Abstract
Stem cells are a key component of regenerative medicine strategies. Particular areas of musculoskeletal application include cartilage and bone regeneration in arthritis and trauma. There are several types of stem cell and this article will focus on the adult derived cells. The review includes current issues and future developments.
Tissue engineering emerged in the early 1990s as an “interdisciplinary science involving life sciences and engineering to bring about biological substitutes that restore, maintain, or improve tissue function”, with cartilage, bone and muscle identified as therapeutic targets.1 In recent years, a new field of regenerative medicine has been developed that aims to replace or regenerate “human cells, tissue or organs, to restore or establish normal function”2 and it is in this context that cell therapy is an important orthopaedic technology. There are many cells that could be used in cell therapy but this review concerns stem cells, particularly mesenchymal stem cells, and their potential use in orthopaedic surgery.
What are stem cells?
Stem cells have unique characteristics which include the ability to differentiate into more specialist types of cell. The number of different cell types into which a stem cell can differentiate varies, and includes all cell types (totipotent), most cell types (pluripotent) or a limited number of cell types (multipotent). Cells obtained from fertilised gametes or from the morula (up to 16-cell stage) are totipotent. Embryonic stem cells (ESC), typically obtained from the inner cell mass of five- to six-day-old embryos,3 are pluripotent. Cells with similar pluripotent ability can be induced by gene transfer to cells that were not originally ESC; this type of cell is termed an induced pluripotent stem cell (iPSC). Stem cells are also found in many adult tissues, where they are commonly multipotent.4 They were originally discovered in bone marrow5 and identified as two main types, haematopoietic and mesenchymal stem cells (MSC). Cells from the haematopoietic lineage are familiar to haematologists and are vital to bone marrow transplantation. They can also be collected from peripheral blood by apheresis when the marrow is medically stimulated. This type of cell when stimulated from marrow is known as the peripheral blood stem cell (PBSC). This cell type has been used in orthopaedic practice6 and is the subject of another article in this edition of 360. The second cell type, the adult MSC, has also emerged as an attractive target for regenerative therapies. They are numerous, relatively easy to isolate and are not associated with some of the regulatory and ethical issues currently associated with ESCs.
What are mesenchymal stem cells?
The MSC is a multipotent stem cell that can differentiate easily into cell lineages relevant to orthopaedic surgery, e.g. cartilage, bone, and tendon (Fig. 1).7 In addition to bone marrow, adult MSCs have been isolated from synovial fluid,8 synovial membrane,9 periosteum,10,11 intervertebral disc end plates,12 adipose tissue and articular cartilage.13 In each location, the cells are believed to be located in a specialised microenvironment referred to as a ‘niche’, where the cell is living but may be quiescent. When specific stimuli are applied, the cells leave their respective niches, proliferate and differentiate to contribute to repair mechanisms.14 The MSC is not easily identified by simply looking down a microscope. The International Society for Cellular Therapy described minimal criteria15 for defining multipotent mesenchymal stem cells in culture. This includes adherence to plastic, an appropriate immunophenotype expression (CD73+, CD90+, CD105+, CD14- or CD11b-, CD19- or CD79α-, CD34-, CD45- and HLA-DR-), and the ability to differentiate down chondrogenic, osteoblastic and adipocytic lineages.
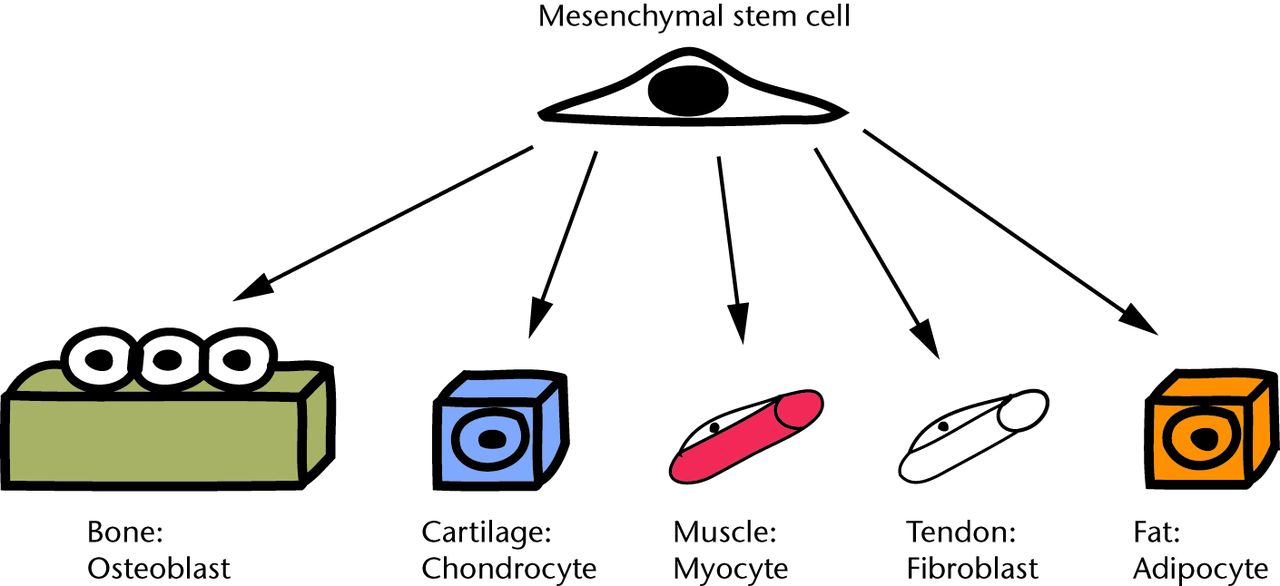
Fig. 1
Schematic diagram showing how multipotent mesenchymal stem cells (MSCs) can differentiate into musculoskeletal cell lines.
Strategies for musculoskeletal repair
Tissue engineering strategies often describe an interaction between cells, scaffold and other factors (Fig. 2). A scaffold provides a three-dimensional environment to allow the patient’s or delivered cells to bring about tissue formation. Factors, such as cell signalling molecules, can help drive the appropriate biological pathways. Scaffolds do not always require the addition of cells. Cell therapy strategies seek to use the cell as a component of the treatment, and it is in this area that the MSC has been identified as useful. The cell can be derived from the patient (autologous) and can be minimally biologically manipulated before treatment or expanded in culture. Cells can also be derived from an allogeneic source such as donors. A cell-based strategy can be used alone or combined with the scaffold and factors. There are two main concepts for achieving synthesis in tissue regeneration (Fig. 3). Synthesis outside the body combines the cells, scaffolds and factors in a laboratory environment, e.g. a bioreactor, to create tissue such as a piece of cartilage. Synthesis inside the body uses cells, scaffolds and factors such that the body itself creates the final tissue. In this approach, one or two of the classical triad may be provided by the patient rather than the treatment; ultimately, in the future, introduction of either cells alone or a factor to trigger the appropriate response from endogenous stem cells may be successful. Understanding of the theoretical approaches to the problem allows a closer examination of what treatments have been developed for the clinic.
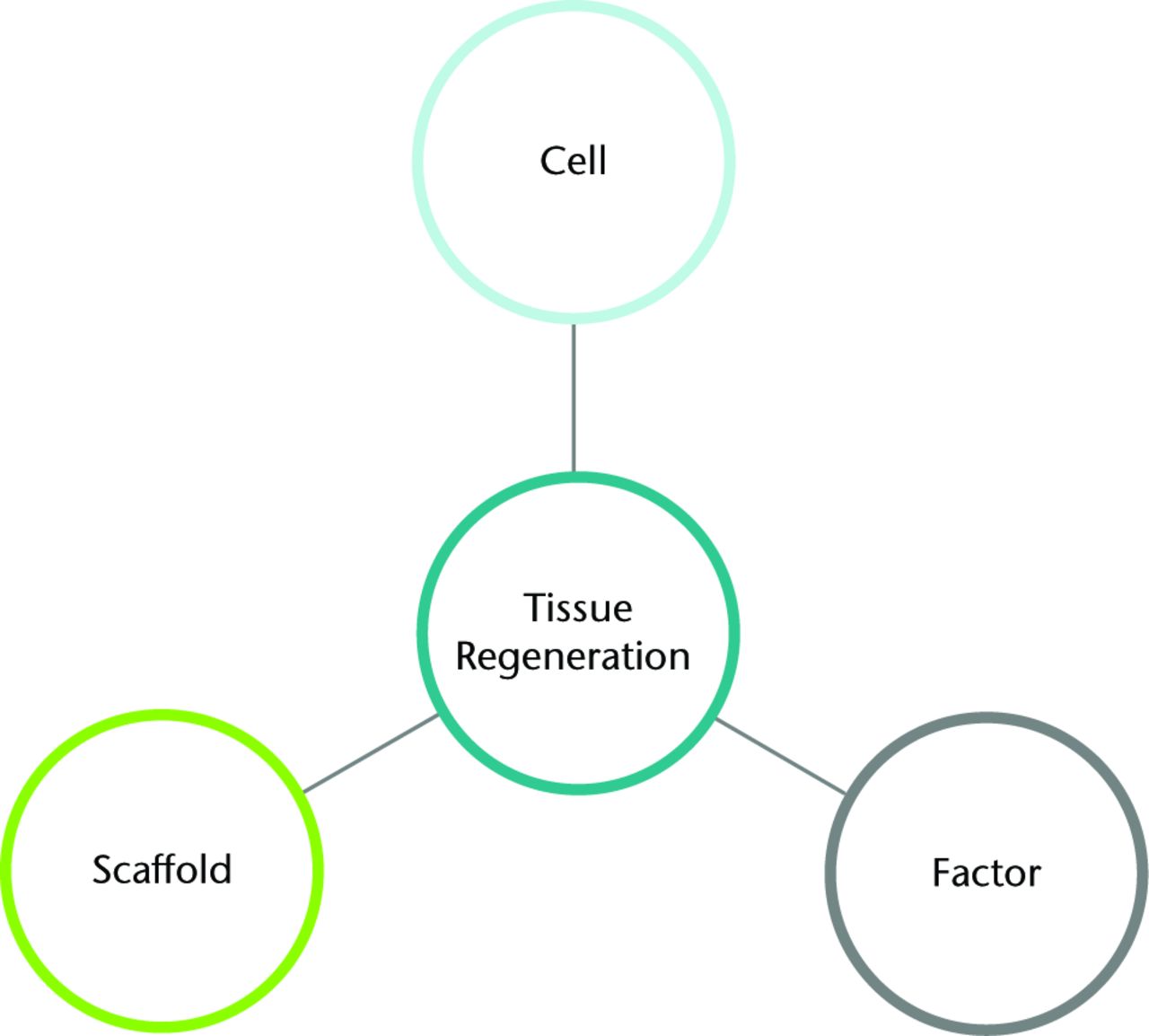
Fig. 2
Diagram showing the key parts of tissue regeneration strategy.
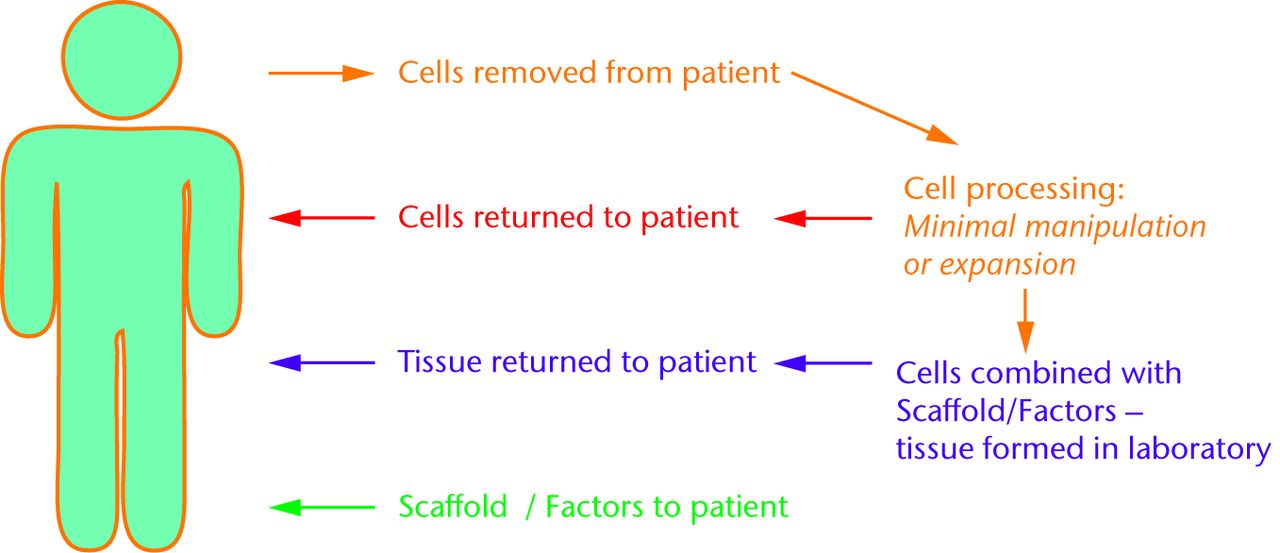
Fig. 3
Schematic showing some of the possible ways that cells (e.g. mesenchymal stem cells) could be used for tissue regeneration. Cells removed from the patient can be utilised for tissue synthesis back in the patient (red) or in the laboratory (purple). Another approach would use scaffolds and factors to work with cells in the patient without removal (green).
Articular cartilage regeneration
Cartilage repair is an important target for tissue engineering and several orthopaedic techniques have been developed. Autologous chondrocyte implantation (ACI) was described by Brittberg et al16 in 1994. The technique involves removing cartilage and isolating chondrocytes from the patient and then expanding the number of cells in the laboratory. In a second procedure, the increased number of chondrocytes is reintroduced into the cartilage lesion, under a periosteal or fabricated flap, to bring about repair. The technique does produce cartilage and can give improved knee function.17,18 Other techniques, such as microfracture, create a communication between the bone marrow and the joint interior to stimulate repair. In a recent systematic review, clinical outcomes of ACI were not found to be markedly different from microfracture.19 A better understanding of cell therapies led to the introduction of second generation ACI, where autologous chondrocytes are added to a matrix, such as hyaluronan or collagen, to simulate a three-dimensional culture environment. Mesenchymal stem cells may overcome some of the limitations of chondrocyte techniques, used either as an alternative or in conjunction with chondrocytes. A few case studies using MSCs in place of chondrocytes in humans have been reported but comparison between the studies is difficult due to lack of uniform protocols. In 2002, culture-expanded adherent MSCs seeded below periosteum in osteoarthritic cartilage defects at the time of osteotomy resulted in increased arthroscopic and histologic grading scores in 12 patients compared with 12 controls, but there was no difference in the patients’ function scores.20 In 2007 the same group21 treated three patients with auto-transplantation of culture-expanded bone marrow-derived mesenchymal cells into articular lesions of pateIlofemoral joint. Six months later the patients’ symptoms had improved. Histology was performed in one patient that showed, disappointingly, that the repaired cartilage was predominantly composed of fibrocartilage. The challenge in generating articular cartilage with MSCs, is to produce cells with features of stable chondrocytes which resist hypertrophy and terminal differentiation.22 Unstable chondrocytes may become hypertrophic resulting in mineralisation and vascular invasion, similar to the process of ectopic ossification. Transplantation of stem cells after a short induction period of one week, has been suggested to counter hypertrophy and terminal differentiation.23 Another option is the combination of a proportion of chondrocytes grown from cartilage in culture, which may help drive the MSC to a chondrocytic phenotype. Osteoarthritis can clearly involve both bone as well as cartilage and multipotent cells may well be useful. A recent study6 detailed arthroscopic subchondral drilling of knee cartilage defects, followed by five weekly intra-articular injections of stem cells (PBSC) derived from autologous peripheral blood. The authors reported arthroscopically-confirmed articular cartilage regeneration, containing proteoglycans and collagen II.6
Bone regeneration
Bone formation is a key part of many non-articular conditions and is driven by the osteoblast, which is one of the possible MSC differentiation lineages. Fracture healing requires bone formation and remodelling to achieve stable union, a process that can be delayed or absent. It has been reported that MSCs from nonunion sites have less osteogenic differentiation and mineralisation capability, at least in vitro, compared with bone marrow-derived MSCs.24 Provision of stem cells with good functional capacity could help to resolve this problem. Several animal studies report bone regeneration using bone marrow-derived MSC,25 alone, in combination with growth factors (BMP-7) or after genetic modification.26 However, human studies are extremely limited27-29 but this may well be a growing area of clinical application.
Other applications
Other aspects of MSC biology can be used therapeutically. It should be remembered that the MSC is not simply a cell that manufactures tissues, but is active in inflammation and immune modulation. Mesenchymal stem cells have been used to treat a variety of skeletal pathologies including osteogenesis imperfecta (OI), osteoporosis, infantile hypophosphatasia and bone metastases. Osteogenesis imperfecta is a genetic disorder characterised by defective production of type I collagen, leading to generalised osteopenia, multiple fractures and short stature. In one study30 six children suffering from OI received two infusions of allogeneic gene-marked, marrow-derived mesenchymal cells. The group observed nearly 50% acceleration in growth velocity during the first six months after the infusion. Besides being able to regenerate tissues, one of the attractive qualities of MSCs is their apparent ability to modulate and perhaps suppress the host’s immune system, which is at the root of auto-immune diseases. Laboratory research is showing early signs that may lead to the development of new therapies in rheumatoid arthritis31,32 and graft versus host disease.33
The stem cell niche
In living organisms, local immune homeostasis in tissues is maintained by the intricate interaction of stem cells with other neighbouring cells. Stem cells are surrounded by a complex three-dimensional network and are interconnected to a variety of cells which are also embedded within their surrounding extracellular matrix. It is now believed that replication of such a three-dimensional network surrounding stem cells, referred to as a ‘niche’, may be relevant to enhancing outcomes in clinical applications. One strategy in the future will be to develop target factors and scaffolds to stimulate recruitment and activation of a patient’s own MSCs in situ.
Safety and regulation
Our understanding of stem cell biology is increasing but is incomplete. The potential for regenerative medicine is clear but patient safety must be ensured. Regulatory governance controlling cell therapy is an evolving field. In Europe, it is now governed by the European Medicines Agency, via the Medicines and Healthcare Regulatory products Agency in the UK. Cell products are subject to the same controls, checks and licensing requirements as pharmaceutically manufactured products. The processing of cells (ex-vivo expansion and differentiation) in particular, must be carefully controlled to avoid disease transmission. In terms of differentiation, one potential problem is unwanted changes towards neoplasia. We found two studies which described the safety of using bone marrow-derived MSCs to treat cartilage defects.34,35 The first study34 reported that patients treated with MSCs had no evidence of tumours or infections in the treated joints of 41 patients between five and 137 months (mean 75) of follow-up. In the second study,35 227 patients had been treated using injection of culture-expanded autologous MSCs in the joint. Follow-up was by high field MRI tracking of the treated defect in 45 patients and general surveillance in 227 patients. No neoplastic complications were detected at any stem cell re-implantation site at a mean of 10.6 months.
Future
There is still much to be learnt about how stem cells form and repair tissue. The presence of multipotent cells, easily available in the adult, points to a potential self-regeneration tool kit in a convenient location. We need to understand more about the different preparations of such cells; minimally manipulated, culture-expanded, autologous and allogeneic forms. A better understanding not only of the cells, but also their niche, is crucially important in translating laboratory medicine to proven therapy. Local activation of the stem cells in their niche may enable orchestration of the restoration of healthy articular cartilage, bone or even other musculoskeletal tissues. The finding that MSCs are able to ‘home in’ on the area of injury and modulate the local immune environment required for regeneration and repair of the injured tissue, opens avenues for development of non-cellular pharmacological therapies.
In addition, any treatment must undergo stepwise translation to the clinic. The treatment must improve patient care and must be safe, well regulated and cost-effective. These challenges are more acute for cell therapy where the science and its applications are still relatively new. There is clearly potential in the use of MSCs in the clinic, and an integrated approach between stakeholders (patients, clinicians, regulators, healthcare providers and industry) will be required to bring this into routine practice.
1 Langer R , VacantiJP. Tissue engineering. Science1993;260:920–926.CrossrefPubMed Google Scholar
2 Mason C , DunnillP. A brief definition of regenerative medicine. Regen Med2008;3:1–5.CrossrefPubMed Google Scholar
3 Thomson JA , Itskovitz-EldorJ, ShapiroSS, et al.Embryonic stem cell lines derived from human blastocysts. Science1998;282:1145–1147.CrossrefPubMed Google Scholar
4 Pittenger MF , MackayAM, BeckSC, et al.Multilineage potential of adult human mesenchymal stem cells. Science1999;284:143–147.CrossrefPubMed Google Scholar
5 Friedenstein AJ , ChailakhjanRK, LalykinaKS. The development of fibroblast colonies in monolayer cultures of guinea-pig bone marrow and spleen cells. Cell Tissue Kinet1970;3:393–403.CrossrefPubMed Google Scholar
6 Saw KY , AnzA, MericanS, et al.Articular cartilage regeneration with autologous peripheral blood progenitor cells and hyaluronic acid after arthroscopic subchondral drilling: a report of 5 cases with histology. Arthroscopy2011;27:493–506.CrossrefPubMed Google Scholar
7 Jones EA , EnglishA, HenshawK, et al.Enumeration and phenotypic characterization of synovial fluid multipotential mesenchymal progenitor cells in inflammatory and degenerative arthritis. Arthritis Rheum2004;50:817–827.CrossrefPubMed Google Scholar
8 De Bari C , Dell'AccioF, TylzanowskiP, LuytenFP. Multipotent mesenchymal stem cells from adult human synovial membrane. Arthritis Rheum2001;44:1928–1942.CrossrefPubMed Google Scholar
9 De Bari C , Dell'AccioF, VandenabeeleF, et al.Skeletal muscle repair by adult human mesenchymal stem cells from synovial membrane. J Cell Biol2003;160:909–918.CrossrefPubMed Google Scholar
10 Nakahara H , DennisJE, BruderSP, et al.In vitro differentiation of bone and hypertrophic cartilage from periosteal-derived cells. Exp Cell Res1991;195:492–503.CrossrefPubMed Google Scholar
11 De Bari C , Dell'AccioF, LuytenFP. Human periosteum-derived cells maintain phenotypic stability and chondrogenic potential throughout expansion regardless of donor age. Arthritis Rheum2001;44:85–95.CrossrefPubMed Google Scholar
12 Liu LT , HuangB, LiCQ, et al.Characteristics of stem cells derived from the degenerated human intervertebral disc cartilage endplate. PLoS One2011;6:26285.CrossrefPubMed Google Scholar
13 Dowthwaite GP , BishopJC, RedmanSN, et al.The surface of articular cartilage contains a progenitor cell population. J Cell Sci2004;117:889–897.CrossrefPubMed Google Scholar
14 Ehninger A , TrumppA. The bone marrow stem cell niche grows up: mesenchymal stem cells and macrophages move in. J Exp Med2011;208:421–428.CrossrefPubMed Google Scholar
15 Dominici M , Le BlancK, MuellerI, et al.Minimal criteria for defining multipotent mesenchymal stromal cells: the International Society for Cellular Therapy position statement. Cytotherapy2006;8:315–317. Google Scholar
16 Brittberg M , LindahlA, NilssonA, et al.Treatment of deep cartilage defects in the knee with autologous chondrocyte transplantation. N Engl J Med1994;331:889–895.CrossrefPubMed Google Scholar
17 Saris DB , VanlauweJ, VictorJ, et al.Treatment of symptomatic cartilage defects of the knee: characterized chondrocyte implantation results in better clinical outcome at 36 months in a randomized trial compared to microfracture. Am J Sports Med2009;37(Suppl 1):10S–19S.CrossrefPubMed Google Scholar
18 Saris DB , VanlauweJ, VictorJ, et al.Characterized chondrocyte implantation results in better structural repair when treating symptomatic cartilage defects of the knee in a randomized controlled trial versus microfracture. Am J Sports Med2008;36:235–246.CrossrefPubMed Google Scholar
19 Nakamura N , MiyamaT, EngebretsenL, YoshikawaH, ShinoK. Cell-based therapy in articular cartilage lesions of the knee. Arthroscopy2009;25:531–552.CrossrefPubMed Google Scholar
20 Wakitani S , ImotoK, YamamotoT, et al.Human autologous culture expanded bone marrow mesenchymal cell transplantation for repair of cartilage defects in osteoarthritic knees. Osteoarthritis Cartilage2002;10:199–206.CrossrefPubMed Google Scholar
21 Wakitani S , NawataM, TenshoK, et al.Repair of articular cartilage defects in the patello-femoral joint with autologous bone marrow mesenchymal cell transplantation: three case reports involving nine defects in five knees. J Tissue Eng Regen Med2007;1:74–79.CrossrefPubMed Google Scholar
22 Pelttari K , SteckE, RichterW. The use of mesenchymal stem cells for chondrogenesis. Injury2008;39(Suppl 1):S58–S65. Google Scholar
23 Hamid AA , IdrusRB, SaimAB, SathappanS, ChuaKH. Characterization of human adipose-derived stem cells and expression of chondrogenic genes during induction of cartilage differentiation. Clinics (Sao Paulo)2012;67:99–106.CrossrefPubMed Google Scholar
24 Bajada S , MarshallMJ, WrightKT, RichardsonJB, JohnsonWE. Decreased osteogenesis, increased cell senescence and elevated Dickkopf-1 secretion in human fracture non union stromal cells. Bone2009;45:726–735.CrossrefPubMed Google Scholar
25 Undale AH , WestendorfJJ, YaszemskiMJ, KhoslaS. Mesenchymal stem cells for bone repair and metabolic bone diseases. Mayo Clin Proc2009;84:893–902.CrossrefPubMed Google Scholar
26 Krebsbach PH , GuK, FranceschiRT, RutherfordRB. Gene therapy-directed osteogenesis: BMP-7-transduced human fibroblasts form bone in vivo. Hum Gene Ther2000;11:1201–1210.CrossrefPubMed Google Scholar
27 Quarto R , MastrogiacomoM, CanceddaR, et al.Repair of large bone defects with the use of autologous bone marrow stromal cells. N Engl J Med2001;344:385–386.CrossrefPubMed Google Scholar
28 Marcacci M , KonE, MoukhachevV, et al.Stem cells associated with macroporous bioceramics for long bone repair: 6- to 7-year outcome of a pilot clinical study. Tissue Eng2007;13:947–955.CrossrefPubMed Google Scholar
29 Hernigou P , PoignardA, BeaujeanF, RouardH. Percutaneous autologous bone-marrow grafting for nonunions: influence of the number and concentration of progenitor cells. J Bone Joint Surg [Am]2005;87-A:1430–1437. Google Scholar
30 Horwitz EM , GordonPL, KooWK, et al.Isolated allogeneic bone marrow-derived mesenchymal cells engraft and stimulate growth in children with osteogenesis imperfecta: implications for cell therapy of bone. Proc Natl Acad Sci U S A2002;99:8932–8937.CrossrefPubMed Google Scholar
31 Choi JJ , YooSA, ParkSJ, et al.Mesenchymal stem cells overexpressing interleukin-10 attenuate collagen-induced arthritis in mice. Clin Exp Immunol2008;153:269–276.CrossrefPubMed Google Scholar
32 Zheng ZH , LiXY, DingJ, JiaJF, ZhuP. Allogeneic mesenchymal stem cell and mesenchymal stem cell-differentiated chondrocyte suppress the responses of type II collagen-reactive T cells in rheumatoid arthritis. Rheumatology (Oxford)2008;47:22–30.CrossrefPubMed Google Scholar
33 Uccelli A , PistoiaV, MorettaL. Mesenchymal stem cells: a new strategy for immunosuppression?Trends Immunol2007;28:219–226.CrossrefPubMed Google Scholar
34 Wakitani S , OkabeT, HoribeS, et al.Safety of autologous bone marrow-derived mesenchymal stem cell transplantation for cartilage repair in 41 patients with 45 joints followed for up to 11 years and 5 months. J Tissue Eng Regen Med2011;5:146–150.CrossrefPubMed Google Scholar
35 Centeno CJ , SchultzJR, CheeverM, et al.Safety and complications reporting on the re-implantation of culture-expanded mesenchymal stem cells using autologous platelet lysate technique. Curr Stem Cell Res Ther2010;5:81–93.CrossrefPubMed Google Scholar