Abstract
In this in vitro study of the hip joint we examined which soft tissues act as primary and secondary passive rotational restraints when the hip joint is functionally loaded. A total of nine cadaveric left hips were mounted in a testing rig that allowed the application of forces, torques and rotations in all six degrees of freedom. The hip was rotated throughout a complete range of movement (ROM) and the contributions of the iliofemoral (medial and lateral arms), pubofemoral and ischiofemoral ligaments and the ligamentum teres to rotational restraint was determined by resecting a ligament and measuring the reduced torque required to achieve the same angular position as before resection. The contribution from the acetabular labrum was also measured. Each of the capsular ligaments acted as the primary hip rotation restraint somewhere within the complete ROM, and the ligamentum teres acted as a secondary restraint in high flexion, adduction and external rotation. The iliofemoral lateral arm and the ischiofemoral ligaments were primary restraints in two-thirds of the positions tested. Appreciation of the importance of these structures in preventing excessive hip rotation and subsequent impingement/instability may be relevant for surgeons undertaking both hip joint preserving surgery and hip arthroplasty.
Cite this article: Bone Joint J 2015; 97-B:484–91.
There is increasing interest in early interventional hip surgery to treat conditions from instability to femoroacetabular impingement (FAI) in young adults.1-3 However, concerns exist regarding post-operative instability following resection of the hip joint ligaments during open4 or arthroscopic surgery.5 Case reports describe instances of rapid joint degeneration following early interventional hip surgery, to a point where total hip arthroplasty (THA) becomes necessary secondary to joint subluxation6,7 as well as complete hip joint dislocation.8-11 This raises questions about the role of soft tissues and ligaments in the maintenance of hip stability.
Instability is not an infrequent complication of THA. Excessive hip rotation can lead to impingement. This may destabilise the hip by forcing the femoral head to lever out of the acetabular component, causing edge loading, high wear,12,13 or even dislocation.14 Repairing the capsule and neighbouring muscles can help reduce the post-operative dislocation rate in THA.15 However, the importance of the capsule in restraining excessive rotation and preventing impingement in the native hip is not understood. It is unclear whether these capsular repair strategies could be optimised to further improve outcomes in THA and early interventional hip surgery.
Cutting capsular ligaments results in large increases in hip joint rotational laxity.16-18 However, few research studies include the hip joint reaction force, which is common to all functional tasks.19 The hip joint reaction force is essential in gaining stability from the osseous anatomy as it forces the femoral head into the acetabulum. There is some evidence that the ligamentum teres could restrain hip rotation and help stabilise the hip.20,21 Its relative importance compared with the capsular ligaments is unknown. For informed decisions to be made about which soft tissues should be preserved or repaired during hip surgery, a complete picture of the relative contributions of each structure to hip stability needs to be defined. The preservation or repair of the capsule and ligaments varies between open and arthroscopic approaches, so an appreciation of the relative importance of each structure will inform the debate about surgical approaches and repairs.
We undertook this study to quantify the relative contributions of each ligament to restraining hip rotation throughout a complete range of movement (ROM) and under joint compression. Our null hypothesis was that the capsular ligaments, acetabular labrum and ligamentum teres contribute equally to hip joint rotational restraint.
Materials and Methods
Following approval from the local research ethics committee, nine fresh–frozen cadaveric pelves (six male), mean age 76.4 years (61 to 89) with full-length femora were defrosted and stripped of their soft tissues, while carefully preserving the hip joint capsule. Specimens were bisected into hemi-pelves and the left hip was mounted into a six degrees of freedom testing rig (Fig. 1) according to the International Society of Biomechanics (ISB) coordinate system.22 Movements in this coordinate system mimic movements of the lower limb in a clinical environment (Fig. 2), with neutral flexion, rotation and ab/adduction equating to a standing upright position (when the ISB pelvic and femoral axes align). Flexion and abduction torques were applied by hanging weights, and angular positions applied using screw clamps. Internal/external rotation torques/angles were controlled by the rotating axis of a dual-axis Instron servohydraulic materials testing machine (Instron Ltd, High Wycombe, United Kingdom) equipped with a two degrees of freedom (tension/torsion) load cell. Throughout testing, a fixed joint compression force of 110 N angled 20° medially/proximally relative to the mechanical axis of the femur was applied using the servohydraulic machine’s vertical axis and a horizontal hanging weight. This load is similar to the direction of the peak hip joint contact force measured for a variety of functional tasks.19 Translations were not applied, but were free to occur in response to the applied load by mounting the test fixture on to a freely moving table mounted on rollers to allow unrestricted translation in the horizontal plane. For each specimen all tests and measurements were performed on the same day, without removing the specimen from the testing rig. The tests were done at room temperature and the specimens were kept moist by sparaying regularly with water.
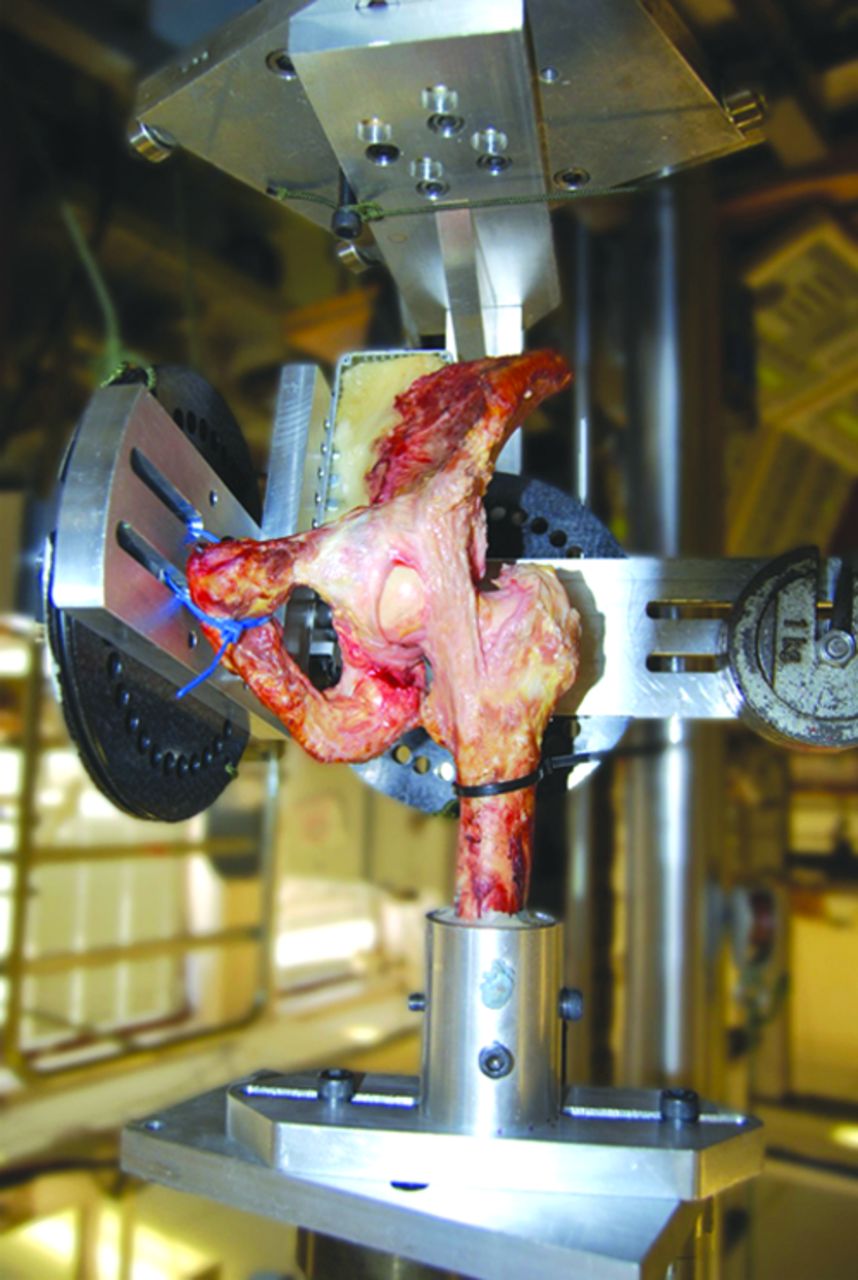
Fig. 1
Photograph showing the anterior view of a left hemi-pelvis mounted in the custom-built testing rig. The hip is in neutral ad/abduction, extension and external rotation and all capsular ligaments have been resected except for the medial iliofemoral ligament, which can be seen to be taut.
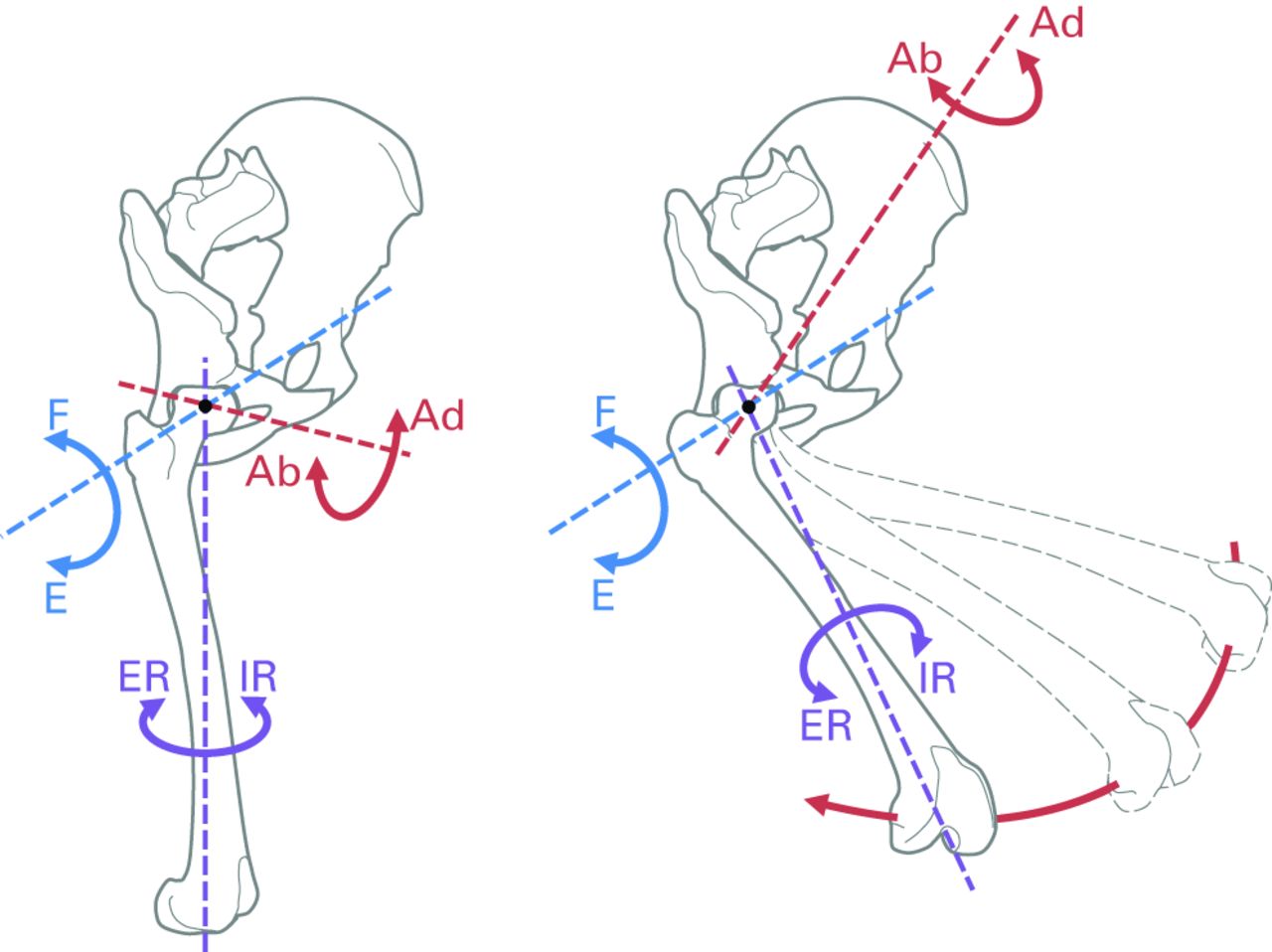
Fig. 2
Diagram showing the co-ordinate system used throughout testing. The axis for flexion/extension (F/E, blue) is fixed to the pelvis. The axis for internal/external rotation (IR/ER, purple) is fixed to the femoral mechanical axis (connecting the hip and knee joint centres). The axis for ab/adduction (Ab/Ad, red) is floating and varies with hip flexion; ab/adduction moves the knee away-from/towards the sagittal plane (without changing the angle of hip flexion or rotation).
For each specimen, the ROM, with the joint capsule intact, was established by applying 5 Nm flexion/extension torques with the hip joint in neutral rotation and ab/adduction to define angles of maximum hip flexion (FLX) and extension (EXT). Then, with the joint still in neutral rotation, 5 Nm ab/adduction torques were applied at different positions of hip flexion (EXT, 0°, 30°, 60°, 90° and FLX) to define maximum hip adduction (ADD) and abduction (ABD) at each of the six flexion angles. Finally, 5 Nm torques were applied in external/internal rotation at all different positions of the hip (ADD, 0° and ABD at all six flexion/extension angles) to define maximum hip internal and external rotation (IR and ER, respectively) for all 18 positions. Importantly, throughout testing the joint was never rotated beyond the angles determined by these initial 5 Nm passive resistance boundaries, such that when a ligament was resected, the remaining ligaments did not experience increased strain.
One of the following ligaments was then resected: pubofemoral, medial arm of iliofemoral, lateral arm of iliofemoral, ischiofemoral or ligamentum teres. For each of the 18 angular positions of flexion/extension and ab/adduction, the torque required to rotate the joint back to the intact IR and ER angular positions was measured. This process was then repeated after cutting another ligament, until no ligaments remained. With all the ligaments resected, the passive resistance caused by labral impingement was measured in the same way. The cutting order for the capsular ligaments was varied, such that each ligament was cut first and last at least once in different specimens, with no pattern to the cutting order; however, the intra-articular ligamentum teres had to be resected after the capsular ligaments had been divided, as the joint had to be dislocated to gain access. The principle of superposition was used to determine the contribution to rotational restraint of each ligament and the labrum by calculating the negative change in passive resistance torque after a ligament was cut as a percentage of the intact passive resistance (5 Nm).23,24
Pilot testing showed that in some cases, the native hip could dislocate after division of the capsular ligaments for some of the positions tested. If no capsular ligaments remained and adjacent non-dislocating trials had zero passive rotation resistance, a zero value was assumed for the dislocating trial as well. When this was not the case, subsequent measurements were taken by running the vertical axis of the servohydraulic machine in position control (instead of load control). This resulted in small fluctuations of the otherwise constant applied force (variations of 70 N to 110 N magnitude and 20° to 32° angle). In total, dislocation affected five of the nine hips but only 2.8% (52/1836) of the measurements made.
Statistical analysis
Internal and external rotation contributions were analysed as separate dependent variables using SPSS software version 21 (SPSS Inc., Chicago, Illinois) and a two-way repeated measures analysis of variance (RMANOVA) with independent variables of soft-tissue state and hip position. Post hoc one-way RMANOVAs and paired t-tests with a Bonferroni correction were applied when differences across tests were found, and significance was set to p < 0.05.
Two analyses were performed: first, the respective contributions of extra- and intra-articular tissues (capsule, labrum and ligamentum teres) were compared against each other to determine their relative importance. Second, the effects of cutting the soft tissues (iliofemoral medial/lateral arms, ischiofemoral, pubofemoral, labrum and ligamentum teres) were examined to determine where each tissue made a significant contribution to rotational restraint.
A primary restraint was defined in each position as the tissue with the greatest statistically significant mean passive resistance contribution, and secondary stabilisers as any other ligaments with statistically significant mean contributions > 10%.
Results
External rotation data for one female specimen were lost owing to a pull-out failure of the lateral iliofemoral ligament at its femoral attachment under the 5 Nm external rotation torque when the hip was in a position of full flexion and full abduction. Therefore, the results presented are for eight specimens in external rotation and nine in internal rotation, resulting in a total of 1836 torque measurements. An example of the resistive torque measured for a specimen in a single position as the ligaments were cut away is given in Figure 3.
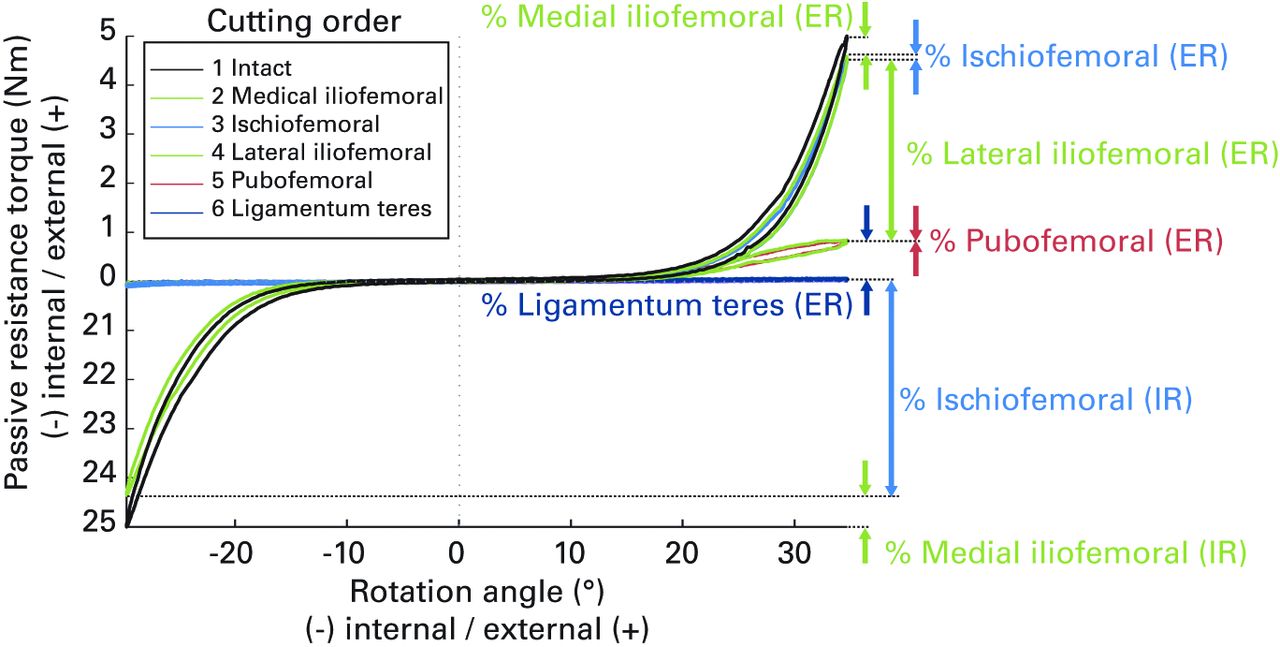
Fig. 3
A graph of the internal/external rotation cycles for one specimen positioned at 60° flexion and full adduction showing the change in torque as different ligaments are resected. Percentage changes not labelled (including the labrum) all lie on the zero torque axis. It can be seen that cutting the ischiofemoral and lateral iliofemoral ligaments caused the largest respective drops in internal and external passive rotation resistance.
The capsular ligaments provided primary internal and external rotation restraint throughout a complete range of hip movement (Fig. 4), protecting the hip from labral impingement. The contribution of the ligaments was significantly greater than that from the labrum and ligamentum teres in all hip positions (p < 0.05; Tables I and II). Labral impingement only provided secondary restraint in the native hip, and only when it was positioned in: high flexion (≥ 60°), full abduction and internal rotation; in low flexion (≤ 0°), full abduction and external rotation; or in extension, any ad/abduction and external rotation (Fig. 4). The ligamentum teres also had a small secondary role restraining external rotation when the hip was in high flexion (≥ 60°) with neutral/full adduction (Fig. 4).25
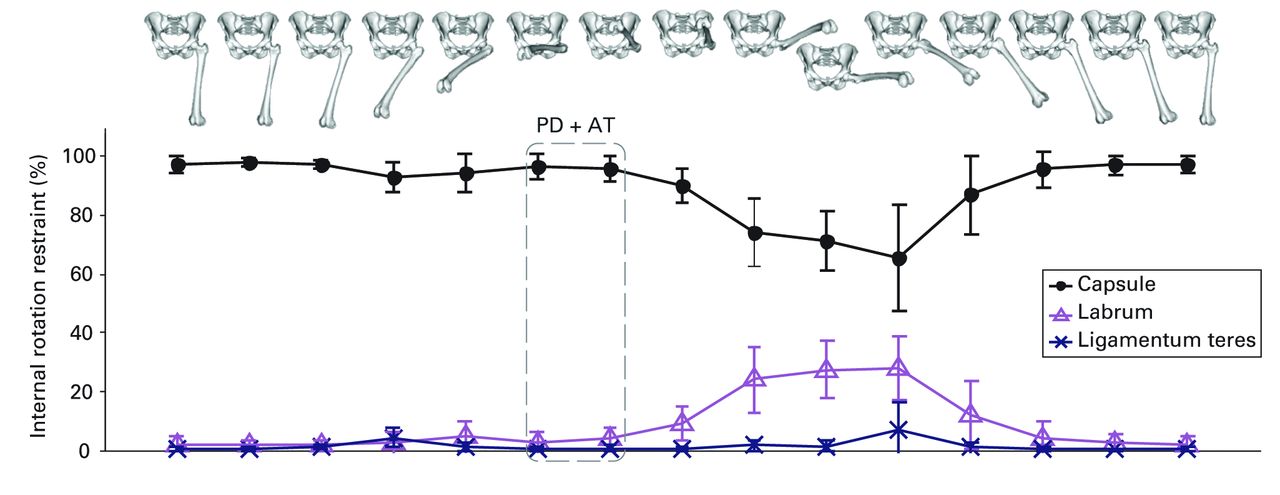
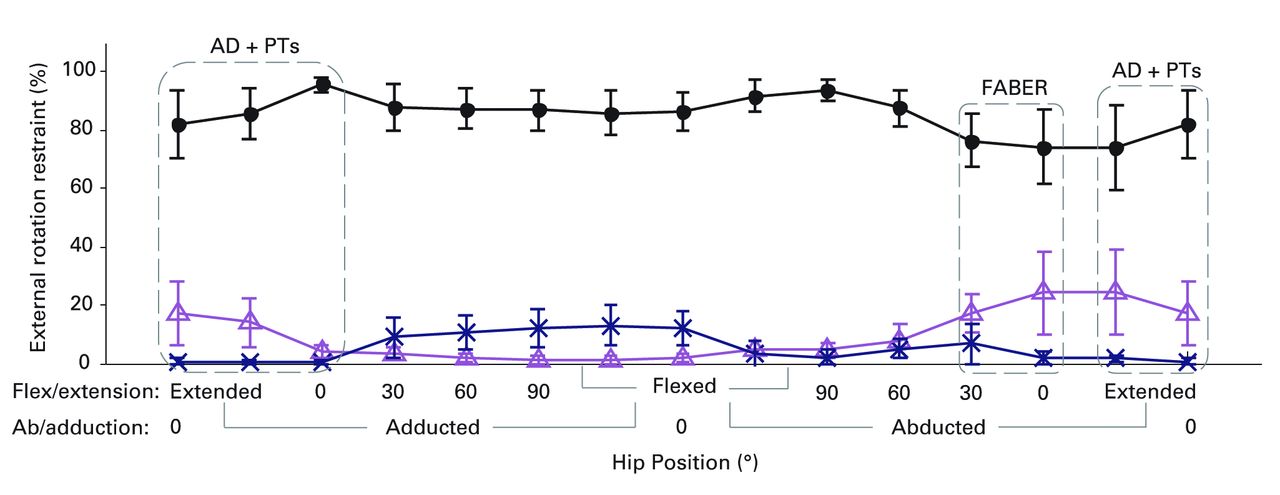
Figs. 4a - 4b
Graphs showing the mean percentage internal (a) and external (b) rotation restraint with 95% confidence intervals provided by the hip capsule, labrum and ligamentum teres for a complete range of movement. The images illustrate the hip positions on thex-axis. The dashed boxes highlight at-risk positions for anterior/posterior dislocation (AD/PD) in total hip arthroplasty,12 and physical tests for femoracetabular impingement including the anterior impingement test (AT), hyperextension, log-roll and posterior impingement tests (PTs) and the end-point of a FABER test.23
Table I
The mean and standard deviation (sd) percentage contributions of the capsular ligaments (C), the ligamentum teres (LT) and the labrum (L) to internal rotation restraint in all hip positions.
Hip position (°) | Capsular ligaments (%) | Ligamentum teres (%) | Labrum (%) | C vs LT (p-value) | C vs L (p-value) | LT vs L (p-value) | |
---|---|---|---|---|---|---|---|
Extended | Add | 98 (sd 2) | 0 (sd 1) | 1 (sd 1) | < 0.001* | < 0.001* | 0.195 |
0 | 97 (sd 4) | 1 (sd 1) | 2 (sd 3) | < 0.001* | < 0.001* | 0.337 | |
Abd | 97 (sd 4) | 0 (sd 1) | 2 (sd 4) | < 0.001* | < 0.001* | 0.201 | |
0° | Add | 98 (sd 2) | 1 (sd 1) | 1 (sd 1) | < 0.001* | < 0.001* | 1.000 |
0 | 96 (sd 8) | 0 (sd 1) | 3 (sd 6) | < 0.001* | < 0.001* | 0.391 | |
Abd | 96 (sd 8) | 0 (sd 1) | 4 (sd 8) | < 0.001* | < 0.001* | 0.467 | |
30° | Add | 93 (sd 6) | 4 (sd 4) | 3 (sd 5) | < 0.001* | < 0.001* | 1.000 |
0 | 93 (sd 16) | 1 (sd 3) | 6 (sd 13) | < 0.001* | < 0.001* | 0.510 | |
Abd | 87 (sd 17) | 1 (sd 2) | 12 (sd 15) | < 0.001* | < 0.001* | 0.115 | |
60° | Add | 94 (sd 9) | 1 (sd 2) | 5 (sd 7) | < 0.001* | < 0.001* | 0.371 |
0 | 83 (sd 19) | 3 (sd 6) | 13 (sd 14) | < 0.001* | 0.001* | 0.084 | |
Abd | 66 (sd 24) | 7 (sd 12) | 28 (sd 14) | 0.003* | 0.046* | 0.003* | |
90° | Add | 97 (sd 6) | 0 (sd 1) | 3 (sd 5) | < 0.001* | < 0.001* | 0.254 |
0 | 74 (sd 16) | 5 (sd 9) | 21 (sd 17) | < 0.001* | 0.003* | 0.169 | |
Abd | 71 (sd 13) | 1 (sd 2) | 27 (sd 13) | < 0.001* | 0.003* | 0.001* | |
Flexed | Add | 95 (sd 6) | 1 (sd 1) | 4 (sd 5) | < 0.001* | < 0.001* | 0.185 |
0 | 90 (sd 8) | 1 (sd 1) | 9 (sd 8) | < 0.001* | < 0.001* | 0.022* | |
Abd | 74 (sd 15) | 2 (sd 2) | 24 (sd 15) | < 0.001* | 0.003* | 0.006* | |
-
* Significant differences between these contributions detected by the post-hoc paired t-tests with a Bonferroni correction Abd, abduction; Add, adduction
Table II
The mean and standard deviation (sd) percentage contributions of the capsular ligaments (C), the ligamentum teres (LT) and the labrum (L) to external rotation restraint in all hip positions.
Hip position (°) | Capsular ligaments (%) | Ligamentum teres (%) | Labrum (%) | C vs LT (p-value) | C vs L (p-value) | LT vs L (p-value) | |
---|---|---|---|---|---|---|---|
Extended | Add | 85 (sd 10) | 0 (sd 1) | 14 (sd 10) | < 0.001* | < 0.001* | 0.012* |
0 | 82 (sd 14) | 1 (sd 1) | 17 (sd 13) | < 0.001* | 0.001* | 0.017* | |
Abd | 74 (sd 17) | 2 (sd 1) | 25 (sd 16) | < 0.001* | 0.012* | 0.018* | |
0° | Add | 95 (sd 3) | 1 (sd 1) | 4 (sd 3) | < 0.001* | < 0.001* | 0.055 |
0 | 82 (sd 17) | 0 (sd 1) | 18 (sd 17) | < 0.001* | 0.003* | 0.056 | |
Abd | 74 (sd 15) | 2 (sd 3) | 24 (sd 16) | < 0.001* | 0.009* | 0.028* | |
30° | Add | 88 (sd 9) | 9 (sd 8) | 3 (sd 2) | < 0.001* | < 0.001* | 0.164 |
0 | 88 (sd 10) | 6 (sd 7) | 6 (sd 4) | < 0.001* | < 0.001* | 1.000 | |
Abd | 76 (sd 11) | 7 (sd 8) | 17 (sd 8) | < 0.001* | < 0.001* | 0.129 | |
60° | Add | 87 (sd 8) | 11 (sd 7) | 2 (sd 1) | < 0.001* | < 0.001* | 0.014* |
0 | 83 (sd 16) | 13 (sd 17) | 4 (sd 3) | 0.002* | < 0.001* | 0.493 | |
Abd | 88 (sd 7) | 5 (sd 4) | 7 (sd 7) | < 0.001* | < 0.001* | 1.000 | |
90° | Add | 87 (sd 9) | 12 (sd 8) | 1 (sd 1) | < 0.001* | < 0.001* | 0.008* |
0 | 83 (sd 20) | 16(sd 20) | 2 (sd 2) | 0.006* | < 0.001* | 0.279 | |
Abd | 94 (sd 4) | 2 (sd 3) | 4 (sd 3) | < 0.001* | < 0.001* | 0.595 | |
Flexed | Add | 86 (sd 9) | 13 (sd 9) | 1 (sd 1) | < 0.001* | < 0.001* | 0.011* |
0 | 86 (sd 7) | 12 (sd 7) | 2 (sd 2) | < 0.001* | < 0.001* | 0.013* | |
Abd | 92 (sd 6) | 3 (sd 6) | 5 (sd 4) | < 0.001* | < 0.001* | 1.000 | |
-
* Significant differences between these contributions detected by the post hoc paired t-tests with a Bonferroni correction Abd, abduction; Add, adduction
The contribution of the capsular ligaments was not uniform: the ischiofemoral ligament provided primary internal rotation restraint in more than half the tested positions and was particularly prominent in high flexion (≥ 30°), adduction and internal rotation, or low flexion (≤ 30°), abduction and internal rotation (Fig. 5). The iliofemoral ligament provided primary external rotation restraint in all hip positions, and both primary internal and external rotation restraint when the hip was extended or in neutral hip flexion (Fig. 5). The medial arm of the iliofemoral ligament dominated in neutral flexion or extension, and the lateral arm in all other positions (Fig. 5). The pubofemoral ligament only contributed significantly to rotational restraint when the hip was fully abducted, contributing to external rotation restraint in extension and internal rotation restraint in hip flexion (Fig. 5).
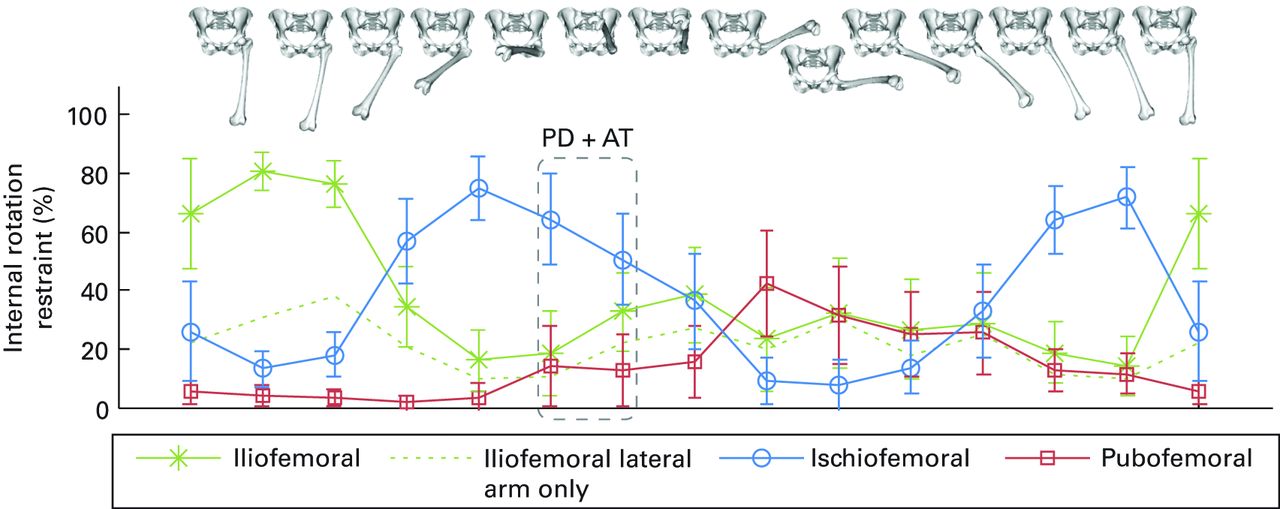
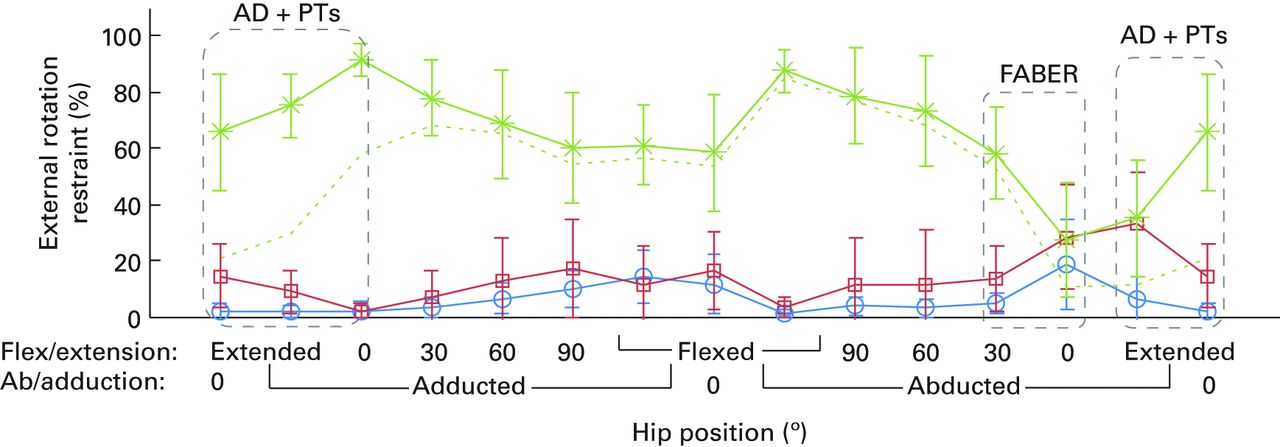
Figs. 5a - 5b
Graphs showing mean percentage contributions to internal (a) and external (b) rotation restraint with 95% confidence intervals provided by individual capsular ligaments for a complete range of hip movement. The images illustrate the hip positions on thex-axis and the dashed boxes are equivalent to those shown in Figure 4.
Dislocations were only recorded in high flexion (≥ 60°) with neutral/full adduction and only after resection of the ischiofemoral ligament, indicating that tension in this ligament as it wrapped around the femoral head provided a net force that restrained the head from subluxing from the acetabulum (Fig. 6). For one hip dislocation occurred in high flexion and adduction after resection of the joint capsule, but ceased to occur following resection of the ligamentum teres; the ligamentum teres was wrapping around the posterior femoral head in external rotation, causing the head to sublux laterally and inferiorly out of the acetabulum, in a slingshot-like mechanism. This specific hip specimen had an unusually high proportional contribution to external rotation from the ligamentum teres; however, non-dislocating subluxations caused by the ligamentum teres in high flexion and adduction were also observed in other hips following resection of the joint capsule.
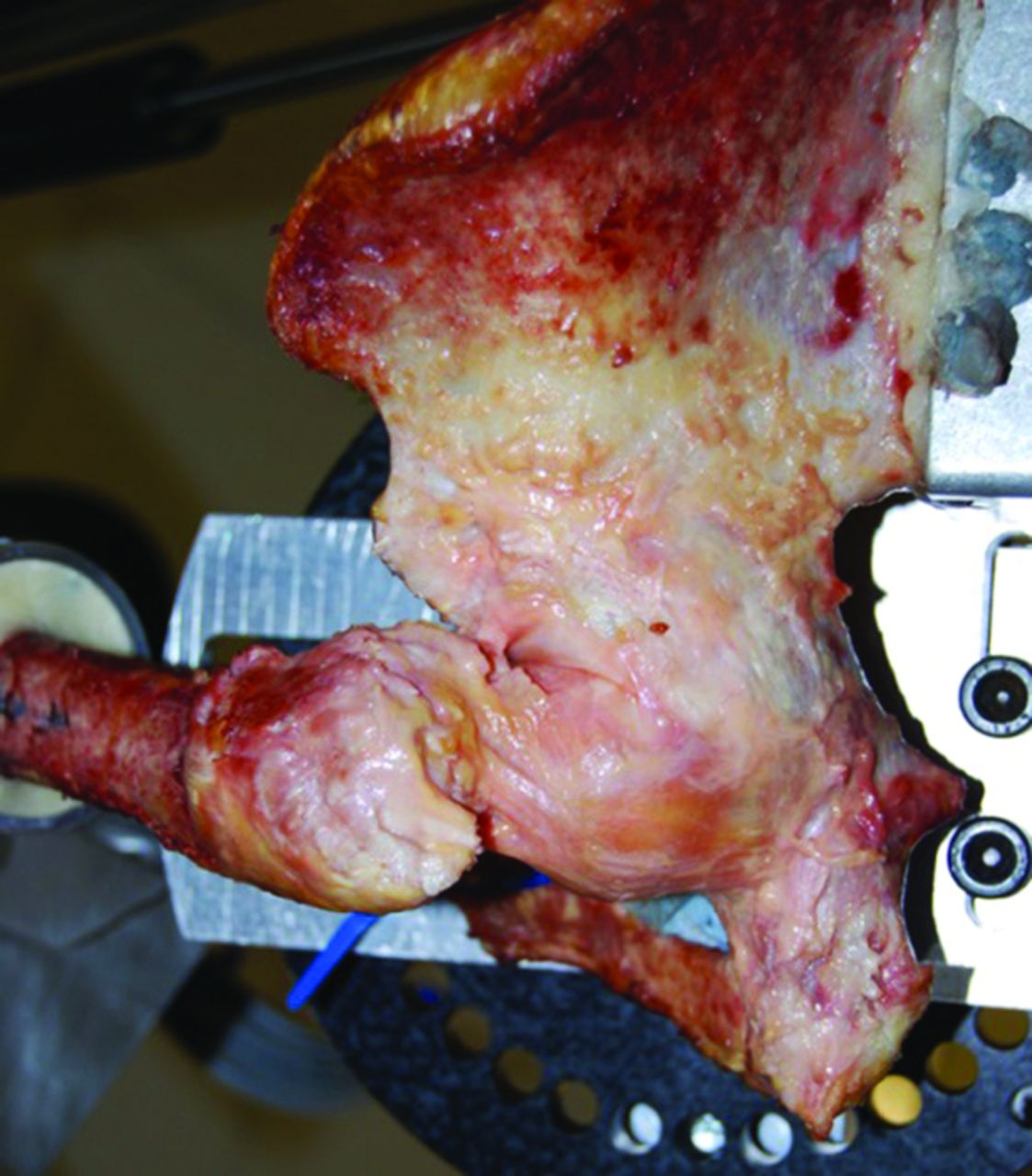
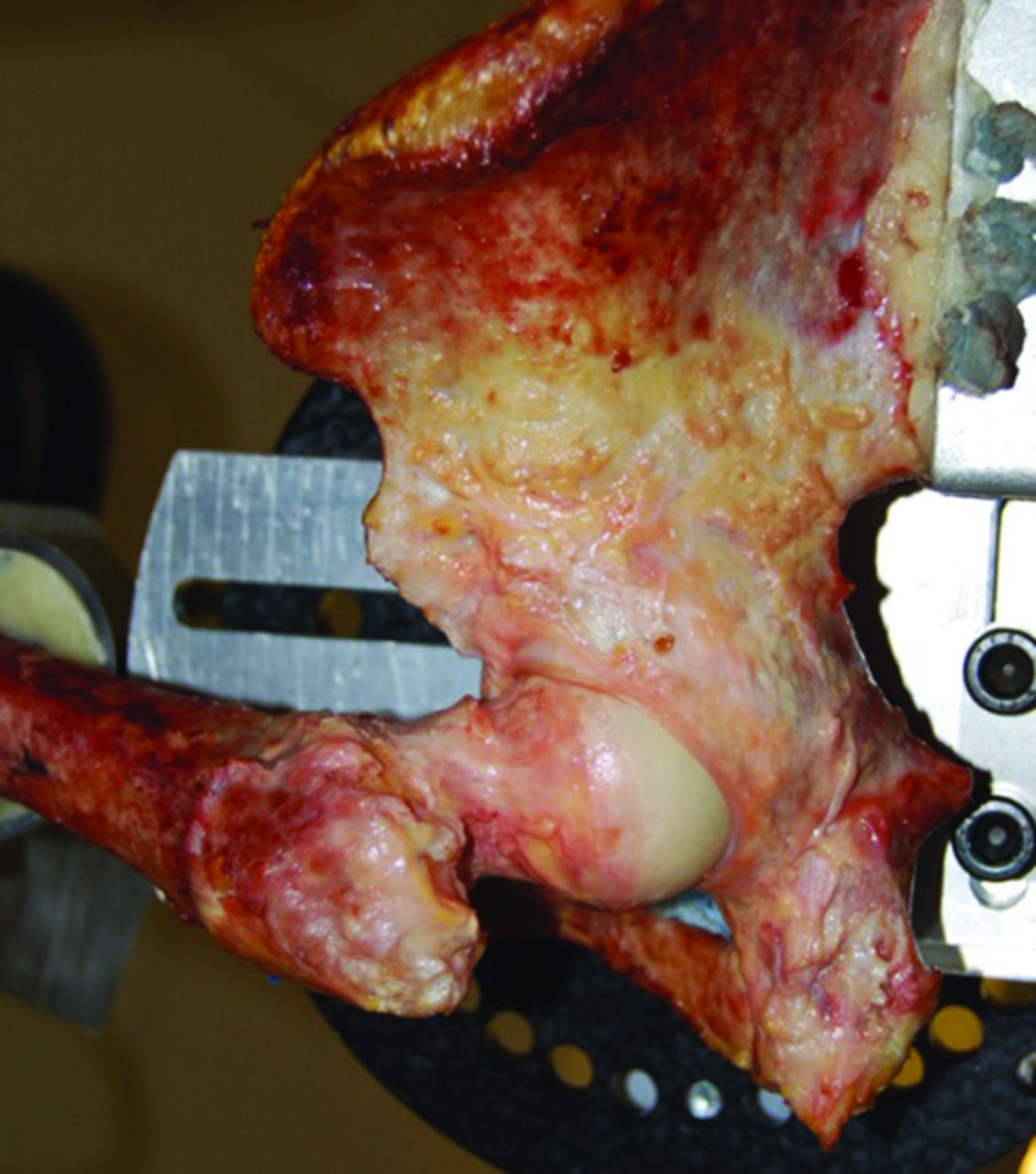
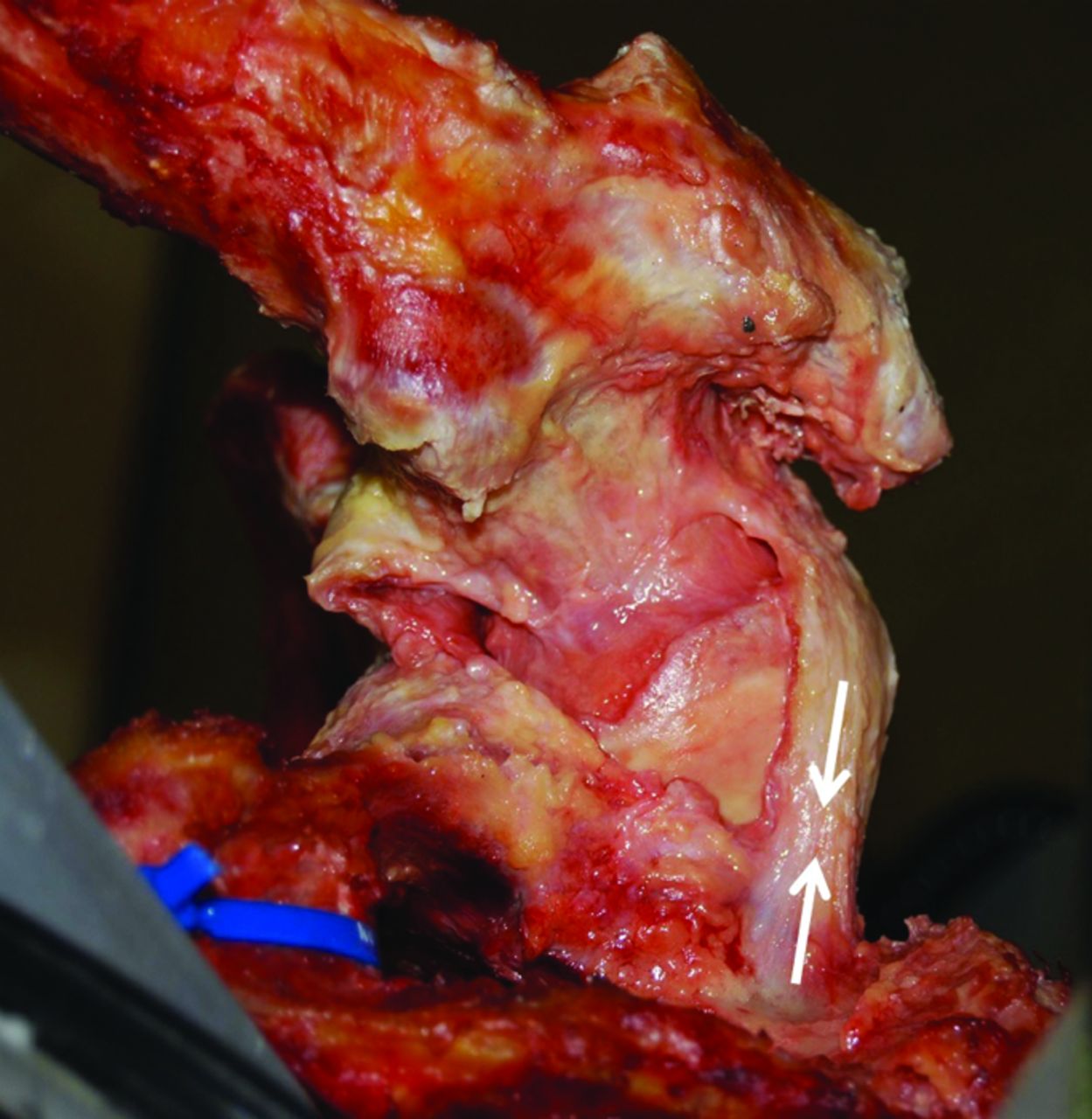
Figs. 6a - 6c
Photographs showing posterolateral views (a and b) and inferior view (c) of a hip in deep flexion with adduction and internal rotation. (a) The ischiofemoral ligament fibres are taut and wrap around the femoral head, stabilising the hip against excessive internal rotation and, thus, reducing the risk of impingement while supporting the femoral head in a sling. (b) When the ligament is resected the internal rotation restraint is lost and the femoral head is exposed, with little support from the acetabulum and labrum. (c) Tension in the ischiofemoral ligament (white arrows) pulls the femoral head into the acetabulum, stabilising the hip.
Discussion
This was a small study using cadavers and, therefore, conclusions should be applied clinically with caution. However, we show a difference in importance between structures, thereby overturning the null hypothesis with confidence.
Capsular ligaments provide primary rotational restraint and prevent labral impingements around the limits of a complete range of hip movement (Fig. 4). Within the capsule, the ischiofemoral and iliofemoral ligaments are the dominant restraints for internal and external rotation, respectively, in more than two-thirds of the possible hip positions (Fig. 5). The ischiofemoral ligament also cradles the femoral head in deep hip flexion, stabilising the hip against posterior subluxation and dislocation (Fig. 6). Damage to these two ligaments in particular may possibly predispose to or be a cause of labral impingement, hip instability or damaging loads to the articular cartilage. These ligaments should be preserved as a priority during surgery to maintain/restore normal hip mechanics, particularly if early restoration of function is essential following joint preservation surgery.
Labral impingement only contributed significantly to resisting hip rotation in a limited number of positions by providing a soft buffer against bony impingement. These labral impingements triggered small subluxations of the femoral head out of the acetabulum. However, the restraint provided by the labrum was always less than the sum of contributions from the capsular ligaments (Fig. 4). Thus, the capsule protects the labrum against the full force of impingement by providing most of the rotational restraint. Release of the posterosuperior capsule during hip arthroscopy could therefore potentially increase the post-operative load on the labrum.
The ligamentum teres has a small role in limiting external rotation, but its contribution is considerably less than that of the lateral iliofemoral ligament (Fig. 4), indicating that repair of the ligamentum teres to restore native restraint should only be considered second to repair of any deficiencies in the anterior joint capsule. In the complete absence of the joint capsule, the ligamentum teres can wrap around the femoral head and cause a destabilising subluxation in high flexion, adduction and external rotation, potentially leading to dislocation. Thus, with the capsular ligaments intact, this destabilising subluxation, caused by ligamentum teres tension, will be resisted by opposing tension from the capsular ligaments, resulting in increased joint stiffness with high passive stability.
By denuding the hip of its surrounding soft tissues with the exception of the capsule this study was limited, measuring only the contributions of the capsular ligaments, the ligamentum teres and the labrum. Any resistance caused by other soft tissues, such as muscles or fat, could not be assessed. Only a small number of specimens were included, and although visual observation suggested subjectively that the primary restraints for both male and female hips were similar, larger numbers would be needed to draw a significant conclusion on any differences between the genders. We also only modelled a single compressive loading condition whereas in vivo the magnitude and direction of this force varies for different activities19 and could act to increase or decrease subluxation and loading on the labrum. Another limitation is that the tests were performed on cadaveric specimens with a mean age greater than that of patients undergoing hip arthroscopy, so the true contribution of the ligamentum teres in these patients may be higher than measured here, as the ligamentum can degenerate with age.26 An intact capsule was necessary for ligamentum teres tension to develop without subluxation, and so our measurement of ligamentum teres contributions may be artificially reduced owing to cutting order bias, having for practical reasons always been resected after the capsular ligaments.
For the joint capsule, these results agree with a laxity-based cadaveric model which quantified their function in low flexion/extension.16 They also agree with a detailed anatomical description of where the ligaments are taut throughout a complete ROM.27 As for the labrum, the results corroborate a study which found that it only provides secondary stability in low hip flexion/extension, with primary stability coming from the iliofemoral ligament.18 The position of deep flexion, full abduction and internal rotation where labral impingements were recorded in this study correlate well with those measured in ballet dancers with normal hip anatomy and normal passive range of hip movement during extreme dancing positions.28 For the ligamentum teres, the findings support some results from a simple string model5 and arthroscopic observations that it tightens in flexion, adduction and external rotation.29 Recent work20,21 also suggested that it has a role in preventing hip subluxation in high flexion and abduction, and low flexion/extension and external rotation; in these positions we found that the hip was indeed at risk of impingement and subluxation (Fig. 4), but did not find that the ligamentum teres provided consistent rotational restraint.
The iliofemoral ligament may be cut during treatment of FAI when performed by either open30 or arthroscopic2 surgery. Some reports indicate that hip instability is not a problem after these procedures,31 whereas others have found that capsular defects32 and instability33 are more common in patients requiring revision hip arthroscopy, and that post-operative subluxations can be under-reported.2 This study did not find an increase in risk of dislocation after resection of the iliofemoral ligament, but the loss of primary external rotational restraint across the complete ROM (Fig. 4) could increase the load on the labrum through impingement in extension or abduction, which may be perceived as pain and possible subluxation. Thus, we advocate repair of the capsular ligaments during early intervention surgery to reduce the risk of hip subluxation and labral overloading. This is particularly relevant when treating dysplastic hips, or trimming the rim of the acetabulum to treat FAI with a cam-pincer diagnosis,34 because an deficient acetabulum increases the risk of hip instability.6,7,31
The internal rotation restraint and supporting sling protecting against subluxation in deep hip flexion (Figs 5 and 6) offered by the ischiofemoral ligament could benefit patients who have undergone THA by passively stabilising the hip in a position where few muscles have favourable lines of action.35 This may reduce the risk of dislocation but could also prevent posterior edge loading in THA. Posterior edge loading can lead to damage of the bearing surfaces and has been identified as a causative factor in ceramic hip squeaking.12 The restraint provided by the ischiofemoral ligament likely increases with increasing bearing couple sizes as tension develops in the ligament as it wraps around the femoral head (Fig. 6): less subluxation means less edge loading,13 but also a reduction in the pumping action that potentially expels joint fluid and edge loading wear debris into neighbouring soft tissues.36 This study therefore supports repair of the ischiofemoral ligament after using a posterior approach for THA, particularly if a larger-diameter bearing couple is used.
In conclusion, the iliofemoral and ischiofemoral capsular ligaments are important passive rotational restraints to hip movement. The integrity of these ligaments appears to be important for maintaining normal hips mechanics and preventing excessive hip rotation. Preservation of them may prevent both impingement and hip instability.
1 Bedi A , GalanoG, WalshC, KellyBT. Capsular management during hip arthroscopy: from femoroacetabular impingement to instability. Arthroscopy2011;27:1720–1731.CrossrefPubMed Google Scholar
2 Domb BG , PhilipponMJ, GiordanoBD. Arthroscopic capsulotomy, capsular repair, and capsular plication of the hip: relation to atraumatic instability. Arthroscopy2013;29:162–173.CrossrefPubMed Google Scholar
3 Bedi A , KellyBT, KhandujaV. Arthroscopic hip preservation surgery: current concepts and perspective. Bone Joint J2013;95-B:10–19.CrossrefPubMed Google Scholar
4 Phillips AR , BartlettG, NortonM, FernD. Hip stability after ligamentum teres resection during surgical dislocation for cam impingement. Hip Int2012;22:329–334.CrossrefPubMed Google Scholar
5 Martin RL , PalmerI, MartinHD. Ligamentum teres: a functional description and potential clinical relevance. Knee Surg Sports Traumatol Arthrosc2012;20:1209–1214.CrossrefPubMed Google Scholar
6 Mei-Dan O , McConkeyMO, BrickM. Catastrophic failure of hip arthroscopy due to iatrogenic instability: can partial division of the ligamentum teres and iliofemoral ligament cause subluxation?Arthroscopy2012;28:440–445.CrossrefPubMed Google Scholar
7 Benali Y , KatthagenBD. Hip subluxation as a complication of arthroscopic debridement. Arthroscopy2009;25:405–407.CrossrefPubMed Google Scholar
8 Sansone M , AhldénM, JónassonP, et al.Total dislocation of the hip joint after arthroscopy and ileopsoas tenotomy. Knee Surg Sports Traumatol Arthrosc2013;21:420–423.CrossrefPubMed Google Scholar
9 Matsuda DK . Acute iatrogenic dislocation following hip impingement arthroscopic surgery. Arthroscopy2009;25:400–404.CrossrefPubMed Google Scholar
10 Ranawat AS , McClincyM, SekiyaJK. Anterior dislocation of the hip after arthroscopy in a patient with capsular laxity of the hip; a case report. :. J Bone Joint Surg [Am]2009;91-A:192–197. Google Scholar
11 Austin DC , Horneff IiiJG, Kelly IvJD. Anterior hip dislocation 5 months after hip arthroscopy. Arthroscopy2014;30-10:1380–1382.CrossrefPubMed Google Scholar
12 Esposito CI , WalterWL, RoquesA, et al.Wear in alumina-on-alumina ceramic total hip replacements: a retrieval analysis of edge loading. J Bone Joint Surg [Br]2012;94-B:901–907.CrossrefPubMed Google Scholar
13 De Haan R , CampbellPA, SuEP, De SmetKA. Revision of metal-on-metal resurfacing arthroplasty of the hip: the influence of malpositioning of the components. J Bone Joint Surg [Br]2008;90-B:1158–1163.CrossrefPubMed Google Scholar
14 Nadzadi ME , PedersenDR, YackHJ, CallaghanJJ, BrownTD. Kinematics, kinetics, and finite element analysis of commonplace maneuvers at risk for total hip dislocation. J Biomech2003;36:577–591.CrossrefPubMed Google Scholar
15 Kwon MS , KuskowskiM, MulhallKJ, et al.Does surgical approach affect total hip arthroplasty dislocation rates?Clin Orthop Relat Res2006;447:34–38.CrossrefPubMed Google Scholar
16 Martin HD , SavageA, BralyBA, et al.The function of the hip capsular ligaments: a quantitative report. Arthroscopy2008;24:188–195.CrossrefPubMed Google Scholar
17 Safran MR , LopomoN, ZaffagniniS, et al.In vitro analysis of peri-articular soft tissues passive constraining effect on hip kinematics and joint stability. Knee Surg Sports Traumatol Arthrosc2013;21:1655–1663.CrossrefPubMed Google Scholar
18 Myers CA , RegisterBC, LertwanichP, et al.Role of the acetabular labrum and the iliofemoral ligament in hip stability: an in vitro biplane fluoroscopy study. Am J Sports Med2011;3(9Suppl):85S–91S.CrossrefPubMed Google Scholar
19 Bergmann G , DeuretzbacherG, HellerM, et al.Hip contact forces and gait patterns from routine activities. J Biomech2001;34:859–871.CrossrefPubMed Google Scholar
20 Martin RL , KivlanBR, ClementeFR. A cadaveric model for ligamentum teres function: a pilot study. Knee Surg Sports Traumatol Arthrosc2013;21:1689–1693.CrossrefPubMed Google Scholar
21 Kivlan BR , RichardClemente F, MartinRL, MartinHD. Function of the ligamentum teres during multi-planar movement of the hip joint. Knee Surg Sports Traumatol Arthrosc2013;21:1664–1668.CrossrefPubMed Google Scholar
22 Wu G , SieglerS, AllardP, et al.ISB recommendation on definitions of joint coordinate system of various joints for the reporting of human joint motion--part I: ankle, hip, and spine. International Society of Biomechanics. J Biomech2002;35:543–548.CrossrefPubMed Google Scholar
23 Amis AA , DawkinsGP. Functional anatomy of the anterior cruciate ligament. Fibre bundle actions related to ligament replacements and injuries. J Bone Joint Surg [Br]1991;73-B:260–267.CrossrefPubMed Google Scholar
24 Xerogeanes JW , TakedaY, LivesayGA, et al.Effect of knee flexion on the in situ force distribution in the human anterior cruciate ligament. Knee Surg Sports Traumatol Arthrosc1995;3:9–13.CrossrefPubMed Google Scholar
25 Tijssen M , van CingelR, WillemsenL, de VisserE. Diagnostics of femoro-acetabular impingement and labral pathology of the hip: a systematic review of the accuracy and validity of physical tests. Arthroscopy2012;28:860–871. Google Scholar
26 Tan CK , WongWC. Absence of the ligament of head of femur in the human hip joint. Singapore Med J1990;31:360–363.PubMed Google Scholar
27 Fuss FK , BacherA. New aspects of the morphology and function of the human hip joint ligaments. Am J Anat1991;192:1–13.CrossrefPubMed Google Scholar
28 Charbonnier C , KoloFC, DuthonVB, et al.Assessment of congruence and impingement of the hip joint in professional ballet dancers: a motion capture study. Am J Sports Med2011;39:557–566.CrossrefPubMed Google Scholar
29 Kelly BT , WilliamsRJ 3rd, PhilipponMJ. Hip arthroscopy: current indications, treatment options, and management issues. Am J Sports Med2003;31:1020–1037.CrossrefPubMed Google Scholar
30 Leunig M , GanzR. The evolution and concepts of joint-preserving surgery of the hip. Bone Joint J2014;96-B:5–18.CrossrefPubMed Google Scholar
31 Ilizaliturri VM Jr . Complications of arthroscopic femoroacetabular impingement treatment: a review. Clin Orthop Relat Res2009;467:760–768.CrossrefPubMed Google Scholar
32 McCormick F , SlikkerW 3rd, HarrisJD, et al.Evidence of capsular defect following hip arthroscopy. Knee Surg Sports Traumatol Arthrosc2014;22:902–905.CrossrefPubMed Google Scholar
33 Philippon MJ , SchenkerML, BriggsKK, et al.Revision hip arthroscopy. Am J Sports Med2007;35:1918–1921. Google Scholar
34 Masjedi M , NightingaleCL, AzimiDY, CobbJP. The three-dimensional relationship between acetabular rim morphology and the severity of femoral cam lesions. Bone Joint J2013;95-B:314–319.CrossrefPubMed Google Scholar
35 van Arkel RJ , ModeneseL, PhillipsAT, JeffersJR. Hip abduction can prevent posterior edge loading of hip replacements. J Orthop Res2013;31:1172–1179.CrossrefPubMed Google Scholar
36 Wroblewski BM , SineyPD, FlemingPA. Microseparation, fluid pressure and flow in failures of metal-on-metal hip resurfacing arthroplasties. Bone Joint Res2012;1:25–30.CrossrefPubMed Google Scholar
Author contributions
R. J. van Arkel: Design of experiment, Testing, data analysis, Statistical analysis, Writing the paper.
A. A. Amis: Design of the experiment, Writing the paper.
J. P. Cobb: Design of the experiment, Writing the paper.
J. R.T. Jeffers: Design of the experiment, Data analysis, Writing the paper.
This study was funded by the Wellcome Trust and EPSRC [088844/Z/09/Z] and the Institution of Mechanical Engineers. The dual-axis Instron materials testing machine was provided by an equipment grant from Arthritis Research UK.
No benefits in any form have been received or will be received from a commercial party related directly or indirectly to the subject of this article.
This article was primary edited by D. Johnstone and first proof edited by G. Scott.