
2 Hak DJ , AlthausenP, HazelwoodSJ. Locked plate fixation of osteoporotic humeral shaft fractures: are two locking screws per segment enough?J Orthop Trauma2010;25:207–211.CrossrefPubMed Google Scholar
3 Egol KA , KubiakKN, FulkersonE, KummerFJ, KovalKJ. Biomechanics of locked plates and screws. J Orthop Trauma2004;18:488–493.CrossrefPubMed Google Scholar
4 Haidukewych GJ . Innovations in locking plate technology. J Am Acad Orthop Surg2004;12:205–212.CrossrefPubMed Google Scholar
5 Farouk O , KrettekC, MiclauT, et al.Minimally invasive plate osteosynthesis: does percutaneous plating disrupt femoral blood supply less than the traditional technique?J Orthop Trauma1999;13:401–406.CrossrefPubMed Google Scholar
6 Tepic S , PerrenS. The biomechanics of the PC-Fix internal fixator. Injury1995;26(Suppl 2):5–10. Google Scholar
7 Hertel R , EijerH, MeisserA, HaukeC, PerrenSM. Biomechanical and biological considerations relating to the clinical use of the point contact fixator: evaluation of the device handling test in the treatment of diaphyseal fractures of the radius and/or ulna. Injury2001;32(Suppl 2):B10–B14. Google Scholar
8 Hofer HP , WildburgerR, SzyszkowitzR. Observations concerning different patterns of bone healing using the Point Contact Fixator (PC-Fix) as a new technique for fracture fixation. Injury2001;32(Suppl 2):B15–B25.CrossrefPubMed Google Scholar
9 Frigg R . Locking Compression Plate (LCP): an osteosynthesis plate based on the Dynamic Compression Plate and the Point Contact Fixator (PC-Fix). Injury2001;32(Suppl):63–66. Google Scholar
10 Perren SM . Evolution and rationale of locked internal fixator technology: introductory remarks. Injury2001;32(Suppl 2):B3–B9. Google Scholar
11 Wagner M . General principles for the clinical use of the LCP. Injury2003;34(Suppl 2):B31–B42.CrossrefPubMed Google Scholar
12 Cordey J , BorgeaudM, PerrenSM. Force transmission between the plate and the bone: relative importance of the bending stiffness of the screws friction between plate and bone. Injury2000;31(Suppl 3):C21–C28. Google Scholar
13 Zlowodzki M , WilliamsonS, ColePA, ZardiackasLD, KregorPJ. Biomechanical evaluation of the less invasive stabilization system, angled blade plate, and retrograde intramedullary nail for the internal fixation of distal femur fractures. J Orthop Trauma2004;18:494–502.CrossrefPubMed Google Scholar
14 Kubiak EN , FulkersonE, StraussE, EgolKA. The evolution of locked plates. J Bone Joint Surg [Am]2006;88-A(Suppl 4):189–200.CrossrefPubMed Google Scholar
15 Ellis T , BourgeaultCA, KyleRF. Screw position affects dynamic compression plate strain in an in vitro fracture model. J Orthop Trauma2001;15:333–337.CrossrefPubMed Google Scholar
16 Gautier E , SommerC. Guidelines for the clinical application of the LCP. Injury2003;34(Suppl 2):B63–B76.CrossrefPubMed Google Scholar
17 Stoffel K , DieterU, StachowiakG, GächterA, KusterMS. Biomechanical testing of the LCP: how can stability in locked internal fixators be controlled?Injury2003;34(Suppl 2):B11–B19. Google Scholar
18 Fulkerson E , EgolKA, KubiakEN, et al.Fixation of diaphyseal fractures with a segmental defect: a biomechanical comparison of locked and conventional plating techniques. J Trauma2006;60:830–835.CrossrefPubMed Google Scholar
19 Ansell RH , ScalesJT. A study of some factors which affect the strength of screws and their insertion and holding power in the bone. J Biomech1968;1:279–302. Google Scholar
20 Kennedy JG , CarterDR. Long bone torsion: I. Effects of heterogeneity, anisotropy and geometric irregularity. J Biomech Eng1985;107:183–188.CrossrefPubMed Google Scholar
21 Schatzker J , HorneJG, Sumner-SmithG. The effect of movement on the hodling power of screws in bone. Clin Orthop Relat Res1975;111:257–272. Google Scholar
22 Peindl RD , ZuraRD, VincentA, et al.Unstable proximal extraarticular tibia fractures: a biomechanical evaluation of four methods of fixation. J Orthop Trauma2004;18:540–545.CrossrefPubMed Google Scholar
23 Siffri PC , PeindlRD, ColeyER, et al.Biomechanical analysis of blade plateversus locking plate fixation for a proximal humerus fracture: comparison using cadaveric and synthetic humeri. J Orthop Trauma2006;20:547–554. Google Scholar
24 Gösling T , SchandelmaierP, MartiA, et al.Less invasive stabilization of complex tibial plateau fractures: a biomechanical evaluation of a unilateral locked screw plate and double plating. J Orthop Trauma2004;18:546–551.CrossrefPubMed Google Scholar
25 Sanders R , HaidukewychGJ, MilneT, DennisJ, LattaLL. Minimalversus maximal plate fixation techniques of the ulna: the biomechanical effect of number of screws and plate length. J Orthop Trauma2002;16:166–171. Google Scholar
26 Zlowodzki M , WilliamsonS, ColeP, ZardiackasLD, KregorPJ. Biomechanical evaluation of the less invasive stabilization system, angled blade plate, and retrograde intramedullary nail for the internal fixation of distal femur fractures. J Orthop Trauma2004;18:494–502.CrossrefPubMed Google Scholar
27 Zlowodzki M , WilliamsonS, ZardiackasLD, KregorPJ. Biomechanical evaluation of the less invasive stabilization system and the 95-degree angled blade plate for the internal fixation of distal femur fractures in human cadaveric bones with high bone mineral density. J Trauma2006;60:836–840.CrossrefPubMed Google Scholar
28 Ehmke LW. Stability of plate fixation constructs with locked and non-locked screws. Procs ASB 29th Annual Meeting, 2005:745. Google Scholar
29 Gardner MJ , BrophyRH, CampbellD, et al.The mechanical behavior of locking compression plates compared with dynamic compression plates in a cadaver radius model. J Orthop Trauma2005;19:597–603.CrossrefPubMed Google Scholar
30 DeCoster TA , HeetderksDB, DowneyDJ, FerriesJ, JonesW. Optimizing bone screw pullout force. J Orthop Trauma1990;4:169–174.CrossrefPubMed Google Scholar
31 Asnis SE , ErnbergJJ, BostromMP, et al.Cancellous bone screw design and holding power. J Orthop Trauma1996;10:462–469. Google Scholar
32 Cantu RV , KovalKJ. The use of locking plates in fracture care. J Am Acad Orthop Surg2006;14:183–190.CrossrefPubMed Google Scholar
Funding statement:
None declared
Author contributions:
B. Grawe: Study design, Writing the paper
T. Le: Study design, Writing the paper
A. Archdeacon: Study design, Writing the paper
S. Williamson: Data collection, Data analysis
L. Zardiackas: Data collection, Data analysis
ICMJE Conflict of Interest:
None declared
©2012 British Editorial Society of Bone and Joint Surgery. This is an open-access article distributed under the terms of the Creative Commons Attributions licence, which permits unrestricted use, distribution, and reproduction in any medium, but not for commercial gain, provided the original author and source are credited.